UNIT I FUNDAMENTALS OF BIOCHEMISTRY (2) PDF
Document Details
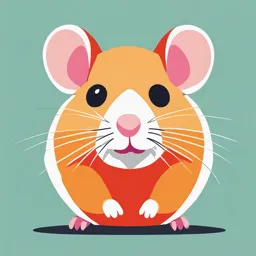
Uploaded by JawDroppingCerium
Jawaharlal Nehru Technological University Hyderabad
Prof. Sesha Srinivas Vutukuru
Tags
Related
- Biochemistry for Nurses PDF
- Introduction To Biochemistry PDF
- BIO 024 Biochemistry/Biomolecules Module 1 PDF
- Biochemistry Review - A New Approach for Diagnosis of Systemic and Oral Diseases Based on Salivary Biomolecules (PDF)
- Biochemistry Manual - Structure and Function of Biomolecules PDF
- Biochemistry PDF
Summary
This document is a lecture presentation on the Fundamentals of Biochemistry. It covers various aspects of biochemistry, including the overview, scope of biochemistry, and the role in disease understanding and treatment. It examines the principles of biochemistry and its impact on other fields.
Full Transcript
B.TECH BIOTECHNOLOGY BT301 PC: BIOCHEMISTRY Prof. Sesha Srinivas Vutukuru, M.Sc., M.Tech., Ph.D., PGDEM, PGD in Patent Law Guest Professor, Centre for Biotechnology, College for Engineering, Science and Technology, JNTUH Hyderabad PROFESSIONAL SUMMARY Academ...
B.TECH BIOTECHNOLOGY BT301 PC: BIOCHEMISTRY Prof. Sesha Srinivas Vutukuru, M.Sc., M.Tech., Ph.D., PGDEM, PGD in Patent Law Guest Professor, Centre for Biotechnology, College for Engineering, Science and Technology, JNTUH Hyderabad PROFESSIONAL SUMMARY Academics: I have over 24 years of teaching experience and taught core and allied courses in Biotechnology and Environmental Science to B. Tech and M. Tech students at SNIST, M.Sc. students at JNTUH Research: Received two major research grants from AICTE and DST worth Rs.28 lakhs. Actively involved in Consultancy projects in the area of Environmental Biotechnology, successfully guided 4 Ph.D. students under JNTUH, >10 M. Tech and > 35 B. Tech student projects, published 61 research papers (53 international and 08 national) in peer reviewed and indexed journals, Reviewer for >12 International Journals Google Scholar h- index 10, API score: 728, Total citations: 1243 Administration: Had 5 years of administrative as HoD, Chairman, Board of Studies, Member, Senior Management Team at SNIST, and gained adequate expertise in Curriculum Design and Reforms, Outcome Based Education, NBA accreditation system and NAAC, Creating a good R&D ecosystem, Grant Writing, and academic liaison. Courses taught B.Tech Animal Biotechnology, Animal Cell Science Technology, Biochemistry, Bioethics, Biosafety and IPR, Cell Biology, Microbiology, Industrial and Environmental Biotechnology, Environmental Studies, Intellectual Property Rights M.Tech Animal Biotechnology, Molecular Biology and Virology, General and Industrial Microbiology, Enzyme Engineering and Bioreactor Design, Environmental Microbiology and Biotechnology M.Sc Ecology and Ecotoxicology, Biochemistry, Biochemistry Lab, Biochemical and Biophysical Techniques Research Interests Environmental and Molecular Toxicology using zebra fish model Nanomaterials toxicology Oxidative stress Endocrine disruption Cancer Research using in vitro studies Biomarkers of exposure to heavy metals Overview of Biochemistry Biochemistry is the study of chemical processes and substances within living organisms We can explore the molecular and chemical basis of life, focusing on the structure, function, and interactions of bio-macromolecules like proteins, nucleic acids, carbohydrates, and lipids Biochemistry helps us understand how cells and organisms work at a molecular level It integrates both biology and chemistry, allowing us to explore complex biological systems through a chemical lens. This domain essentially reveals the mechanisms of life, from cellular metabolism to gene expression and Scope of Biochemistry Understandi ng Disease Environme ntal Developing Sustainabil Treatments ity BIOCHEMIST RY Genetic Agricultur Engineerin al g and Applicatio Biotechnol ns Diagnost ogy ics and Biomark ers 1.Understanding Disease: Biochemistry provides insights into the molecular causes of diseases, such as cancer, diabetes, and genetic disorders. By studying biochemical pathways, We can identify abnormalities that lead to illness 2. Developing Treatments: Biochemical research is key to drug development and therapeutic interventions. By targeting specific molecules or pathways, new medications