Cell Energy - Photosynthesis and Cellular Respiration
Document Details
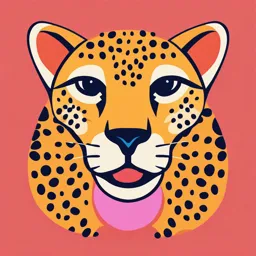
Uploaded by PleasingAntigorite7063
Youngstown State University
Tags
Related
Summary
These are student notes on the principles of cell energy, covering the role of ATP in cellular respiration, photosynthesis, and how these metabolic reactions work. The notes cover glycolysis, the Krebs cycle, the electron transport chain, and how energy is captured by plants.
Full Transcript
How Cells Harvest Energy Week 3: Cell Energy A primary function of the capsid is to protect the viral genome from environmental conditions and ultimately to deliver the genome to the interior of a homologous host cell. 2 Capsids Definition:...
How Cells Harvest Energy Week 3: Cell Energy A primary function of the capsid is to protect the viral genome from environmental conditions and ultimately to deliver the genome to the interior of a homologous host cell. 2 Capsids Definition: _________________________________________ ___________________ Can have various shapes: icosahedral, helical, or complex. Function: _________________________________________ ___________________ _________________________________________ ___________________ 3 Capsids & Energy Model for Nano-Biotechnology: ____________________________________________________________ ____________________________________________________________ Energy Transfer in Viral Infections: ____________________________________________________________ ____________________________________________________________ Studying Protein Dynamics: ____________________________________________________________ ____________________________________________________________ Applications in Synthetic Biology: ____________________________________________________________ ____________________________________________________________ 4 Virus Capsid vs. Capsomere Capsid: ____________________________________________ ________________ ____________________________________________ ________________ ____________________________________________ ________________ Capsomere: ____________________________________________ ________________ ____________________________________________ ________________ 5 Microtubules ____________________________________________________ ____________________________________________________ ___________________________________________________ ____________________________________________________ ___________________________________________________ ____________________________________________________ ___________________________________________________ ___________________________________________________ 6 Microtubules ____________________________________________________ _______________________________________________________ ________________________________________________ _______________________________________________________ ________________________________________________ ___________________________________________________ Polymerization and Depolymerization: ____________________________________ ________________ ____________________________________ _______________ Organization: ____________________________________ ________________ ____________________________________ 7 _______________ Microtubule Functions Structural Support: ____________________________________________________ ___________________________________________________ Intracellular Transport: ____________________________________________________ ___________________________________________________ Cell Division: ____________________________________________________ ___________________________________________________ Cilia and Flagella Movement: ____________________________________________________ ___________________________________________________ 8 Microtubules & Energy Relation to Energy ____________________________________________________ ___________________________________________________ Motor Proteins: ____________________________________________________ ___________________________________________________ Dynamic Instability: ____________________________________________________ ___________________________________________________ Cellular Work: ____________________________________________________ ___________________________________________________ ____________________________________________________ ___________________________________________________ 9 The First Law of Thermodynamics The First Law of Thermodynamics, also known as the ___________________states that: Energy cannot be created or destroyed, only transformed or transferred from one form to another. ___________________________________ _________________ ___________________________________ ________________ 10 The Second Law of Thermodynamics The Second Law of Thermodynamics states that: In any energy transfer or transformation, the total entropy (disorder) of a system and its surroundings will increase over time. ____________________________________________________ ______________________________________________________________________ _________________________________ ___________________________________________________ ___________________________________________________ 11 Figure 2-38 Molecular Biology of the Cell (© Garland Science 2008) The Second Law of Thermodynamics ____________________________________________ _ ____________________________________________ ____________________________________________ __ ____________________________________________ ____________________________________________ __ ____________________________________________ ____________________________________________ __ ____________________________________________ _ 12 Figure 2-38 Molecular Biology of the Cell (© Garland Science 2008) Potential and Kinetic Energy Potential energy is stored energy Kinetic energy is the energy of motion, including heat. 13 Figure 2-39 (part 1 of 4) Molecular Biology of the Cell (© Garland Science 2008) Cellular Energy in Metabolism __________________________ __________________________ __________________________ __________________________ __________________________ _________________________ __________________________ __________________________ __________________________ __________________________ 14 Figure 2-36 Molecular Biology of the Cell (© Garland Science 2008) Anabolism Anabolism is one of the key metabolic pathways in cellular biology and refers to _______________________________________________ _______________________________________________. _______________________________________________ Anabolism plays a central role in energy utilization within cells because it requires energy to construct macromolecules such as proteins, nucleic acids, lipids, and carbohydrates. _______________________________________________ _______________________________________________. _______________________________________________ _______________________________________________ _______________________________________________. _______________________________________________ 15 Anabolism _________________________________________________ _________________________________________________ _________________________________________________ _________________________________________________ _________________________________________________ _________________________________________________ _________________________________________________ _______________________________________________ For example: _______ supplies the energy needed to drive the synthesis of molecules by phosphorylating intermediates. _______ provides reducing power in biosynthetic reactions, such as fatty acid synthesis. 16 Anabolism Anabolism is crucial for: _________________: Building tissues and expanding cell size. _________________: Replacing damaged molecules and organelles. _________________: Synthesis of energy-rich molecules like glycogen and triglycerides for later use. Regulation of Anabolism: Cells regulate anabolic pathways to balance energy expenditure with energy availability. This regulation occurs through: _________________:, such as insulin (which promotes anabolic processes like glycogen and protein synthesis). _________________:, where end-products of anabolic pathways inhibit the enzymes involved in their synthesis. 17 Catabolic Catabolism is one of the key components of cellular metabolism, referring to the breakdown of complex molecules into simpler ones. __________________________________ __________________________________ __________________________________ __________________________________ __________________________________ __________________________________ __________________________________ __________________________________ 18 Catabolic __________________________________ Catabolic reactions are exergonic, meaning they release energy as complex molecules (e.g., carbohydrates, lipids, and proteins) are broken down into simpler ones like glucose, fatty acids, and amino acids. Energy Carriers: ____________________________________________________ _ _____________________________________________________ _____________________________________________________ _____________________________________________________ _____________________________________________________ 19 Types of Catabolic Pathways: Glycolysis: _____________________________________________________ Citric Acid Cycle (Krebs Cycle): _____________________________________________________ _____________________________________________________ Oxidative Phosphorylation: _____________________________________________________ Beta-Oxidation: _____________________________________________________ 20 Catabolism's Role in Energy Production: Provides the __________ required for cellular processes, such as: __________ __________ __________ Powers __________ ensuring the synthesis of essential biomolecules. Carbohydrate Breakdown: ______________________________________________________ Protein Catabolism: ______________________________________________________ Fat Catabolism: ______________________________________________________ 21 Importance of Catabolism ___________ : Catabolism is the primary source of energy for cellular activities, ensuring survival and function. ___________ : Maintains energy balance by regulating the production and consumption of ATP based on cellular demand. ___________ : In times of scarcity, cells prioritize catabolism of stored molecules (e.g., glycogen or fats) to meet energy needs. Hormonal Control: ___________ : Reduces catabolic activity (e.g., inhibits fat breakdown). ___________ and ___________ : Promote catabolism during low energy states (e.g., stimulates glycogenolysis and lipolysis). Feedback Mechanisms: ____________________________________________________ 22 Catabolism & Anabolism 23 Metabolism is the result of series of chemical reactions catalyzed by enzymes It is important to know that the chemical reactions of metabolic pathways do not take place on their own. Each reaction step is facilitated, or catalyzed, by a protein called an enzyme. 