01 Lect 1 INTRO PHYSIOL 1 MBS 2024 FINAL (1).pdf
Document Details
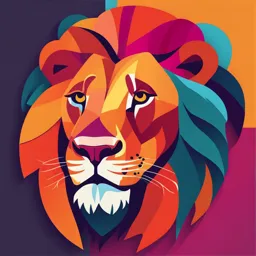
Uploaded by JovialManticore
2024
Tags
Full Transcript
Skeletal Muscle Introduction to Physiology 1 timing should be reasonablbly good Prof. M.A.W. Andrews, Ph.D., FNAOME Prof. M. Andrews © 2023 Skeletal Muscle Introduction to Physiology 1 L...
Skeletal Muscle Introduction to Physiology 1 timing should be reasonablbly good Prof. M.A.W. Andrews, Ph.D., FNAOME Prof. M. Andrews © 2023 Skeletal Muscle Introduction to Physiology 1 LEARNING OBJECTIVES 1. Understand how physiology fits in with medicine. 2. Understand the Tenets of Osteopathic Medicine, and how these general concepts apply to our understanding of physiology. 3. Understand the bases of physiologic differences among humans. 4. Understand the general concepts of dynamic equilibrium (Bernard) and homeostasis (Cannon). 5. Understand the general distribution of the multicellular constitution of the human body, including numbers of cells and total mass of cell types. 6. List some characteristics of life, and describe the levels of organization of the human organism. 7. Define tissue, and list and describe the four primary tissue types, and some of the various types of cells in those tissue. Prof. M. Andrews © 2023 Skeletal Muscle Physiology = Function Physiology is the scientific study of the normal functioning of a living organism and its component parts. More specifically, it is the study of mechanisms. It is the basis of our understanding of the body, and leads us to understand pathology, and then we can move on to treatment, (the 3 Ps, or 2Ps+T) whether that is pharmacological, surgical, osteopathic (OMT), or other, to restore the normal. While, in many cases, we speak and learn within systems of physiology, biochemistry, neuroscience, pathology, pharmacology, etc., it is essential to keep in mind that we must assess the entire organism, the whole, and the interrelatedness within that organism (not an easy task). We can refer to this as a holistic view. Holism is also a major tenet of Osteopathy. In studying osteopathic medicine, we try our best to attain a view of the whole organism, i.e., we strive for holism... “the tendency of nature to form wholes—or organisms, and systems—from the ordered grouping of single units.” General Jan Christian Smuts (1926)* South African statesman, politician, and philosopher This echoes the Aristotelian notion that, “the whole is greater than the sum of its parts.” *In proposing his “Theory of the Whole,” Smuts coined holism from the Greek hólos, meaning “whole,” the reason behind that W-less spelling. Going a step further, the wh- was added in the 15th c. No one fully knows why… Prof. M. Andrews © 2023 Skeletal Muscle Tenets of Osteopathic Medicine The Tenets of Osteopathic Medicine express the underlying holistic philosophy of osteopathic medicine (approved by the AOA, as policy): 1. The body is a unit; the person is a unit of body, mind, and spirit. 2. The body is capable of self-regulation, self-healing, and health maintenance. 3. Structure and function are reciprocally interrelated. 4. Rational treatment is based upon an understanding of the basic principles of body unity, self-regulation, and the interrelationship of structure and function. The philosophy of holism has sparked many debates and a still ongoing shift in focus, from effect to cause, from “signs and symptoms” to “whole individual,” from disease-centered to person-centered care. A holistic treatment might encompass Western medication and procedures, complementary therapy such as massage, and education on lifestyle changes for the patient. Essentially, the focus is on the patient’s overall wellness and not just on disease, keeping in mind the body, mind, spirit connection. 4 Prof. M. Andrews © 2023 Skeletal Muscle Physiology Physiology, a sub-discipline of biology, focuses on how organisms, carry out the chemical and physical functions in a living system, and lead us to what medicine is about, the restoration of the normal function. In the case of osteopathic medical physiology, we are also interested in understanding the holism of human function, so as to eventually treat. The typical parts of medicine are: ↳ PHYSIOLOGY ➔ PATHOLOGY ➔ TREATMENT ↰ Physiology is always complementary to anatomy: structure and function integrate into the totality or whole of the human experience…again holism. Anatomical structures often seem “designed” to perform specific functions because of their unique size, shape, form, or body location. Physiology flows from this design. Which is the driver of this integration? While no two humans are exactly alike anatomically, likewise, while not as easily seen, and less acknowledged, physiological differences exist related to: 1. sex, 2. age, 3. diet, 4. lean body / fat mass, 5. physical activity… 5 Prof. M. Andrews © 2023 Skeletal Muscle Physiology The study of human physiology as a medical field originated in classical Greece, at the time of Hippocrates (late 5th century BC). Outside of the Western tradition, early thoughts of physiology and anatomy can be dated as having originated around the same time in China, India, and elsewhere. Aristotle (384 to 322 BC) speculated about body function with an emphasis on the relationship between structure and function, marking the beginning of true physiology in Ancient Greece. The Greek physician Galen (c. 130–200 AD), a practical student of the function of the body, rose to physician of Roman Emporer Marcus Aurelius, and was one of the first to closely study body function and treat in a practical, not a mystical manner. Galen recognized three systems: the brain and nerves responsible for thoughts and sensations; the heart and arteries which give life; and the liver and veins which he attributed nutrition and growth. For 1,400 years Galenic physiology influenced medicine. At this point, these are primarily thinkers, not doers, and their realm is said to be that of natural philosophy. Galen stated that a physician must be a healer and a philosopher. 6 Prof. M. Andrews © 2023 Skeletal Muscle Physiology Jean Fernel (1497 to 1558) was a French physician who introduced the term “physiology” to describe the study of the function of the body. His treatise, Universa Medicina, was comprised of three parts: the Physiologia, the Pathologia, and the Therapeutice. Starting the marriage of the three concepts into modern medicine. – Physiology is a combination of two Greek terms: physis, meaning “nature,” and logos, meaning “study of”. Many contributed to the development of concepts within the medical and physiological realm, including Ibn al-Nafis, Miguel Serveto, and William Harvey. These and others have been responsible for demonstrating and described many physiological and anatomical concepts through the years. 7 Prof. M. Andrews © 2023 Skeletal Muscle Claude Bernard (1813-78): Modern Physiology Along with his mentor Magendie, started experimental (modern) physiology. He also came to proposed that we have a state of internal balance, achieved via multiple internal control mechanisms which oppose the external forces of change. He went on to discuss the ability of the body to maintain a constant milieu intérieur, as an example of a dynamic equilibrium. At times our systems leave this state more than momentarily and we experience problems, aka, pathologies. 