and treatments can be designed to combat diseases more effectively 3. Genetic Engineering and Biotechnology: Biochemistry allows scientists to manipulate DNA and proteins to create genetically modified organisms (GMOs) or develop novel biotechnological applications 4. Diagnostics and Biomarkers: Biochemistry plays a crucial role in medical diagnostics. By identifying biomarkers—specific molecules indicative of certain diseases—medical professionals can diagnose conditions more accurately and earlier 5. Agricultural and Environmental Applications: Biochemistry contributes to advancements in agriculture through improved crop yields and resistance to pests. It also helps address environmental challenges through bioremediation and understanding ecological interactions at a molecular level Foundational Knowledge for Other Fields: Biochemistry provides the foundation for other scientific disciplines, such as genetics, molecular biology, pharmacology, immunology, and neuroscience. This interdisciplinary approach makes biochemistry a cornerstone of modern science and medicine. Overall, biochemistry is a dynamic and evolving field that continues to play a crucial role in advancing our understanding of life and thereby improving human health and well-being Its applications extend across various industries, making it an indispensable area of study and research Career Paths in Biochemistry Healthcare and Medicine Clinical Biochemist: Work in hospitals, clinics, or diagnostic laboratories, analyzing patient samples and helping diagnose medical conditions. Pharmacist: Combine knowledge of biochemistry and pharmacology to advise on the appropriate use of medications and contribute to drug development. Pharmaceutical and Biotechnology Industries Pharmaceutical Scientist: Work in drug development, testing, and production. This role involves designing, synthesizing, and analyzing pharmaceuticals for safety and efficacy. Biotechnologist: Develop products or technologies based on biological processes, such as genetically engineered crops, biofuels, or bioplastics. Environmental and Agricultural Sciences Environmental Biochemist: Focus on environmental issues like pollution, bioremediation, and the impact of chemicals on ecosystems. Agricultural Scientist: Work on improving crop yields, pest resistance, or animal health through biochemical research. Government and Regulatory Agencies Regulatory Affairs Specialist: Ensure that products and processes in the pharmaceutical and biotechnology industries comply with government regulations Public Health Official: Use biochemistry to study public health issues and develop policies or interventions to improve community health Science Communication and Education Science Writer/Communicator: Create educational content about biochemistry for a general audience, whether for scientific publications, media, or educational platforms. Teacher: Teach biology or chemistry at the UG/PG level, using a strong understanding of biochemistry to inform curriculum development Business and Management Project Manager: Oversee projects in pharmaceutical or biotechnology companies, requiring a blend of scientific knowledge and organizational skills. Biotech Entrepreneur: Start a biotechnology company, focusing on innovation and commercial applications of biochemistry. Unit I: FUNDAMENTALS OF BIOCHEMISTRY Contents Water pH pK Buffers Covalent and Non-covalent Interactions Oxido-reduction reactions Colligative properties CO1: Understand the basics of Biochemistry and Bioenergetics Water Water molecule is fundamentally composed of two hydrogen atoms and one oxygen atom (H₂O), is the most abundant and essential substance on Earth It is the elixir of life and plays a pivotal role in various natural and Water accounts biological for about 60-75% of a human’s weight (In some processes cases Blood-83%, Bone 22%) A loss of just 4% of total body water leads to dehydration, and a loss of 15% can be fatal Water is one of the most important resources gifted to us after the air. It forms the basis of life for every living organism on earth Life without water is unimaginable and impossible This is the reason why we are exploring planets to finding Interesting Facts traces or signs About Water of water Planet Earth consists of 78% water, and the rest constitutes