24 Figure 2-34 Molecular Biology of the Cell (© Garland Science 2008) Ultimate energy for most life is the sun The process of using sunlight as an energy source to build biological molecules is called photosynthesis ________________________________________________. ________________________________________________. ________________________________________________. ________________________________________________. 25 Figure 2-40 Molecular Biology of the Cell (© Garland Science 2008) ________________________________________________________ ________________________________________________________ ________________________________________________________ ________________________________________________________ ________________________________________________________ 26 Figure 2-42 Molecular Biology of the Cell (© Garland Science 2008) Energy and Thermodynamics in Cells The relationship between energy, heat, and disorder in cells is described by the equation: ∆G = ∆H – T∆S ____________________________________________ ____________________________________________ ____________________________________________ ____________________________________________ ____________________________________________ 27 27 Energy and Thermodynamics in Cells ______: Change in free energy (usable energy for cellular work). ______: Change in enthalpy (total energy, including heat). ______: : Temperature (in Kelvin). ______: : Change in entropy (disorder or randomness). The Equation Explains: The free energy available (∆G) depends on: __________________________________________ __________________________________________ Reactions occur spontaneously when ______________. 28 28 H = G + TS H= enthalpy or total energy G= free energy or amount of energy for work (Gibbs free energy) S= entropy or unusable energy T= absolute temperature in Kelvin (K) 29 29 Energy and Thermodynamics in Cells _____________:Breakdown of glucose releases energy (negative ∆G), used to form ATP. _____________:Building molecules requires energy (positive ∆G), driven by ATP hydrolysis. 30 30 Spontaneous reactions? Spontaneous reactions occur without the input of external energy. _______________________________________ Key factor is the free energy change ____________________________________________ __________________________________ 31 31 Spontaneous reactions? _______________ : Energy is released during the reaction. Example: Cellular respiration (breakdown of glucose). Driven by Thermodynamics: Spontaneity depends on: _______________ _______________ _______________ ______________________________________________ Not Instantaneous: Spontaneous does not mean "fast." Some 32 32 reactions require enzymes to lower the Exergonic Although exergonic reactions are said to occur spontaneously, this does not imply that the reaction will take place at an observable rate. 33 33 Endergonic An endergonic reaction will not take place on its own without adding free energy. Also called a heat absorbing nonspontaneous reaction or an unfavorable reaction. Is a chemical reaction in which the standard change in free energy is positive, and an additional driving force is needed to perform this reaction 34 34 35 Figure 2-44 Molecular Biology of the Cell (© Garland Science 2008) Enzymes and Ribozymes A spontaneous reaction is not necessarily a fast reaction __________ – an agent that speeds up the rate of a chemical reaction without being consumed during the reaction __________ – protein catalysts in living cells __________ – RNA molecules with catalytic properties 36 36 Activation energy Initial __________ to start reaction Allows molecules to get close enough to cause bond rearrangement Can now achieve __________ where bonds are stretched Common ways to overcome activation energy Large amounts of __________ Using __________ to lower activation energy 37 37 Copyright © The McGraw-Hill Companies, Inc. Permission required for reproduction or display. ATP Reactant molecules Glucose Enzyme Transition state Activation energy (EA) without enzyme Activation energy (EA) with enzyme Free energy (G) Reactants Change in free energy (G) Products 38 Progress of an exergonic reaction 38 How enzymes lower activation energy Straining bonds in reactants ________ ____________ Positioning reactants together ______________________ Changing local environment ________________________ 39 39 Other enzyme terminology __________– location where reaction takes place __________ – reactants that bind to active site ___________________– formed when enzyme and substrate bind 40 40 Substrate binding Enzymes have a high __________ for their substrate Lock and key metaphor __________ ______________________________ Induced fit phenomenon – __________ ______________________________ 41 41 Inhibition Competitive inhibition Molecule binds __________ Inhibits ability of substrate to bind Apparent KM __________ – more substrate needed Noncompetitive inhibition __________ Vmax without affecting Km Inhibitor binds to __________, not active site 42 42 Other requirements for enzymes _______________– small molecules