8 Prof. M. Andrews © 2023 Skeletal Muscle Walter Cannon (1871-1945) Cannon (professor and chairman of Physiology at Harvard Medical School) took the conceptual framework of Bernard, and others, the ideas of internal balance, and dynamic equilibrium, and in 1932 proposed the conceptual framework of homeostasis, which is now well accepted in physiology and medicine. The constancy of homeostasis is the main purpose of our physiological mechanisms and is accomplished most often by negative feedback loops. Long-term deviation from homeostasis, and / or loss of feedback, indicates disease. Walter Bradford Cannon The physiological processes which maintain the steady states are so complex that I have suggested a special designation for these states, homeostasis... a condition which may vary, but which is relatively constant. 9 Prof. M. Andrews © 2023 Skeletal Muscle Human Physiology unicellular → multicellular How many cells are there in a human body? Multicellularity allows: – Growth – Differentiation – Complexity 10 Prof. M. Andrews © 2023 Skeletal Muscle How many cells are there in a human body? Approximately 30 TRILLION cells! 30,000,000,000,000 (3.0 x1013) 11 Prof. M. Andrews © 2023 Skeletal Muscle Distribution of Cells in the Human Body Six cell types comprise 96% of the human cell count, and average 100 μm3 /cell : red blood cells (84%; 90 μm3 /cell) platelets (5%) bone marrow cells (2.5%) endothelial cells (2%) lymphocytes (1.5%) hepatocytes (1%) The remaining 50 cell types account for 4% of the total cells. By weight, as the reference man has a mass of 70 kg: 25% is extracellular fluid; 7% is extracellular solids. Leaving 46 kg of cell mass, of which 75% is adipocytes and muscle cells; adipocytes and myocytes are 0.2% by number. Eva Bianconi, Allison Piovesan, Federica Facchin, Alina Beraudi, Raffaella Casadei, (0.2 kg) Flavia Frabetti, Lorenza Vitale, Maria Chiara Pelleri, Simone Tassani, Francesco Piva, Soledad Perez-Amodio, Pierluigi Strippoli & Silvia Canaider (2013) An estimation of the number of cells in the human body, Annals of Human Biology, 40:6, 463-471. Sender R, Fuchs S, Milo R. (2016) Revised Estimates for the Number of Human and Bacteria Cells in the Body. PLoS Biol. 2016 Aug 19;14(8). Prof. M. Andrews © 2023 12 Skeletal Muscle Characteristics and Organization of Life 1. Organization 2. Cellular composition (at least one) 3. Metabolism anabolism, catabolism and excretion 4. Responsiveness and movement stimuli 5. Homeostasis 6. Development differentiation and growth 7. Reproduction including cellular replacement; species continuation 8. Evolution mutations Courtesy Barbara Cousins Prof. M. Andrews © 2023 Skeletal Muscle Organs are composed of multiple tissue Before we start to speak of organs, organ systems, and the entire human organism, it is good to know and to understand the tissue types. – Tissues are important to understand as they are the next rung up on the ladder from cells. – Tissue are a group of (two or more) similar cells which come together to form a working unit. – Organs are, as we proceed to the next higher level, composed of two or more tissue, functioning together. – As you can see, knowing of cells helps you understand tissues, and knowledge of tissues will, in turn, help you understand organs and their physiology. Four primary tissues differ from one another in the: – types and functions of their cells; – the characteristics of the matrix (extracellular material); – the relative amount of space occupied by cells versus matrix. Extracellular matrix (ECM) is biochemically complex, but nonliving, material between and among cells. This extracellular material is composed of: – fibrous proteins such as collagen, elastin; – a clear gel known as ground substance; and – extracellular fluid (ECF). – N.B., The four tissue types vary regarding the amount of ECM. 14 Prof. M. Andrews © 2023 Skeletal Muscle Organs are composed of multiple tissue The four tissue types are: 1. Epithelial tissue, also referred to as epithelium (covering) and endothelium (lining), refers to the sheets of cells that cover exterior surfaces of the body, line internal cavities and passageways, and form glands (glandular epithelium). 2. Connective tissue, as its name implies, binds the cells and organs of the body together and functions in the protection, support, and integration of all parts of the body. 3. Muscle tissue is excitable, responding to stimulation and contracts to provide force generation and possibly movement (if F > R), and occurs as three types: skeletal (voluntary) muscle, cardiac muscle, and smooth muscle. 4. Nervous tissue is also excitable, allowing the propagation of electrochemical signals in the form of nerve impulses that communicate between different regions of the body. Nervous tissue is composed of neurons and supporting cells called neuroglia or glial cells (there are six types of neuroglia, four in the central nervous system and two in the PNS). 15 Prof. M. Andrews © 2023 Skeletal Muscle Epithelial Tissue (Epi- and Endothelium) consists of a flat sheet of closely Dense irregular adhering cells Dead squamous cells Living epithelial cells connective tissue one or more cells thick upper / apical surface usually exposed Areolar tissue to the environment or an internal space in the body; basal surface attached to other cells covers body surface lines body cavities forms the external and internal linings of many organs constitutes exocrine and endocrine glands extracellular material is so thin it is not visible with a light microscope Example: keratinized stratified epithelium (skin): epithelia allows no room for blood vessels multiple cell layers with cells becoming flat and scaly toward surface basal surface lies on a layer of loose epidermis; palms and soles heavily connective tissue and depend on its keratinized blood vessels for nourishment and resists abrasion; retards water loss through waste removal skin; resists penetration by pathogenic organisms 16 Prof. M. Andrews © 2023 Skeletal Muscle Connective Tissue most abundant, widely distributed, and Collagen fibers Ground substance Fibroblast nuclei histologically variable of the primary tissues cells usually occupy less space than the extracellular material and most cells of connective tissue are not in direct contact with each other, separated by extracellular material generally highly vascular binds organs to each other: tendons and ligaments supports and protects organs: bones (cranium, ribs, sternum) and cartilage movement: bones provide lever system for skeletal muscle densely, packed, parallel collagen fibers, with immune protection: leukocytes compressed fibroblast nuclei storage: adipose (fat), bones (calcium, tendons attach muscles to bones and phosphorus) ligaments hold bones together heat production: adipocytes transport: the blood 17 Prof. M. Andrews © 2023 Skeletal Muscle Nervous Tissue nervous tissue – specialized for Nuclei of glial cells Axon Neurosoma Dendrites communication by electrical and chemical signals consists of neurons (excitable cells) – detect stimuli – respond quickly – transmit coded information rapidly to other cells and neuroglia (glial) – protect and assist neurons – ‘housekeepers’ of nervous system neuron parts – neurosoma (cell body) houses nucleus and other organelles cell’s center of genetic control and protein synthesis – dendrites multiple short, branched processes receive signals from other cells transmit messages to neurosoma – axon (one per cell, efferent activity) sends outgoing signals to other cells can be more than a meter long 18 Prof. M. Andrews © 2023 Skeletal Muscle Neuroglial Cells Capillary Neurons Astrocyte Oligodendrocyte Perivascular feet Myelinated axon Myelin (cut) Ependymal cell Microglia neuroglia (glial cells) outnumber neurons by about 50:1 – in fetus, they guide migrating neurons to their destination; – bind neurons together and form a framework for nervous tissue prevents neurons from inappropriate contact with one another gives precision to conduction pathways – support and protect the neurons throughout life 19 Prof. M. Andrews © 2023 Skeletal Muscle Astrocytes