land While 97% of the water on earth is salt water, freshwater constitutes only 3% More than 69% of this freshwater is trapped in glaciers Only 0.01% of the water available is fresh water(drinkable/potable) World There Water are two primary day sources is celebrated of water on 22nd March– surface water and every year groundwater. Uses: drinking, cooking, washing, farming, and other commercial purposes These primary water sources depend on rainfall and snow, which form a part of the hydrological cycle The water cycle or hydrologic cycle, describes where water is stored on Earth and how it moves. Water is stored in the atmosphere, on the land surface, and below the ground. It Biochemical significance of Water Water plays a pivotal role in various natural and biological processes. Some of the significant aspects of water are: 1. Molecular Structure and Properties 2. Hydrogen Bonding 3. Thermal Properties 4. Solvent Properties 5. Role in Life and Ecosystems 6. Biochemical Reactions 7. Environmental Significance 1. Molecular Structure and Properties Water is a simple molecule composed of two small, positively charged hydrogen atoms and one large negatively charged oxygen atom When the hydrogens bind to the oxygen, it creates an asymmetrical molecule with positive charge on one side and negative charge on the other side This charge differential is called polarity and dictates how water interacts with other molecules. The angle between the hydrogen atoms and the oxygen atom, along with the distribution of electrons, gives water its polarity 2. Hydrogen Bonding Hydrogen bonds are attractions of electrostatic force caused by the difference in charge between slightly positive hydrogen ions and other, slightly negative ions In the case of water, hydrogen bonds form between neighboring hydrogen and oxygen atoms of adjacent water molecules, creating a cohesive network This cohesion contributes to water's high surface tension 3. Thermal Properties Water has a high specific heat capacity, allowing it to absorb and retain heat This characteristic helps to maintain stable temperatures in biological systems, which is essential for enzymatic reactions and other biochemical processes Water's high heat of vaporization is crucial for processes like perspiration, where heat is released as water evaporates from the skin 4. Solvent Properties Water's polarity allows it to dissolve various substances, from salts to organic compounds. Hence, it is called Universal solvent This solvent property is critical in biochemical processes, enabling the transportation of nutrients, hormones, and waste products in living organisms. It also plays a vital role Water interacts in weathering andbest withinother erosion polar processes geological molecules, such as itself. The positive hydrogen of one water molecule will bond with the negative oxygen of the adjacent molecule, whose own hydrogens are attracted to the next oxygen, and so on. This bonding makes water molecules stick together in a property called cohesion The Cohesion also contributes to water’s high boiling point 5. Role in Life and Ecosystems Water plays a crucial part in most biological functions as a solvent Several waste materials are also eliminated as a solution via urination and sweat. Water aids in maintaining and regulating body temperature. Flushing waste from the body Transportation of nutrients and is necessary for digestion People get about 20 percent of their daily water intake from food. The rest is dependent on drinking water and 6. Biochemical Reactions Water Supports Cellular Structure Water has an important structural role in biology. Water impacts cell shape. Water creates pressure inside the cell that helps it maintain shape. In the hydrated cell (left), the water pushes outward and the cell maintains a round shape. In the dehydrated cell, there is less water pushing outward so the cell becomes wrinkled Water contributes to the formation of membranes surrounding cells Every cell on Earth is surrounded by a membrane, most of which are formed by two layers of molecules called phospholipids (Figure). The phospholipids, like water, have two distinct components: a polar “head” and a nonpolar “tail.” The polar heads interact with water, while the nonpolar tails try to avoid water and interact with each other