permanently attached to the enzyme __________ – usually inorganic ion that temporarily binds to enzyme __________ – organic molecule that participates in reaction but is left unchanged afterward 43 43 Enzymes are affected by environment Most enzymes function maximally in a __________ of temperature and pH Copyright © The McGraw-Hill Companies, Inc. Permission required for reproduction or display. High chemical reaction Rate of a 0 0 10 20 30 40 50 60 44 Temperature (ºC) 44 How to Run Endergonic Reactions Forward in a Cell 1) Increase the energy in the reactants by coupling with an exergonic reaction 2) Keep the product concentration very low by a second highly energetically favorable reaction 3) Drive with a molecular machine that uses another energy source 45 Cells use ATP hydrolysis An endergonic reaction can be coupled to an exergonic reaction Endergonic reaction will be spontaneous if net free energy change for both processes is __________ 46 46 47 Table 2-5 Molecular Biology of the Cell (© Garland Science 2008) All reactions can run forward or backwards. The equilibrium constant which represents the relative concentration of reactants and products depends on ∆G. So endergonic reactions produce very low amounts of product. 48 Table 2-4 Molecular Biology of the Cell (© Garland Science 2008) Energy in Cells Figure 12.1 Overview of aerobic oxidation and photosynthesis. 49 Chemical Energy to Drive Metabolism Autotrophs _______________ _____________________ ______________ Heterotrophs _______________ _____________________ ______________ 50 Cellular Respiration A multi step process that converts the chemical energy in food into usable cellular energy in the form of adenosine triphosphate (ATP) Aimed at creating ATP ATP is the energy currency of cells, it is necessary for cellular function ATP produced mainly by the ___________________ ________________ are cellular organelles that synthesize ATP for our cells 51 Cellular Respiration Cells harvest energy by breaking bonds and shifting electrons from one molecule to another. ___________- final electron acceptor is oxygen ___________- final electron acceptor is inorganic molecule other than oxygen ___________- final electron acceptor is an organic molecule 52 ATP Adenosine Triphosphate (ATP) is the energy currency of the cell. used to drive movement used to drive endergonic reactions 53 ATP Most of the ATP produced in cells is made by the enzyme ____________. Enzyme is embedded in the membrane and provides a channel through which protons can cross the membrane down their concentration gradient. ATP synthesis is achieved by a rotary motor driven by a gradient of protons. 54 Glucose Catabolism Cells catabolize organic molecules and produce ATP in two ways: ____________________ ____________________ in most organisms, both are combined ____________________ ____________________ ____________________ ____________________ 55 How Cellular Respiration Works Inside The Mitrochondria Broken into 3 steps Glycolysis Krebs Cycle Electron Transport Chain 56 Glycolysis Glycolysis is the metabolic process that serves as the foundation for both aerobic and anaerobic cellular respiration. In glycolysis, _________ is converted into ______________. Glucose is a six- memebered ring molecule found in the blood and is usually a result of the breakdown of carbohydrates into sugars. It enters cells through specific transporter proteins that move it from outside the cell into the cell’s cytosol. All of the glycolytic enzymes are found in the ________. 57 58 Introduction to Glycolysis Definition: Glycolysis is the metabolic pathway that converts glucose into pyruvate to generate ATP. Location: Occurs in the ________ of cells. Phases: Glycolysis has two major phases: Energy Investment Phase __________________________________________ __________________________________________ Energy Payoff Phase __________________________________________ __________________________________________ End Products: __ pyruvate molecules Net ________(produced) ___________(electron carriers) 59 Step 1 – _____________________ Enzyme: _____________________ Reaction: Glucose + ATP → Glucose-6-Phosphate (G6P) + ADP Purpose: _________________________________________ _________________________________________ 60 Step 1 Hexokinase The first step in glycolysis is the conversion of D- glucose into glucose-6-phosphate. The enzyme that catalyzes this reaction is hexokinase. Glucose ring is phosphorylated. Phosphorylation is the process of adding a phosphate group to a molecule derived from ATP. As a result, at this point in glycolysis, 1 molecule of ATP has been consumed. 61 Step 2 – _________________________ Enzyme: _________________________ Glucose-6- Phosphate → Fructose-6-Phosphate Purpose: Converts a six-membered ring (glucose) into a five- membered ring (fructose) for better cleavage in later steps. 62 Step 2: Phosphoglucose Isomerase The second reaction of glycolysis is the rearrangement of glucose 6-phosphate (G6P) into fructose 6-phosphate. The reaction involves the rearrangement of the carbon-oxygen bond to transform the six- membered ring into a five-membered ring. To rearrangement takes place when the six- membered ring opens and then closes in such a way that the first carbon becomes now external to the ring. 63 Step 3 – ___________________________________ Enzyme: _______________________ Reaction: Fructose-6-Phosphate + ATP → Fructose-1,6-Bisphosphate + ADP Purpose: _______________________– commits glucose to glycolysis. _______________________enzyme in metabolism. 