Astrocytes Astrocytes are stellate and the most abundant glia in the CNS. Their processes cling to and cover neurons and capillaries, anchoring neurons to their nutrient supply lines. They are responsible for interacting metabolically with neurons uptake of K+ from the ECF to maintain ionic environment for neurons; Astrocytes form the Blood-Brain Barrier processes uptake glucose from brain capillaries, can store and then convert (BBB), which is formed by the processes of astrocytes; the glucose to lactate so that neurons can more readily use the energy to limits the passage of materials between produce ATP; blood and brain; uptake and recycle neurotransmitter CNS capillaries cells are joined by tight- released from axon terminals; tight jcts between cells; substances can thus move only by very help guide the migration of young neurons in the developing CNS for selective processes transcellular, not at synapses. all paracellular (between capillary cells; Release molecules (gliotransmitters) one problem this presents is that that can stimulate or inhibit neurons: chemotherapeutic agents have difficulty glutamate, ATP, adenosine, D-serine. penetrating the BBB. 20 Prof. M. Andrews © 2023 Skeletal Muscle Oligodendrocytes Oligodendrocytes Oligodendrocytes of the CNS associate with a number of nearby axons and produce an insulating cover called myelin, which they share with and help wrap these multiple axons. A single oligodendrocyte can extend its processes to 50 axons, wrapping approximately 1 μm of myelin sheath around each axon (neurolemocytes differ greatly here); These are the “white matter” of the CNS. The myelination is responsible for increased conduction velocity and reducing the energy needed to conduct an impulse. 21 Prof. M. Andrews © 2023 Skeletal Muscle Neurolemmocytes Neurolemmocytes (Schwann Cells) Neurolemmocytes are the primary glia of the peripheral nervous system, surrounding all axons in the PNS to form a neurilemmal sheath, and wrapping axons with myelin sheaths where appropriate. So, their function is similar to oligodendrocytes of the CNS, however, they differ in that one neurolemmocytes is associated with a single axon, each cell myelinating about 1 mm length of axon, with many associated with each axon. Myelin sheath are produced in the PNS by wrapping multiple times in a process called myelination. Myelination is essential as it aides in conducting neural impulses in a more rapid manner than if the axons are non-myelinated (demyelination, aka, loss of myelination which at some time existed, is a disease process). The gaps of myelin, which exist between adjacent myelinated sections, are called the nodes of Ranvier. 22 Prof. M. Andrews © 2023 Skeletal Muscle Neuroglial Cells Microglial Cells Microglial cells are small and ovoid in shape with thorny processes. They are responsible for phagocytosis of pathogens and cellular debris in the CNS when invading microorganism or dead neurons are present. Ependymal Cells Ependymal cells are ciliated and line (like epithelial cells elsewhere; these are neural tissue) the central cavities (ventricles) of the brain and central canal of the spinal cord where they form a relatively permeable barrier between the CSF that fills these cavities and the tissue cells of the CNS. Ependymal cells also cover tufts of capillaries to form the choroid plexus of lateral ventricles that produce cerebrospinal fluid. Satellite Cells Satellite cells surround neuron cell bodies in the peripheral nervous system (PNS), working analogous to the astrocytes of the CNS, particularly notable of the support functions within sensory and autonomic ganglia. 23 Prof. M. Andrews © 2023 Skeletal Muscle Muscular Tissue Elongated cells that are specialized to generate force in response to stimulation primary job is to exert physical force creates movements for skeletal structure, digestion, waste elimination, breathing, and blood circulation important source of body heat (all types) three types of muscle: skeletal, cardiac, and smooth, each having specific characteristics and functions (magnifications of these photomicrographs are not the same). multiple nuclei adjacent to only found in heart short, fusiform cells which lack visible striations membrane striations like skeletal striations – alternating dark single (some bi-) nucleated, involuntary, ANS input and light protein bands centrally located with referred to as visceral muscle, it forms layers of digestive, voluntary – conscious glycogen respiratory, urinary tracts, blood control via 𝝰-MN involuntary and vessels, uterus and other viscera most attach to bone with autorhythmic, can be propels contents through the some exceptions. modulated by ANS lumen of an organ, regulates intercalated discs join diameter of blood vessels cardiocytes toprovide electrical and mechanical Prof. M. Andrews © 2023 connections Skeletal Muscle Feedback Control and the Extracellular Environment Prof. M.A.W. Andrews, Ph.D., FNAOME Prof. M. Andrews © 2023 Skeletal Muscle Introduction to Physiology 2 LEARNING OBJECTIVES 1. Understand the principles and functions of positive and negative feedback in physiological systems, and be able to offer examples of both. 2. Define and differentiate between intrinsic and extrinsic regulation of body processes. 3. Define and differentiate among ECF, ICF, interstitial fluid and plasma, and the general volumes of these in the human body. 4. Know advantages and disadvantages to multicellularity. Understand the importance of the circulatory system in a multicellular organism, and the importance of capillaries in transport. Prof. M. Andrews © 2023 Skeletal Muscle Control Systems: Regulation Negative feedback – This is the basis of all regulation in the body, and is responsible for the maintenance of homeostasis, and maintaining physiological paraments within the normal range. – It is important to realize that a single negative feedback system never works in isolation. – The products of a process act at an earlier stage in the process to inhibit their own formation. – This tends to stabilize the process. Positive feedback – The products of a process act at an earlier stage in the process to enhance their own formation or trigger a further step. – This tends to amplify the process. 27 Prof. M. Andrews © 2023 Skeletal Muscle Negative Feedback Loop Room temperature and an HVAC system is a common example. – It does not stay at set point of 68 degrees – it only averages 68 degrees – Body senses a change and activates mechanisms to reverse it – Maintain a dynamic equilibrium 1 Room temperature falls to 66°F (Room cools (19°C) C 10° 15° 20° 25° 6 1) Sense and 2) compare down) F 50° 60° 70° 80° the condition to a set-point. 2 4) The final effect C 10° 15° 20° 25° inhibits the system Thermostat activates the F 50° 60° 70° 80° that was activated furnace 5 Thermostat shuts off furnace 4 Room temperature rises to 70°F (21°C) 3 Heat output; aka, the product 3) An effector Prof. M. Andrews © 2023 generates an output Skeletal Muscle Negative Feedback Loops Pathway – Sensors in the body to detect change and send information to the – Integrating centers, which assesses change around a set point, sending instructions to an – Effector, which can make the appropriate adjustments to counter change from set- point Mechanism of Negative Feedback Loops – Move in the opposite direction from the change; – Minimizing the change from the set-point; – This is a continuous process, with fine adjustments constantly being made 29 Prof. M. Andrews © 2023 Skeletal Muscle Negative Feedback Loop: Temperature Maintenance of body temperature is an example of negative feedback mechanisms at work – Sensors in the hypothalamus (an important autonomic N.S. center) of the brain detect deviations from the set point (37°C), assesses this as actionable, and effectors (sweat glands or shivering) are stimulated to cool or heat the body. – Once the body is back to its normal set point, sensors alert the integrating center and the initial effectors are inhibited, shutting off or “down-regulating” the process. This is why it is called a negative feedback loop. – Antagonistic Effectors : this is a major concept involved in homeostasis as constancy is often maintained by opposing effectors that move conditions in opposite directions. This maintains conditions within a normal range, or dynamic equilibrium, aka homeostasis. Example: when you are hot, you sweat; when you are cold, you shiver. Other examples – blood glucose levels, blood calcium levels, heart rate, and blood pH. 30 Prof. M. Andrews © 2023 Skeletal Muscle Negative Feedback Loop: BP Failure of this feedback loop may produce dizziness in dehydrated, ill or elderly. 