instead. Water also impacts some fundamental components of every cell: DNA and proteins. Proteins are produced as a long chain of building blocks called amino acids and need to fold into a specific shape to function correctly Water drives the folding of amino acid chains as different types of amino acids seek and avoid interacting with water Water molecules surround DNA in an ordered fashion to support its characteristic double-helix conformation. Without this shape, cells would be unable to follow the careful instructions encoded by DNA or to pass the instructions onto future cells, making human growth, reproduction, and, Water buffers cells from the dangerous effects of acids and bases. Highly acidic or basic substances, like bleach or hydrochloric acid, are corrosive to even the most durable materials. This is because acids and bases release excess hydrogens or take up excess hydrogens, respectively, from the surrounding materials. Losing or gaining positively-charged hydrogens disrupts the structure of molecules. Water does this by acting as both an acid and a base. Although the chemical bonds within a water molecule are very stable, it’s possible for a water molecule to give up a hydrogen and become OH–, thus acting as a base, or accept another hydrogen and become H3O+, thus acting as an acid. This adaptability allows water to combat drastic changes of pH due to acidic or basic substances in the body in a process called buffering. 7. Environmental Significance Water plays a crucial role in shaping Earth's landscape through erosion and sedimentation It is also a key factor in weather patterns, ocean currents, and climate regulation The distribution and availability of fresh water impact ecosystems, agriculture, and human settlements. Overall, water's properties as a solvent, its ability to form hydrogen bonds, its thermal characteristics, and its involvement in chemical reactions make it a crucial component in the structure, function, and regulation of biochemical processes Carbon: Significance in Biochemistry Carbon (C), is a fundamental chemical element with the atomic number 6 It is an essential compound and plays a significant role in chemistry, biology, and environmental science It is the 4th most abundant element after hydrogen, helium, oxygen Compounds that contain carbon are called organic Carbon is nonmetallic and compoundsin nature, has four tetravalent valence (outer) electrons, six protons, and six neutrons Versatile Bonding: Carbon can form four covalent bonds with itself and other atoms like hydrogen, oxygen, phosphorus, nitrogen, and many others elements Carbon forms single bonds, double bonds, and triple bonds to create a wide variety of biomolecules This versatility lets it form chains, rings, branches, and other configurations, which are fundamental to the structure of biomolecules Carbon is capable of forming bonds because the outer valence of a carbon molecule holds 4 electrons but needs 8 to be filled. Hence, it will share the remaining electrons with other atoms to fill its outer valence Formation of Functional Groups Carbon-based compounds can include various functional groups, like hydroxyl (-OH), carboxyl (-COOH), amino (-NH2), and phosphate (-PO4). These groups contribute to the chemical reactivity and Carbon-Based Signaling functionality Molecules of biomolecules Many hormones and neurotransmitters are carbon-based These signaling molecules regulate a wide range of physiological processes and are central to communication within and between cells Saturated Carbon Unsaturated Carbon Compounds Compounds Carbon compounds, Atoms that are only satisfied by the single satisfied either by bond between them, are double or triple bonds called saturated are called unsaturated compounds. carbon compounds One of the examples of One of the examples of this this is Ethene - C2H4, is Ethane - C2H6. Here, the which is an octet or duplet of both the unsaturated carbon atoms is fully completed compound, having a only by a single bond. double bond Biological Role of Carbon The oxygen (O2) you breathe in is dependent on how much carbon dioxide (CO2) plants take in. Carbon dioxide and oxygen are cycled back and forth in the carbon cycle. potential of hydrogen (pH) The letters pH stand for potential of hydrogen, since pH is effectively a