64 Step 3: Phosphofructokinase Phosphofructokinase, with magnesium as a cofactor, changes fructose 6-phosphate into fructose 1,6-bisphosphate. Similar to the reaction that occurs in step 1 of glycolysis, a second molecule of ATP provides the phosphate group that is added on to the F6P molecule. The enzyme that catalyzes this reaction is phosphofructokinase (PFK). As in step 1, a magnesium atom is involved to help shield negative charges. 65 Step 4 – _______________________ Enzyme: _______________________ Reaction: Fructose-1,6-Bisphosphate → Dihydroxyacetone Phosphate (DHAP) + Glyceraldehyde-3- Phosphate (G3P) Purpose: Splits the 6-carbon sugar into two _____________________ _______________________ 66 Step 4: Aldolase The enzyme Aldolase splits fructose 1, 6-bisphosphate into two sugars that are isomers of each other. These two sugars are dihydroxyacetone phosphate (DHAP) and glyceraldehyde 3-phosphate (GAP). This step utilizes the enzyme aldolase, which catalyzes the cleavage of FBP to yield two 3- carbon molecules. One of these molecules is called 67 Step 5 – _______________________ Enzyme: _______________________ Reaction: Dihydroxyacetone Phosphate (DHAP) → Glyceraldehyde-3- Phosphate (G3P) Purpose: _______________________ _______________________ 68 Step 5: Triosephosphate isomerase The enzyme triosephosphate isomerase rapidly inter- converts the molecules dihydroxyacetone phosphate (DHAP) and glyceraldehyde 3-phosphate (GAP). Glyceraldehyde phosphate is removed / used in next step of Glycolysis. GAP is the only molecule that continues in the glycolytic pathway. As a result, all of the DHAP molecules produced are further acted on by the enzyme Triosephosphate isomerase (TIM), which reorganizes the DHAP into 69 GAP so it can continue in glycolysis. Step 6 – _______________________ Enzyme: _______________________ Reaction: G3P + NAD⁺ + Pi → 1,3- Bisphosphoglycerate (1,3-BPG) + NADH Purpose: _______________________ _______________________ 70 Step 6: Glyceraldehyde-3- phosphate Dehydrogenase Glyceraldehyde-3-phosphate dehydrogenase (GAPDH) dehydrogenates and adds an inorganic phosphate to glyceraldehyde 3-phosphate, producing 1,3-bisphosphoglycerate. In this step, two main events take place: 1) glyceraldehyde-3-phosphate is oxidized by the coenzyme nicotinamide adenine dinucleotide (NAD); 2) the molecule is phosphorylated by the addition of a free phosphate group. The enzyme that catalyzes this reaction is glyceraldehyde-3- phosphate dehydrogenase (GAPDH). 71 Step 7 – _______________________Enzyme: Phosphoglycerate Kinase Reaction: 1,3-BPG + ADP → 3- Phosphoglycerate + ATP Purpose: Produces ______________________per glucose. ATP is made 72 Step 7: Phosphoglycerate Kinase Phosphoglycerate kinase transfers a phosphate group from 1,3-bisphosphoglycerate to ADP to form ATP and 3-phosphoglycerate. In this step, 1,3 bisphoglycerate is converted to 3-phosphoglycerate by the enzyme phosphoglycerate kinase (PGK). This reaction involves the loss of a phosphate group from the starting material. The phosphate is transferred to a molecule of ADP that yields our first molecule of ATP. Since we actually have two molecules of 1,3 bisphoglycerate (because there were two 3-carbon products from stage 1 of glycolysis), we actually synthesize two molecules of ATP at this step. 73 Step 8 – _________________________ Enzyme: Phosphoglycerate Mutase Reaction: 3-Phosphoglycerate → 2- Phosphoglycerate Purpose: _________________________ 74 Step 8: Phosphoglycerate Mutase The enzyme phosphoglycero mutase relocates the P from 3- phosphoglycerate from the 3rd carbon to the 2nd carbon to form 2-phosphoglycerate. This step involves a simple rearrangement of the position of the phosphate group on the 3 phosphoglycerate molecule, making it 2 phosphoglycerate. The molecule responsible for catalyzing this reaction is called phosphoglycerate mutase (PGM). A mutase is an enzyme that catalyzes the transfer of a functional group from one position on a molecule to another. The enzyme then removes the phosphate from the 3′ position leaving just the 2′ phosphate, and75 Step 9 – _________________________ Enzyme: ________________________ Reaction: 2-Phosphoglycerate → Phosphoenolpyruvate (PEP) + H₂O Purpose: _________________________ 76 Step 9: Enolase The enzyme enolase removes a molecule of water from 2-phosphoglycerate to form phosphoenolpyruvic acid (PEP). This step involves the conversion of 2 phosphoglycerate to phosphoenolpyruvate (PEP). The reaction is catalyzed by the enzyme enolase. Enolase works by removing a water group, or dehydrating the 2 phosphoglycerate. The specificity of the enzyme pocket allows for the reaction to occur through a series of steps too complicated to cover here. 77 Step 10 – ____________________________________ Enzyme: ________________ Reaction: Phosphoenolpyruvate (PEP) + ADP → Pyruvate + ATP Purpose: Produces __________________. Forms ___________, the final product of glycolysis. 