31 Prof. M. Andrews © 2023 Skeletal Muscle Positive Feedback: Amplification The products of a process act at an earlier stage in the process to enhance their own formation or trigger a further step. It is self-amplifying cycle and leads to greater change in the same direction. Ends when a major outcome is reached. This is far less common than negative feedback mechanisms, however it is an excellent means by which rapid changes are produced. Positive feedback is the basis for action potentials, blood clotting, protein digestion, and fever. A common example is the neuroendocrine cycle of childbirth. 3. Brain stimulates 3 pituitary gland to secrete oxytocin. 5. Eventually the major 4 outcome (product) is 2 reached, and no longer is process #1 active. 2. Due to stretch, nerve impulses 4. Oxytocin stimulates from the cervix uterine contractions and are transmitted 1 pushes fetus toward cervix to brain. 1. Head of fetus pushes against cervix. Prof. M. Andrews © 2023 Skeletal Muscle Intrinsic and Extrinsic Regulation Regulation of processes can occur in two ways: a. Intrinsically: cells within the organ sense a change and signal to neighboring cells to respond appropriately. b. Extrinsically: the brain (or other organs) regulates an organ using the endocrine or nervous system. i. The three major parts of the nervous system: sensory, integrative (central nervous system), motor; work via electrochemical messaging. ii. The endocrine system works via hormone release: eight major endocrine glands secrete hormones into the extracellular fluid (the milieu intérieur; ECF). 33 Prof. M. Andrews © 2023 Skeletal Muscle Fluid Compartments in the Body Total Body Water (TBW) – 60+% body weight, variable due to lipid content. Essential differences between ECF and ICF include solutes and ions, some are maintained by transport mechanisms in the cell membranes (e.g., Na-K ATPase, Ca2+ ATPase). Intracellular fluid (ICF) - potassium, magnesium, phosphate, non-diffusible intracellular proteins. Extracellular fluid (ECF) - sodium, chloride and bicarbonate ions, non-diffusible plasma proteins. 34 Prof. M. Andrews © 2023 Skeletal Muscle Human Physiology unicellular → multicellular Advantages: Disadvantages: – Growth – Separated from the nutritive – Differentiation environment – ↑ Energy / waste – Complexity – Affected by pathogens – Longer to maturation 35 Prof. M. Andrews © 2023 Skeletal Muscle ECF and Transport ▪ Throughout the body transport is assured by a circulatory system. ▪ Around the cells – interstitial fluid and plasma exchange assure a constant equilibration of nutrients and waste (cells must be, at most, 50 μm from a capillary) capillary bed with fluid exchange 36 Prof. M. Andrews © 2023 Skeletal Muscle Nutrients and Wastes Respiratory system – blood always passes through lungs – acquires O2 by diffusion – respiratory membrane 0.4-2.0 μm Gastrointestinal Tract – significant blood flow through GI – acquires dissolved nutrients (carbohydrates, fatty acids, amino acids, etc) by absorption into ECF Liver and other metabolically active organs (fat, mucosa, kidneys, endocrine) – modifies nutrients to useable forms and stores them for specific end target tissues Musculoskeletal system – gets us out of harms way, allows us to move to acquire substrates Carbon dioxide – lungs – concurrent functionality – exchange CO2 for O2 Other metabolites – kidney – many cellular metabolites are washed into the ECF – plasma – grossly filtered at the kidney – selective reabsorption within the kidney – excretion of waste/excess Other metabolites – GI – some substances are not needed (primary form or metabolite) and never get absorbed and pass through 37 Prof. M. Andrews © 2023 Skeletal Muscle Vital Quantitative Measurements of ECF Approximate Normal Ranges for Measurements of Some Fasting Serum Blood Values (just a sampling) Quantitative Measurements – In order to study Measurement Normal Range physiological / clinical mechanisms, scientists Arterial pH 7.35 to 7.45 must measure specific values and determine Bicarbonate 22 to 28 mEq/L statistics as averages and Sodium 136 to 142 mEq/L normal ranges. – A knowledge of normal Calcium (total) 8.2 to 10.2 mg/dL ranges aids in Oxygen content (not PO2) 18 to 20 ml/dL diagnosing diseases and in assessing the Urea 8 to 23 mg/dL effects of drugs and other treatments in Creatinine 0.6-1.2 mg/dL experiments. Here are some typical solutes Albumin (plasma protein) 3.5 to 5.0 g/dL and their normal ranges. Osmolality 275–295 mOsm/kg Glucose 70 to 110 mg/dL 38 Prof. M. Andrews © 2023 Skeletal Muscle Membrane Physiology 1 Prof. M.A.W. Andrews, Ph.D., FNAOME Prof. M. Andrews © 2023 Skeletal Muscle Cell Membranes 1 LEARNING OBJECTIVES 1. Describe the general composition of a cell membrane and fully describe the six major functions of cellular membrane proteins, giving examples. 2. Describe the concept of signaling pathways and second messengers, why they are useful and their role in determining cell function. 3. Discuss why membranes “pose a formidable barrier” to most biologically active compounds and be able to explain the statement that, “cell membranes are both a barrier and gateway between the cytoplasm and the ECF.” 4. Know that gradients exist across the cell membrane, how they may be generated, and list the typical value and normal range for the gradients for sodium, potassium, chloride, calcium, bicarbonate and glucose. 5. Contrast the following units used to describe concentration: mM, mEq/l, mg/dl, mg%. 6. Understand Fick’s Laws of diffusion as they relate to a biological system, and how changes in the concentration gradient, surface area, and permeability will influence the diffusional movement of a compound, i.e., flux (J). 7. List and fully describe diffusional and active transport mechanisms which affect transport across cell membranes. 