measure of the concentration of hydrogen ions (that is, protons) in a substance The pH scale was devised in 1923 by Danish biochemist Soren Peter Lauritz Sorensen (1868–1969) pH is defined as the negative logarithm of H+ ion concentration. Hence the meaning of the name pH is justified as the potential of hydrogen The pH scale measures how acidic/basic a solution is Solutions having a value of pH ranging from 0 to 7 on the pH scale are termed as acidic and the value of pH ranging from 7 to 14 on pH scale are known as basic solutions. Solutions having the value of pH equal to 7 on pH scale are known as neutral solutions. Significance of pH in biochemistry pH is a critical parameter in biochemistry, and influences various biological systems and processes 1. Enzyme Activity Enzymes are proteins that catalyze biochemical reactions, and their activity is highly sensitive to pH Each enzyme has an optimal pH range where it functions best. Outside this range, the enzyme's activity may decrease due to changes in its structure or active site 2. Protein Structure and Stability Proteins are composed of amino acids with various side chains that can be charged, neutral, or hydrophobic. The ionization of these side chains depends on pH, affecting protein folding, structure, and stability. Changes in pH can lead to denaturation, where proteins lose their three-dimensional 3. Cellular Function Cells maintain a tightly regulated internal pH for proper functioning Cellular processes like signal transduction, metabolism, and energy production depends on a specific pH range Disruption 4. Membrane in pH homeostasis can lead to cellular dysfunction Transport or death pH gradients across cell membranes drive the transport of ions and molecules For example, the proton gradient in mitochondria, maintained by varying pH across the inner membrane, is 5. Metabolism crucial for ATP synthesis in oxidative phosphorylation Metabolic pathways are sensitive to pH changes. The pH in different cellular compartments, like lysosomes (acidic) and cytosol (neutral), is optimized for specific metabolic processes. This ensures proper functioning of various biochemical pathways 6. DNA and RNA Stability The stability and structure of nucleic acids like DNA and RNA are influenced by pH. Extremes in pH can lead to denaturation or degradation, affecting processes like transcription and replication. Acid-Base Balance in Organisms Organisms have mechanisms to maintain acid-base balance in body fluids For example, the human body regulates blood pH within a narrow range to ensure proper physiological functions Disorders in acid-base balance can lead to conditions like Biochemical acidosisAssays and Experiments or alkalosis In research, pH is critical in biochemical assays, where maintaining specific pH levels is necessary for accurate results Buffer systems are often used to control pH in experimental setups What is pKa is a standard pK a? to specify used the nature of the solution It is generally used to estimate the acidity of a solution If the pKa of a solution is less, the proton would be less tightly bound; hence the solution would be more acidic Are pH and pKa the same? Though pH and pKa are associated, pKa is are more explicit pKa signifies how a molecule will behave at a certain pH Primarily, pKa determines at what pH a solution will effectively lose or gain proton The pK value, also known as the pKa value, is a quantitative measure that represents the acidity of a particular hydrogen atom in a molecule. It is the negative base -10 logarithm of the acid dissociation constant (Ka) of a solution. The formula for calculating pKa is: pKa=−log10Ka Acid Dissociation Constant (Ka): This constant measures the strength of an acid in solution. It is the equilibrium constant for the dissociation of a weak acid into its conjugate base and a proton (H+) For a generic acid (HA), the dissociation can be represented as: HA⇋H++A The expression for 𝐾𝑎 is: 𝐾𝑎=[H+][A−] [HA] How are pH and pKa related? Henderson-Hasselbalch has given a relation between pH and pKa. He said pH = pKa + log([salt]/[Acid]) A lower pKa value indicates a stronger acid, which means it more readily donates protons (H+). Conversely, a higher pKa value indicates a weaker acid Applications of pKa in Biochemistry: pKa values are crucial in