78 Step 10: Pyruvate Kinase The enzyme pyruvate kinase transfers a P from phosphoenolpyruvate (PEP) to ADP to form pyruvic acid and ATP Result in step 10. The final step of glycolysis converts phosphoenolpyruvate into pyruvate with the help of the enzyme pyruvate kinase. As the enzyme’s name suggests, this reaction involves the transfer of a phosphate group. The phosphate group attached to the 2′ carbon of the PEP is transferred to a molecule of ADP, yielding ATP. Again, since there are two molecules of PEP, here we actually generate 2 ATP molecules. 79 Summary of Glycolysis Key Outputs per Glucose Molecule: ____________________ ____________________ ____________________ Pathways After Glycolysis: Aerobic (with oxygen): Pyruvate enters the mitochondria for the Krebs cycle & oxidative phosphorylation. Anaerobic (without oxygen): Pyruvate undergoes fermentation (lactic acid or ethanol production). 80 Glycolysis Steps 1 and 3 = ____________________ Steps 7 and 10 = ____________________ Net “visible” ATP produced = ____________________ 81 Glycolysis = Continue Respiration Immediately upon finishing glycolysis, the cell must continue respiration in either an aerobic or anaerobic direction; this choice is made based on the circumstances of the particular cell. A cell that can perform aerobic respiration and which finds itself in the presence of oxygen will continue on to the aerobic citric acid cycle in the mitochondria. If a cell able to perform aerobic respiration is in a situation where there is no oxygen (such as muscles under extreme exertion), it will move into a type of anaerobic respiration called homolactic fermentation. Some cells such as yeast are unable to carry out aerobic respiration and will automatically move into a type of anaerobic respiration called alcoholic fermentation. 82 Another Way To Look At It Stage One - Glycolysis For each molecule of glucose that passes through glycolysis, the cell nets two ATP molecules. Priming glucose priming cleavage and rearrangement Substrate-level phosphorylation oxidation ATP generation 83 84 Figure 2-70 Molecular Biology of the Cell (© Garland Science 2008) Priming Reactions Fructose 1,6- bisphosphate - 2 ATPs 85 Recycling NADH As long as food molecules are available to be converted into glucose, a cell can produce ATP. Continual production creates NADH accumulation and NAD+ depletion. NADH must be recycled into NAD+. aerobicrespiration fermentation 86 Recycling NADH 87 Krebs (Citric Acid) Cycle Also called the TriCarboxylic Acid (TCA) Cycle. Location: Prokaryotic cells – occurs in the cytoplasm. Eukaryotic cells – takes place in the mitochondrial matrix. Glycolysis converts glucose into pyruvate, which enters the cycle. Oxidizes glucose derivatives, fatty acids, and amino acids. Produces carbon dioxide (CO₂) through enzyme-controlled steps. The cycle collects high-energy electrons by oxidizing fuels.Produces 8 high-energy 88 electrons, carried by:NADH Krebs (Citric Acid) Cycle It is also known as TriCarboxylic Acid (TCA) cycle. In prokaryotic cells, the citric acid cycle occurs in the cytoplasm; in eukaryotic cells, the citric acid cycle takes place in the matrix of the mitochondria. In glycolysis, glucose is converted into pyruvate. The process oxidises glucose derivatives, fatty acids and amino acids to carbon dioxide (CO2) through a series of enzyme controlled steps. The purpose of the Krebs Cycle is to collect (eight) high-energy electrons from these fuels by oxidising them, which are transported by activated carriers NADH and FADH2 to the electron transport chain. The Krebs Cycle is also the source for the precursors of many other molecules, and is therefore an amphibolic pathway (meaning it is both anabolic and89 Krebs Cycle 90 Reaction 1: Formation of Citrate The first step of the Krebs Cycle. Acetyl-CoA combines with oxaloacetate to form citrate. Catalyzed by the enzyme citrate synthase. Acetyl-CoA donates a 2-carbon acetyl group to oxaloacetate. A water molecule helps catalyze the reaction. Coenzyme A (CoA) is released, forming citrate. This step initiates the Krebs Cycle and is essential for energy production. Citrate enters the cycle and undergoes further modifications. Prepares molecules for the production of NADH, FADH₂, and ATP. 91 Reaction 2: Formation of Isocitrate Citrate is rearranged to form its isomer, isocitrate. Enzyme involved: Aconitase. This transformation prepares the molecule for further oxidation in the Krebs Cycle. Water molecule is removed from citrate, causing structural rearrangement. Water is re-added at a different position, shifting the – OH group from the 3' to the 4' position, forming isocitrate. This step creates isocitrate, a key intermediate in the Krebs Cycle. Sets up the next reaction, where isocitrate is oxidized to produce NADH, a crucial step in energy production. 92 Reaction 3: Oxidation of Isocitrate to α-Ketoglutarate Isocitrate is oxidized to form α-ketoglutarate. Enzyme involved: Isocitrate dehydrogenase. This is an oxidative decarboxylation reaction. NAD⁺ is reduced to NADH + H⁺, storing high-energy electrons. A carbon dioxide (CO₂) molecule is released, making this the first decarboxylation step in the Krebs Cycle. This reaction prepares α-ketoglutarate for further oxidation and ATP production. Key role: Contributes electrons to the Electron Transport Chain, driving ATP synthesis. 