8. With relation to facilitated (mediated) transport, briefly discuss specificity, and saturation kinetics and the concept of Tm. 9. Define and describe channels and their selectivity. Prof. M. Andrews © 2023 Skeletal Muscle Cell Membrane Integral proteins are those intricately associated with the lipid bilayer, with some spanning the bilayer and known as transmembrane proteins. Others are neither imbedded nor covalently associated with the membrane and referred to as peripheral proteins. The plasma membrane is both a barrier and a gateway between the cytoplasm and ECF. – it is a selectively permeable barrier which allows some particles to cross, preventing others. The plasma membrane by molecule number is only 2% protein, however, proteins account for 50% of a cell membrane’s mass. 41 Prof. M. Andrews © 2023 Skeletal Muscle Membrane Protein Functions Cell-adhesion Membrane Channels allow ions Cell-identity Anchor proteins Receptors bind molecules (CAM) bound enzymes and water to pass markers, can physically link chemical and intracellular generate or into and out of the glycoprotein intracellular messengers such communication hydrolyze a cell, and may be acting as a cell- structures of the as hormones or proteins chemical reaction either constantly identity marker cytoskeleton with neurotransmitters are proteins which or generate a open (leakage pores) helps extracellular released by other adhere one cell to messenger. or their opening is distinguishing structures. cells. another and regulated (gated), so the body’s own They are usually possibly allow they open and cells from foreign specific for one communication close in response to cells and substrate, and an with the certain stimuli. pathogens. Part of intracellular extracellular the MHC / HLA response is environment. Carriers / complex. generated (2’ Many cells to not transporters attach Glycoproteins messengers). survive unless a solute, release it on contribute to the Those coupled to they are anchored the other side. May glycocalyx G proteins are the in some manner require ATP, or use carbohydrate most common to the ECM or the electrochemical surface coating, (>1000). other cells. gradients across the and acts as a membrane. cell’s identification. Prof. M. Andrews © 2023 Skeletal Muscle G-Protein Coupled Receptor (GPCR) Systems 43 Prof. M. Andrews © 2023 Skeletal Muscle GPCR Example: Adrenergic Receptor Activation 44 Prof. M. Andrews © 2023 Skeletal Muscle GPCR System: Amplification N.B. The amino acids most commonly phosphorylated are serine, threonine, and tyrosine. 45 Prof. M. Andrews © 2023 Skeletal Muscle Some Gradients Across the Cell Membrane 46 Prof. M. Andrews © 2023 Skeletal Muscle Physiol. Application of Fick’s Laws of Diffusion Diffusion flux (J) = -P (c2-c1) * In addition: A form of ↑ temperature Fick’s Equation: → ↑ molecular motion and ↑ diffusion. * P = permeability, i.e., ‘conductance’ c2-c1 = difference in concentration for the direction of flow from c1 to c2 Prof. M. Andrews © 2023 Skeletal Muscle Transport across the cell membrane Diffusion (simple vs. facilitated) Active transport (primary vs. 2’) Occurs down a concentration Occurs against a concentration gradient; gradient There will be net movement of Involves a protein “carrier” molecules until concentration is ATP hydrolysis required at some equal everywhere; flux will point, so an ATPase enzyme is involved; continue, but concentrations stay. There are two major types of active Occurs through lipid bilayer or transporters: primary (ATP directly used) involves a protein “channel” or and secondary (ATP used “indirectly”, in a carrier” related process, e.g., Na-K ATPase). No chemical energy input required. Prof. M. Andrews © 2023 (primary) Skeletal Muscle Transporters and Channels Transport proteins are not pores, and each type specifically binds to glucose, electrolytes, or another solute, allows them to cross the membrane, which they would otherwise be unable to do, either due to charge and /or size. – if the carrier allows ligands to move down their gradients via thermal motion and flux, the transporters are referred to as facilitators, i.e., helpers – or if the transport involves moving a ligand against its gradient, this is an an active process, and active means ATP must used. The transporter can is an enzyme and can be referred to as an ATPase or “pump” protein. Channels are transmembrane proteins that act as facilitators, with selective pores that specifically allow water (aquaporins) or dissolved ions to pass through (across) the membrane. Such charged particles cannot penetrate the phospholipid membranes. – some are constantly open (called leakage pores) – some are controlled by ”gates” in the protein structure, which occlude the pore; each such gated-channel opens and closes in response to specific stimuli and are either ligand (chemically)-regulated gates; voltage-regulated gates; mechanically regulated gates (stretch and pressure). – regulated channels play an important role in most cellular activation 49 Prof. M. Andrews © 2023 Skeletal Muscle Facilitated Diffusion Facilitated diffusion involves carrier-mediated transport of solute through a membrane down its concentration gradient. It does not consume ATP, uses concentration gradient and the thermal energy inherent in the system. The solute attaches to binding site on the carrier, the carrier changes confirmation, then releases the solute across the membrane. This can occur in either direction and depends on the concentration gradient. ECF ICF 1 A solute particle enters 2 The solute binds to a receptor 3 The carrier releases the the channel of a membrane protein (carrier). 3-50 site on the carrier and the carrier changes conformation. solute on the other side of the membrane. 50 Prof. M. Andrews © 2023 Skeletal Muscle Facilitated Diffusion Transporter shows Specificity: Rate of facilitated diffusion is specific for a certain ligand limited by Vmax of a carrier protein solute binds to a specific receptor site carriers do not change their ligand − Units are pmol/min/mg protein picks them up on one side of the membrane, and release them, unchanged, on the other. Saturation: as the solute concentration rises, the rate of a transporter rises, but every carrier has a Transport Maximum (Tm). Once Tm is reached, more transporter molecules are needed to increase rate. Transport maximum (Tm) 51 Prof. M. Andrews © 2023 Skeletal Muscle Channels As noted earlier, channels allow ions and This diagram shows gating of a sodium channel water to pass into and out of a cell, and may be either constantly open (leakage pores) or their opening is regulated (gated) so they open/ close only at certain times, due to specific stimuli, including chemical (ligand) binding (hormones, neurotransmitters), membrane voltage changes (local and action potentials, TBD), or mechanical perturbation (touch, pressure, muscle length). Unhydrated Hydrated Channels are, like transporters, highly Sodium 0.95 Å 3.58 Å specific, and have a Tm. In the case of Potassium 1.33 Å 3.31 Å sodium channels, they are lined with negatively charged amino acids that literally pull the sodium ion away from its water shell. The smaller unhydrated sodium ion can then diffuse through the channel, while the unhydrated potassium ions are larger and cannot pass. 52 Prof. M. Andrews © 2023 Skeletal Muscle Selectivity of Potassium Channel Then what of the potassium channel? If sodium is smaller, why doesn’t it enter. The answer again, has to do with the hydration shells of the two ions. Carbonyl oxygens in the selectivity channel essentially strip water molecules from the potassium molecule (but cannot do the same with sodium ions). So, only potassium ions can permeate. Dehydrated Hydrated Sodium 0.95 Å 3.58 Å Potassium 1.33 Å 3.31 Å 53 Prof. M. Andrews © 2023 Skeletal Muscle Membrane Physiology 2 Prof. M.A.W. Andrews, Ph.D., FNAOME Prof. M. Andrews © 2023 Skeletal Muscle Cell Membranes 2 LEARNING OBJECTIVES 1. Define active transport and distinguish between primary and secondary active transport, giving examples. 2. Describe what is meant by the statement that epithelial cells are “polarized,” and explain the functional significance of polarized distribution of various transport proteins to the apical or the basolateral cell membrane. 3. Compare and contrast transcellular and paracellular movement. 4. Understand the four major functions of the Na-K ATPase. 5. Define, differentiate among, and understand the processes of endocytosis, exocytosis, phagocytosis, pinocytosis, receptor-mediated endocytosis, and vesicle fusion, and understand the function of clathrin. 6. Define and understand the process of osmosis and that movement of water is driven by solute movement, and differentiate among the terms osmole, osmolarity, osmolality and tonicity, and list the typical normal range for plasma osmolality. 