understanding enzyme activity, drug design, and the behavior of biomolecules like amino acids and nucleotides, which contain acidic or basic groups. Pharmaceuticals: The pKa of a drug affects its absorption, distribution, metabolism, and excretion (ADME) properties Analytical Chemistry: pKa values help in choosing appropriate pH conditions for chemical reactions, separation techniques, and titration analysis Buffers Buffers are solutions that resist changes in pH when small amounts of acid or base are added They are essential in many chemical and biological systems to maintain stable pH conditions Components of a Buffer Weak Acid and Its Conjugate Base: A common example is acetic acid (CH₃COOH) and its conjugate base, acetate (CH₃COO⁻). Weak Base and Its Conjugate Acid: An example is ammonia (NH₃) and its conjugate acid, ammonium (NH₄⁺). How Buffers Work Buffers work based on the equilibrium between the weak acid and its conjugate base (or weak base and its conjugate acid) When an acid (H⁺) is added to the buffer, it reacts with the conjugate base to form the weak acid, minimizing the change in pH When a base (OH⁻) is added, it reacts with the weak acid Buffer Equation to form water and the conjugate base, again minimizing The effectiveness of a buffer is described by the Henderson- the change Hasselbalch in pH equation: pH= pKa+log ([A−]/[HA]) Where: pH is the hydrogen ion concentration pKa is the acid dissociation constant. [A−] is the concentration of the conjugate base. [HA] is the concentration of the weak acid. Buffer Capacity Buffer capacity refers to the amount of acid or base that can be added to a buffer solution without causing a significant change in pH. It depends on the concentrations of the acid and its Examples of Buffer Systems conjugate base in the buffer. Acetic Acid/Acetate Buffer: Components: Acetic acid (CH₃COOH) and sodium acetate (CH₃COONa). pKa: Approximately 4.76. Usage: Often used in biochemical experiments. Phosphate Buffer: Components: Dihydrogen phosphate (H2PO4) and hydrogen phosphate (HPO42−). pKa: Around 7.2. Usage: Commonly used in biological systems due to its effective pH range close to physiological pH. Bicarbonate Buffer: Components: Carbonic acid (H₂CO₃) and bicarbonate (HCO₃⁻) Covalent Interactions Covalent interactions involve the sharing of electron pairs between atoms. This type of bond is strong and forms the basis of the structure of molecules. Characteristics of Covalent Bonds Electron Sharing: Covalent bonds occur when two atoms share one or more pairs of electrons. Each atom contributes at least one electron to the bond. Bond Strength: Covalent bonds are generally very strong, with bond energies typically ranging from 50 to 200 kcal/mol. Bond Length: The distance between the nuclei of the bonded atoms. This distance is specific to the types of atoms involved. Bond Polarity: If the atoms involved have different electronegativities, Examples Single Bond: In a molecule of hydrogen (H₂), the two hydrogen atoms share a single pair of electrons Double Bond: In a molecule of oxygen (O₂), the two oxygen atoms share two pairs of electrons Triple Bond: In a molecule of nitrogen (N₂), the two nitrogen atoms share three pairs of electrons Non-Covalent Interactions Non-covalent interactions do not involve the sharing of electron pairs. These interactions are typically weaker than covalent bonds but are crucial for the structure and function of large molecules, such as proteins and DNA. Types of Non-Covalent Interactions Ionic Bonds: These occur between oppositely charged ions. For example, in sodium chloride (NaCl), the sodium ion (Na⁺) and chloride ion (Cl⁻) are held together by electrostatic attraction. Hydrogen Bonds: These occur when a hydrogen atom covalently bonded to an electronegative atom (like oxygen or nitrogen) is attracted to another electronegative atom. For example, the hydrogen bonds between water molecules (H₂O). Van der Waals Forces: These include London dispersion forces and dipole-dipole interactions. They are weak, short-range forces that arise from temporary dipoles in molecules. London Dispersion Forces: These are the weakest intermolecular forces, caused by the correlated movements of electrons in interacting molecules or atoms. Dipole-Dipole Interactions: These occur between polar molecules, where