93 Ketoglutarate to Succinyl- CoA α-Ketoglutarate is oxidized, resulting in the removal of carbon dioxide (CO₂). Coenzyme A (CoA) is added, forming the 4-carbon compound succinyl-CoA. Enzyme involved: α-Ketoglutarate dehydrogenase. NAD⁺ is reduced to NADH + H⁺, capturing high-energy electrons. This reaction is crucial for energy production and the continuation of the Krebs Cycle. Key role: Supplies electrons to the Electron Transport Chain, contributing to ATP synthesis. 94 Reaction 5: Conversion of Succinyl-CoA to Succinate Coenzyme A (CoA) is removed from succinyl-CoA, forming succinate. Enzyme involved: Succinyl-CoA synthase. The energy released is used to generate guanosine triphosphate (GTP) from guanosine diphosphate (GDP) and Pi via substrate-level phosphorylation. GTP can be converted into ATP, contributing to cellular energy production. This step is crucial for ATP synthesis within the Krebs Cycle. 95 Reaction 5: Conversion of Succinyl-CoA to Succinate Coenzyme A (CoA) is removed from succinyl-CoA, forming succinate. Enzyme involved: Succinyl-CoA synthase. The energy released drives the conversion of guanosine diphosphate (GDP) + Pi into guanosine triphosphate (GTP) via substrate-level phosphorylation. GTP can be used to generate ATP, supporting cellular energy production. This step is crucial for energy conservation in the Krebs Cycle. 96 Reaction 6: Oxidation of Succinate to Fumarate Succinate is oxidized to form fumarate. Enzyme involved: Succinate dehydrogenase. FAD (Flavin Adenine Dinucleotide) is reduced to FADH₂, capturing high-energy electrons. The enzyme catalyzes the removal of two hydrogen atoms from succinate. This reaction contributes electrons to the Electron Transport Chain, aiding ATP production. 97 Reaction 7: Hydration of Fumarate to Malate Fumarate is hydrated to form L-malate. Enzyme involved: Fumarase (Fumarate hydratase). This reaction is reversible and plays a key role in the Krebs Cycle. A water molecule (H₂O) is added, inserting hydrogen and oxygen back into the substrate. This step continues the rearrangement process, preparing the molecule for the final oxidation step. 98 Reaction 8: Oxidation of Malate to Oxaloacetate Malate is oxidized to form oxaloacetate, which restarts the Krebs Cycle. Enzyme involved: Malate dehydrogenase. NAD⁺ is reduced to NADH + H⁺, storing high-energy electrons for ATP production. This final step completes the Krebs Cycle, regenerating oxaloacetate for another turn. The generated NADH carries electrons to the Electron Transport Chain for further ATP synthesis. 99 ATP Generation in the Krebs Cycle The Krebs Cycle transforms the acetyl group and water into carbon dioxide and high-energy electron carriers. Total ATP Yield: 12 ATP (per acetyl-CoA). 3 NADH → Produces 9 ATP. 1 FADH₂ → Produces 2 ATP. 1 Direct ATP (or GTP) → Produces 1 ATP via substrate-level phosphorylation. Key Role in Cellular Respiration NADH and FADH₂ transfer electrons to the Electron Transport Chain (ETC). The ETC generates the majority of ATP through oxidative phosphorylation. 100 Significance of Krebs Cycle Biosynthesis of Biomolecules Produces intermediates for amino acids, nucleotides, chlorophyll, cytochromes, and fats. Formation of Chlorophyll Succinyl-CoA is involved in chlorophyll biosynthesis. Amino Acid Production α-Ketoglutarate, pyruvic acid, and oxaloacetate serve as precursors for amino acid synthesis. Energy Production Generates a significant amount of ATP, which is essential for cellular metabolism. 101 Krebs Cycle Acetyl-CoA is oxidized through a series of nine reactions. The process occurs in two main steps: Priming Acetyl-CoA combines with oxaloacetate to form citrate. Citrate undergoes structural modifications to prepare for energy extraction. Energy Extraction NADH and FADH₂ are produced through oxidation. ATP (or GTP) is generated via substrate- level phosphorylation. CO₂ is released, completing the cycle. 102 103 Krebs Cycle / Citric Acid Cycle - Summary of Steps 1.Condensation 1. Acetyl-CoA combines with oxaloacetate to form citrate. 2-3. Isomerization Citrate is rearranged to form isocitrate. 4.First Oxidation 4. Isocitrate is oxidized to α-ketoglutarate, producing NADH and releasing CO₂. 5.Second Oxidation 4. α-Ketoglutarate is oxidized to succinyl-CoA, generating another NADH and CO₂. 104 Krebs Cycle / Citric Acid Cycle - Summary of Steps 4.Substrate-Level Phosphorylation 4. Succinyl-CoA is converted to succinate, generating ATP (or GTP). 5.Third Oxidation 4. Succinate is oxidized to fumarate, producing FADH₂. 8-9. Regeneration of Oxaloacetate Fumarate is converted to malate, then malate is oxidized to oxaloacetate, generating NADH. 105 Key Steps in the Citric Acid Cycle Step 1 Condensation (Formation of Citrate) Acetyl-CoA combines with oxaloacetate to form citrate. Steps 2-3 Isomerization Citrate is rearranged to form isocitrate through cis-aconitate. Step 4 First Oxidation (Production of NADH & CO₂) Isocitrate is oxidized to α- ketoglutarate. NAD⁺ is reduced to NADH, and CO₂ is released. 106 Key Steps in the Citric Acid Cycle Step 6 Substrate-Level Phosphorylation (ATP/GTP Production) Succinyl-CoA is converted to succinate, generating GTP/ATP. Step 7 Third Oxidation (Production of FADH₂) (⭐) Succinate is oxidized to fumarate, reducing FAD to FADH₂. Step 8 Hydration (Addition of Water) Fumarate is converted to malate with the addition of H₂O. Step 9 Final Oxidation (Regeneration of Oxaloacetate) 107 Malate is oxidized to oxaloacetate, Figure 12.10 The citric acid cycle. 