7. Define and understand the force of osmosis v. hydrostatic force. 8. Describe the role of water channels (aquaporins) in facilitating the movement of water across biological membranes. 9. Define isosmotic, hyperosmotic and hypoosmotic conditions and discuss their effect on cell swelling and shrinking. Prof. M. Andrews © 2023 Skeletal Muscle Active Transport Active transport is a carrier-mediated transport of a solute through a membrane, but differs from facilitation in that it requires energy, either directly, or in a second related process, and moves the solute against a chemical or electrochemical gradient. 56 Prof. M. Andrews © 2023 Skeletal Muscle Primary Active Transport In primary active transport, molecules are moved or “pumped” against an electrochemical gradient at the expense of energy (ATP). A classic example is the Na+-K+ ATPase (pump) Antiporter enzyme located on the plasma membrane of all animal cells. Moves sodium ions out of cells (3) and potassium ions (2) into cells against electrochemical gradients. Plays a critical role in regulating osmotic balance by maintaining Na + and K+ balance Accounts for about 60% of typical cell energy output, more so in neurons. Others: Ca2+ ATPase Present on the cell membrane and the sarcoplasmic reticulum in muscle fibers Maintains a low cytosolic Ca2+ concentration H+ ATPase Found in parietal cells of gastric glands (HCl secretion) and intercalated cells of renal tubules (controls blood pH), able to concentrates H + ions up to 1 million-fold. 57 Prof. M. Andrews © 2023 Skeletal Muscle Secondary Active Transport Systems This process is driven by the energy stored in the electrochemical gradient generated by another molecule (usually sodium) moved by ATP hydrolysis. As such, it is an indirect use of energy. Involves the use of an electrochemical gradient (usually for sodium) Protein cotransporters are classified as symporters or antiporters Symporters: transport substance in same direction as a “driver” ion like Na+. (Glucose, AAs, bicarbonate) Antiporters: transport substance in opposite direction as a “driver” ion like Na+. 58 Prof. M. Andrews © 2023 Skeletal Muscle Polarized State of Transport Epithelium Typically epithelial cells are polarized: apical v. “basolateral.” 59 Prof. M. Andrews © 2023 Skeletal Muscle Sodium-Potassium ATPase Functions Regulates cell volume. – Intracellular “fixed anions” attract sodium, causing osmosis. – The cell swelling stimulates the Na+- K+ ATPase to ↓ ion concentration, ↓ osmolarity and ↓ cell swelling (and damage). Allows many secondary active transport processes. – Such processes do not directly consume ATP. – Instead, the steep sodium concentration gradient that is maintained across the cell membrane (like water behind a dam), allows, e.g., – the sodium-glucose transport protein (SGLT) to simultaneously bind sodium and glucose and carry both into the cell. In so doing, the transporter uses the gradient generated by the Na-K ATPase to transport glucose. This is just one example. Generates heat. – Consume ATP and produce heat as a by-product. – Thyroid hormone increase the number of Na+ - K+ ATPases. Maintains a membrane potential in all cells. – Pump helps keep inside more negative, outside more positive. – It is particularly necessary for nerve and muscle function. 60 Prof. M. Andrews © 2023 Skeletal Muscle Vesicular Transport Vesicular Transport: processes that move large particles, fluid droplets, or numerous molecules at once through the membrane in vesicles, i.e., “bubble- like” enclosures of membrane – High energy consuming processes, using motor proteins and consuming ATP Endocytosis: vesicular processes that bring material into the cell. – phagocytosis: “cell eating” in which large particles are engulfed. – pinocytosis: “cell drinking” in which droplets of ECF, containing molecules useful to the cell, are ingested. – receptor-mediated endocytosis: a more precise, receptor driven endocytosis in which molecules of interest bind to specific receptors on the plasma membrane. These areas are associated with the molecule clathrin, and the cells have clathrin-coated pits, which lead to production of clathrin coated vesicles once engulfed. Exocytosis: the opposite process in which material is discharging from the cell. Involved with release of many substances including neurotransmitters. 61 Prof. M. Andrews © 2023 Skeletal Muscle Phagocytosis or “Cell-Eating” Helps keep tissues free of debris and infectious microorganisms. Particle 1 A phagocytic cell encounters a particle of foreign matter. 7 The indigestible residue is voided by Pseudopod exocytosis. Residue 2 The cell surrounds the particle with its Nucleus pseudopods. Includes neutrophils, monocytes, macrophages, mast cells, and dendritic cells which have cell surface receptors that can detect bacteria, etc., not normally found in the body. Phagosome 6 The phagolysosome fuses with the 3 The particle is phagocytized Lysosome and contained in a plasma membrane. Vesicle fusing phagosome. with membrane Phagolysosome 5 Enzymes from the lysosome digest the 4 The phagosome fuses foreign matter. with a lysosome and becomes a phagolysosome. 62 Prof. M. Andrews © 2023 Skeletal Muscle Receptor Mediated Endocytosis More selective endocytosis. Receptors generated by the cell are associated with the clathrin molecules, and the clathrin enables cells to endocytose these receptors and the associated ligands that bind to these receptors. Clathrin-coated vesicle are then taken into the cytoplasm – an example is the uptake of LDL from bloodstream. Extracellular molecules Receptor Coated pit Clathrin- coated Clathrin vesicle 1 Extracellular molecules bind to 2 Plasma membrane sinks inward, 3 Pit separates from the membrane, receptors on plasma membrane; forms clathrin-coated pit. forms clathrin-coated vesicle receptors cluster together. containing concentrated molecules from ECF. 63 Prof. M. Andrews © 2023 Skeletal Muscle Receptor Mediated Endocytosis 64 Prof. M. Andrews © 2023 64 Skeletal Muscle Exocytosis Secretion of materials, including neurotransmitters. Also useful for replacement of plasma membrane removed by endocytosis. Dimple Fusion pore Secretion Plasma membrane Linking protein Secretory vesicle 1 A secretory vesicle approaches 2 The plasma membrane and the plasma membrane and docks vesicle unite to form a fusion on it by means of linking proteins. pore through which the vesicle The plasma membrane caves in contents are released. at that point to meet the vesicle. 65 Prof. M. Andrews © 2023 Skeletal Muscle Osmosis Osmosis is the (always passive) movement of water down its concentration gradient, from high water concentration toward a lower water concentration (thus into a more solute concentrated / higher particle solution). This movement is typical in a biological system, driven by impermeable particles that are thus “osmotically active,” meaning they cause water to move (if a particle easily crosses a membrane, e.g., urea, it is not osmotically active, as it, and not water, moves) and water attempts to equal the concentration of solvent and solute across a cell membrane. It is opposed by the hydrostatic pressure, which is the build up of water which pushes in the opposite direction. Thus Osmolality describes the number of osmotically active (impermeable) particles in a solution. Osmolality is stated in Osm or more typically mOsm (milliosmolal), with units of mOsm/kg H2O. This is also known as the tonicity of a soolution. The tonicity of the ECF is calculated as 290 mOsm. The difference in mOsm between the ECF and ICF is the osmotic gradient which affects the movement of water. When stated for a specific molecular species, e.g., sodium, glucose, calcium, concentrations are stated in mM (millimolal), also noted as mM/kg water, the concentration of the specific molecule per kg H2O. And, in the case of charged molecules, the parameter of mEq (milliequivalents), or mEq/L, is used and relates to the number of charges of the specific molecule per liter (for monovalent ions mEq = mM; for a divalent such as calcium the mEq = mM × 2,, etc.). 