the positive end of one molecule is attracted to the negative end of another. Hydrophobic Interactions: These occur when nonpolar molecules or regions of molecules aggregate in an aqueous solution to minimize their exposure to water. Characteristics of Non-Covalent Bonds Weaker than Covalent Bonds: Non-covalent interactions are typically much weaker than covalent bonds, with bond energies ranging from 1 to 10 kcal/mol. Reversible: These interactions can be easily formed and broken, which is essential for dynamic biological processes. Specificity and Complementarity: Non-covalent interactions play a crucial role in the specificity and complementarity of molecular recognition, such as enzyme-substrate binding and antigen-antibody interactions. Examples Ionic Bond: The attraction between Na⁺ and Cl⁻ ions in table salt. Hydrogen Bond: The bonds that hold together the two strands of DNA through interactions between the bases (adenine with thymine and cytosine with guanine). Van der Waals Forces: The interactions between noble gas atoms in liquid helium. Hydrophobic Interaction: The tendency of oil molecules to clump together in water. BIOENERGETICS Bioenergetics is a field of biology that examines the flow of energy through living systems, such as cells and organisms This discipline combines principles of biology and chemistry to understand how biological processes, such as metabolism and cellular respiration, produce and use energy Key Concepts in Bioenergetics ATP (Adenosine Triphosphate): ATP is the primary energy currency of the cell It stores and provides energy for various cellular processes Energy is released when ATP is hydrolyzed to ADP (Adenosine Diphosphate) and inorganic phosphate Metabolism: Metabolism includes all chemical reactions within cells that maintain life. It is divided into catabolism (breaking down molecules to produce energy) and anabolism (building complex molecules from simpler ones) Cellular Respiration: This is the process by which cells convert glucose and oxygen into ATP, carbon dioxide, and water It involves three main stages: Glycolysis, the Citric acid cycle (Krebs cycle), and Oxidative phosphorylation (electron Photosynthesis: This process occurs in plants, algae, and some bacteria, converting light energy into chemical energy stored in glucose It consists of light-dependent reactions and the Calvin cycle (light-independent reactions) Mitochondria and Chloroplasts: Mitochondria are the powerhouses of the cell, where cellular respiration takes place Chloroplasts are the sites of photosynthesis in plant cells and algae Cellular Respiration Glycolysis: Occurs in the cytoplasm Converts one molecule of glucose into two molecules of pyruvate, producing a net gain of 2 ATP and 2 NADH molecules Citric Acid Cycle (Krebs Cycle): Takes place in the mitochondrial matrix Each acetyl-CoA (derived from pyruvate) enters the cycle, resulting in the production of ATP, NADH, and FADH2 Oxidative Phosphorylation: Occurs across the inner mitochondrial membrane Electrons from NADH and FADH2 travel through the electron transport chain, leading to the creation of a proton gradient that drives ATP synthesis by ATP synthase Photosynthesis Light-Dependent Reactions Occur in the thylakoid membranes of chloroplasts Convert light energy into chemical energy in the form of ATP and NADPH Calvin Cycle: Takes place in the stroma of chloroplasts Uses ATP and NADPH to convert carbon dioxide into glucose Thermodynamics in Bioenergetics First Law of Thermodynamics: Energy cannot be created or destroyed, only transformed from one form to another form Second Law of Thermodynamics: Energy transfer leads to increased entropy (disorder) Bioenergetic Pathways Aerobic Pathways: Require oxygen (e.g., oxidative phosphorylation) Anaerobic Pathways: Do not require oxygen (e.g., fermentation) Importance of Bioenergetics Understanding bioenergetics is crucial for insights into how cells function, grow, and respond to their environment It has applications in medicine, agriculture, and biotechnology, such as in the development of treatments for metabolic disorders and the optimization of crop yields By studying the principles and processes of bioenergetics, scientists can gain a deeper understanding of life at the molecular level, paving the way for advancements in