66 Prof. M. Andrews © 2023 Skeletal Muscle Osmotic Pressure The diagram to the right illustrates osmosis in the Start Side A Side B presence of osmotically active particles. It starts with a water permeable membrane between side A and B. Side A differs in that there is a greater mOsm tonicity of this solution. As a result, water flows by osmosis from side B to A. This model can be used to describe the relationship between the intracellular (B) and extracellular (A) fluids. Aquaporins, water leakage channel proteins which allow passage of water, are the main thoroughfares used by water across cell membranes. Cells can alter the rate of osmosis by installing more or removing aquaporins from membranes. 30 minutes later Water, while polar, is small enough that it can pass through the phospholipid layers of the plasma Osmotic membrane, though far less easily. pressure Once water osmoses across the membrane and the Hydrostatic two sides come to a new steady state (30 minutes), pressure the solution on side A then exerts a hydrostatic pressure (due to the presence of the water) that balances osmosis. Heart drives water out of capillaries by reverse osmosis – capillary filtration. Once water comes into contact with the solute particles on side A, there is a reversible attraction of water to the particles. The water forms hydration spheres, making these water molecules less available to diffuse back to the side from which they came. 67 Prof. M. Andrews © 2023 Skeletal Muscle Tonicity Such osmotically active particles, and thus solution tonicity, have an ability to affect fluid volume and hydrostatic pressure in a cell. A solution is hypotonic if it has a tonicity lower than the ICF. As it has less nonpermeating solutes than intracellular fluid (ICF), we can also see that it has a higher water concentration, and the cells absorb water, swell and may burst (lyse). On the other hand, a solution is hypertonic if it has a tonicity higher than the ICF. As it has more nonpermeating solutes than intracellular fluid (ICF), we can also see that it has a lower water concentration, and the cells lose water and shrivel (crenate). An isotonic solution is one in which tonicity of the ECF and ICF are the same (normal IV saline). In this case there is no gain or loss of water from the cell, and no changes in cell volume or cell shape. Below is a view of a red blood cell placed in solutions of varying tonicity. (a) Hypotonic (b) Isotonic (c) Hypertonic 68 Prof. M. Andrews © 2023 Skeletal Muscle Electrical Activities of the Cell Membrane (Membrane Potentials and Action Potentials) MED 510 — Human Physiology I Lectures 5-7 Dr. Hong Zhu [email protected]; 412-396-1070 DUQCOM MBS 2024 - 2025 Prof. M. Andrews © 2023 Skeletal Muscle Learning Objectives State the distribution of major ions (Na+, K+, Ca2+, and Cl-) in intracellular and extracellular fluids, and the two conditions for ion diffusion across cell membrane. Explain diffusion potential and equilibrium potential. Use Nernst Equation to calculate the equilibrium potential of an ion. Describe K+ efflux as the primary determinant of resting membrane potential. Explain how K+ membrane permeability or concentration changes affect the membrane potential. Define the following terms related to membrane potentials: polarization, depolarization and hyperpolarization. Define threshold potential. Describe the properties of action potential and explain the “all- or-none” concept. Compare action potentials with local (graded) potentials. Diagram the phases of an action potential in neurons and skeletal muscle cells, and the membrane potential changes in each phase. Prof. M. Andrews © 2023 Skeletal Muscle Learning Objectives-Cont’d Explain the ionic bases underlying each phase of an action potential. Explain the three states of voltage-gated Na+ channels: closed and active state, open state, closed and inactive state. Explain the ability of a cell to fire repeated action potentials based on the absolute and relative refractory periods of the action potential. Describe the propagation of action potential and the factors affecting its conduction velocity along the nerve fibers. Explain saltatory conduction of an action potential in myelinated nerves and how demyelination affects action potential conduction. Describe the pathophysiology of multiple sclerosis and Guillain-Barré syndrome. Prof. M. Andrews © 2023 Skeletal Muscle Required Reading: ▪ Boron and Boulpaep Concise Medical Physiology. 1st ed., 2021. Chapters 6-7 Prof. M. Andrews © 2023 Skeletal Muscle Membrane potential and action potential in neurons https://www.youtube.com/watch?v=oa6rvUJlg7o Prof. M. Andrews © 2023 Skeletal Muscle Membrane Potential Potential (voltage) difference that exists across the cell membranes Established by diffusion potentials, which result from the concentration differences for various ions across the cell membrane Guyton and Hall Textbook of Medical Physiology, 14 th ed, 2021 Prof. M. Andrews © 2023 Skeletal Muscle Membrane Potential I. Diffusion Potential: The potential difference created across a membrane due to the diffusion of an ion down its concentration gradient Two conditions for diffusion of an ion: Permeability to the ion Chemical gradient of the ion Prof. M. Andrews © 2023 Skeletal Muscle Membrane Potential Concentrations of common ions across resting cell membrane Ions Intracellular Extracellular concentration concentration (mmol/L) (mmol/L) Na+ 12 145 K+ 155 4 Cl- 4 130 Ca2+ 0.0001 1 (free) Cells like salty bananas Prof. M. Andrews © 2023 Skeletal Muscle Membrane Potential II. Equilibrium Potential The diffusion potential at which there is no net diffusion of the ion Driving force generated by chemical gradient = driving force generated by electrical gradient, but the two forces are opposite on the ion Generation of a Na+ diffusion potential Linda S. Costanzo, Physiology, 7 thed., 2022 Prof. M. Andrews © 2023 Skeletal Muscle Membrane Potential III. Nernst Equation: used to calculate the equilibrium potential (Nernst potential) E = Equilibrium potential (mV) 2.3RT/F = Constant (60 mV at 37°C) Z = Charge on the ion (+1 for Na+; +2 for Ca2+; -1 for Cl-) Ci = Intracellular concentration (mmol/L) Ce = Extracellular concentration (mmol/L) E= Prof. M. Andrews © 2023 Skeletal Muscle Membrane Potential III. Nernst Equation: used to calculate the equilibrium potential (Nernst potential) Note: ▪ Nernst equation is used to calculate the equilibrium potential for an individual ion ▪ An ion’s equilibrium potential represents the membrane potential at which an ion will be at electrochemical equilibrium e.g. EK = -94 mV, if the membrane potential of a neuron is -94 mV, and membrane is K+ permeable, there is no net movement of K+ (at electrochemical equilibrium) ▪ The equilibrium potential is determined by the concentration gradient of an ion, and will change with alterations in the concentration gradient Prof. M. Andrews © 2023 Skeletal Muscle Membrane Potential If a muscle cell has a potassium extracellular concentration of 4 mM, and intracellular concentration of 140 mM , what is the potassium equilibrium potential? E= EK = -60 log(140/4) EK = -60 log(35) EK = -93 mV Prof. M. Andrews © 2023 Skeletal Muscle Membrane Potential Equilibrium potentials of common ions (Nernst potentials) ENa+ = +65 mV EK+ = -95 mV ECl- = -90 mV ECa2+ = +120 mV Prof. M. Andrews © 2023 Skeletal Muscle Membrane Potential IV.Goldman Equation Em Em: membrane potential (mV) P: membrane permeability to the ion Ci: concentration of the ion inside of the cell Co: concentration of the ion outside of the cell Note: ions with large concentration gradients and high membrane permeability contribute the most to membrane potential Prof. M. Andrews © 2023 Skeletal Muscle Resting Membrane Potential Resting membrane potential (RMP) is the potential difference that exists across the cell membranes when cells are at rest Ranges of RMP: ▪ Animal cells: -20 to -120 mV ▪ Muscle cells and neurons: -50 to -90 mV RMP is close to K+ equilibrium potential because the membrane has high permeability to K+ at rest (via K+ channels or K+ leak channels) RMP is mostly generated by K+ diffusion across the membrane (K+ efflux) Prof. M. Andrews © 2023 Skeletal Muscle Resting Membrane Potential Physiologically, RMP is very close to K+ equilibrium potential Changes in K+ permeability and/or K+ chemical gradient affect RMP ▪ ↑ K+ permeability → more negative membrane potential (hyperpolarization) ▪ ↓ K+ permeability → less negative membrane potential (depolarization) ▪ ↑ plasma [K+] → less negative membrane potential ▪ ↓ plasma [K+] → more negative membrane potential Terms related to membrane potential ▪ Polarization: resting state, membrane inside more negative than outside ▪ Depolarization: membrane potential less negative than RMP ▪ Hyperpolarization: membrane potential more negative than RMP Prof. M. Andrews © 2023 Skeletal Muscle Action Potentials in Excitable Cells An action potential is a regenerating depolarization of membrane potential that propagates along an excitable membrane Is an all-or-none event (need to reach threshold voltage-- usually 15 mV positive to resting potential) Is initiated by depolarization, can be induced in nerve and muscle by extrinsic stimulation Involves changes in membrane permeability Relies on voltage-gated ion channels Has constant amplitude and constant conduction velocity for a given fiber Prof. M. Andrews © 2023 Skeletal Muscle Action Potentials in Excitable Cells Overshoot I. Components of the Action Potential Depolarization: membrane potential becomes less negative or even positive (rising phase) Depolarization Overshoot: the part of an action potential where membrane potential is positive Repolarization: membrane potential returns to resting state (falling phase) Hyperpolarization (undershoot): membrane potential is more negative than RMP http://cnx.org/contents/SO2ufnKm@7/Cells-of-the-Nervous- System Prof. M. Andrews © 2023 Skeletal Muscle Action Potentials in Excitable Cells II. Ionic Basis of Action Potentials 1. Voltage-gated Na + channels At rest, activation gate closed, inactivation gate open, channels closed Membrane depolarization opens activation gate, channels open, inward Na + current Inactivation gate closed, channels closed, repolarization begins Slower response of inactivation gate to voltage change Outside Inside Guyton and Hall Textbook of Medical Physiology, 14th ed, 2021 Prof. M. Andrews © 2023 Skeletal Muscle Action Potentials in Excitable Cells II. Ionic Basis of Action Potentials 1. Voltage-gated Na+ channels Note ▪ Threshold potential: membrane potential at which an action potential is fired ▪ The membrane depolarization prior to reaching threshold is local potential or graded potential Amplitude of local potential depends on the strength of the stimulus Local potential is decrementing Local potentials can be summated to produce a large potential Prof. M. Andrews © 2023 Skeletal Muscle Action Potentials in Excitable Cells II. Ionic Basis of Action Potentials 2. Voltage-gated K+ channels Channels closed at rest Membrane depolarization opens channel, causing repolarization Slower response to voltage change Na+ channel closure and K+ channel open lead to rapid membrane repolarization Outside Inside Guyton and Hall Textbook of Medical Physiology, 14 th ed, 2021 Prof. M. Andrews © 2023 Skeletal Muscle Action Potentials in Excitable Cells II. Ionic Basis of Action Potentials 2. Voltage-gated K+ channels Hyperpolarization: membrane Na+ conductance returns to baseline, but K+ conductance remains elevated (slow closing to voltage change) Changes in ionic conductance underlying the action potential Boron and Boulpaep Concise Medical Physiology, 1st ed., 2021 Prof. M. Andrews © 2023 Skeletal Muscle Action Potentials in Excitable Cells II. Ionic Basis of Action Potentials Note: inward and outward currents (defined by the flow direction of positive charges conventionally) ▪ Inward current: positive charges moving from outside to inside of the cells: e.g. Na+ influx → inward current ▪ Outward current: positive charges moving from inside to outside of the cells: e.g. K+ efflux → outward current ▪ Flow of negative charges is defined in the opposite way: e.g. influx of Cl - → outward current Prof. M. Andrews © 2023 Skeletal Muscle Action Potentials in Excitable Cells II. Ionic Basis of Action Potentials 4. Refractory periods Absolute refractory period: ▪ Cells unable to fire a second action potential regardless of stimulus’ strength ▪ Why? Rising phase: insufficient Na+ channels available Falling phase: Na+ channels cannot reopen due to closure of inactivation gate (closed inactive state) Relative refractory period: ▪ Cells able to fire a second action potential with a stronger than normal stimulus (ms) ▪ Why? High K+ permeability opposes membrane depolarization Prof. M. Andrews © 2023 Skeletal Muscle Action Potentials in Excitable Cells Propagation of action potential III. Propagation of Action Potential 1. Propagation of action potential occurs by the spread of local current Local current is generated between depolarized and polarized regions This local current causes adjacent regions to fire action potentials Koeppen and Stanton: Berne & Levy Physiology, 8 th ed., 2024 Prof. M. Andrews © 2023 Skeletal Muscle Action Potentials in Excitable Cells Structure of a neuron III. Propagation of Action Potential Note: In neurons, action potentials are typically first generated at the initial segment of an axon and conducted unidirectionally toward the terminal due to ▪ Low threshold potential at the initial segment ▪ The highest density of voltage-gated Na+ channels at hillock and initial segment https://www.healthline.com/health/neurons#anato my Prof. M. Andrews © 2023 Skeletal Muscle Action Potentials in Excitable Cells III. Propagation of Action Potential 2. Conduction velocity of action potentials along a nerve Diameter of a nerve fiber: ▪ Action potential is conducted faster along fibers with large diameters Berne & Levy Physiology, 8th ed., 2024 Prof. M. Andrews © 2023 Skeletal Muscle Action Potentials in Excitable Cells III. Propagation of Action Potential Myelin sheath around an axon 2. Conduction velocity of action potentials along a nerve Myelination of the nerve fiber ▪ Myelination reduces membrane capacitance, allowing faster membrane depolarization ▪ Myelination increases membrane resistance, forcing current to flow along the low-resistance path (i.e. node of Ranvier) ▪ Saltatory conduction: action potential jumps from one node of Ranvier to the next Note: myelin sheath is provided by oligodendrocytes in CNS, and Schwann cells in PNS Berne & Levy Physiology, 8th ed., 2024 Prof. M. Andrews © 2023 Skeletal Muscle Action Potentials in Excitable Cells Recording of action potentials at nodes of Ranvier For myelinated nerves ▪ Node of Ranvier has high density of voltage-gated Na+ channels ▪ Action potential is regenerated at each node ▪ Action potential is conducted in a saltatory manner and conduction velocity is greatly increased by my