108 109 Figure 2-84 Molecular Biology of the Cell (© Garland Science 2008) Electron Transport Chain The final stage of aerobic respiration, located on the inner mitochondrial membrane. The inner membrane has folds (cristae) to increase surface area for efficient ATP production. The ETC releases stored energy from NADH and FADH₂ to drive ATP synthesis. This process is called oxidative phosphorylation, where ATP is produced using energy from electron transport and oxygen reduction. Key Role of the ETC Converts high-energy electrons from NADH & FADH₂ into ATP. Oxygen acts as the final electron acceptor, 110 forming water (H₂O). Electron Transport Chain Oxidative phosphorylation occurs over a number of distinct steps: Proton pumps create an electrochemical gradient (proton motive force) ATP synthase uses the subsequent diffusion of protons (chemiosmosis) to synthesise ATP Oxygen accepts electrons and protons to form water 111 Electron Transport Chain – Oxidative Phosphorylation Oxidative phosphorylation occurs in distinct steps: 1. Proton Pumping & Electrochemical Gradient Electron carriers NADH and FADH₂ transfer electrons to the ETC. Energy from electrons powers proton pumps, creating a proton motive force across the inner mitochondrial membrane. 2. ATP Synthesis via Chemiosmosis ATP synthase allows protons to diffuse back into the mitochondrial matrix. This diffusion powers ATP synthesis from ADP and Pi. 3. Oxygen as the Final Electron Acceptor O₂ accepts electrons and protons, forming water (H₂O) as a byproduct. 112 Step 1: Generating a Proton Motive Force 1.Oxidation of NADH & FADH₂ 1.NADH and FADH₂ are oxidized, releasing high- energy electrons and protons (H⁺). 2. Electrons are transferred to the Electron Transport Chain (ETC). 2.Electron Transfer & Proton Pumping 1. The ETC consists of transmembrane carrier proteins. 2. As electrons move through the chain, they lose energy. 3. This energy is used to pump protons (H⁺) from the mitochondrial matrix into the intermembrane space. 3.Formation of an Electrochemical Gradient 113 Step 1: Generating a Proton Motive Force 114 Synthesis via Chemiosmosis 1.Proton Motive Force Drives H⁺ Movement 1.H⁺ ions move down their electrochemical gradient from the intermembrane space back into the mitochondrial matrix. 2.Chemiosmosis Facilitates ATP Production 1. This process is known as chemiosmosis, where ATP synthase allows the controlled diffusion of H⁺ ions across the membrane. 3.ATP Synthase Generates ATP 1. As H⁺ ions pass through ATP synthase, the enzyme undergoes molecular rotation. 2. This movement catalyzes the conversion of ADP + Pi into ATP. 115 Synthesis via Chemiosmosis 116 Step Three: Reduction of Oxygen 1. Removal of De-Energized Electrons 1. The Electron Transport Chain (ETC) must clear out used electrons to continue functioning. 2. Without removal, the chain would become blocked, stopping ATP production. 2. Oxygen as the Final Electron Acceptor 3. Oxygen (O₂) removes de-energized electrons, preventing a buildup. 4. This allows the ETC to keep accepting new high-energy electrons from NADH and FADH₂. 117 Step Three: Reduction of Oxygen 3. Formation of Water & Proton Gradient Maintenance 1. Oxygen also binds with free protons (H⁺) in the matrix, forming water (H₂O). 2. This removal of protons helps maintain the hydrogen gradient, ensuring ATP synthesis can continue. 4. Importance of Oxygen in ATP Production 3. Without oxygen, the ETC stops functioning, as hydrogen carriers (NADH & FADH₂) cannot transfer electrons. 4. This results in a halt in ATP production, leading to anaerobic processes instead. 118 Step Three: Reduction of Oxygen 119 Summary: Oxidative Phosphorylation 1. Electron Transport & Energy Release NADH & FADH₂ donate high-energy electrons to the Electron Transport Chain (ETC), located in the mitochondrial cristae. As electrons move through the chain, they lose energy, which is transferred to electron carriers. 2. Proton Pumping & Gradient Formation Energy from electron transfer is used to pump H⁺ ions from the matrix into the intermembrane space. This creates an electrochemical gradient, also known as the proton motive force. 120 Summary: Oxidative Phosphorylation 3. ATP Synthesis via Chemiosmosis H⁺ ions diffuse back into the matrix through ATP synthase, generating ATP from ADP + Pi. 4. Oxygen as the Final Electron Acceptor Oxygen binds with electrons and H⁺ ions to form water (H₂O), preventing electron buildup and maintaining the chain’s function. 121 Stage Four: The Electron Transport Chain Electron Transfer NADH and FADH₂ donate high-energy electrons to the ETC located on the inner mitochondrial membrane. Electrons are passed through a series of membrane-associated proteins, releasing energy at each step. Proton Pumping The energy released is used to pump H⁺ ions (protons) from the matrix into the intermembrane space, creating an electrochemical gradient. This proton gradient is crucial for ATP production in the next stage (chemiosmosis). Facilitates ATP synthesis by creating the proton 122 Harvesting Energy by Extracting Electrons Glucose Catabolism & Energy Release 1. The breakdown of glucose occurs through oxidation-reduction (redox) reactions. 2. These reactions gradually transfer electrons closer to oxygen, releasing energy in the process. Role of NAD⁺ as an Electron Carrier 3.NAD⁺ (Nicotinamide Adenine Dinucleotide) collects high-energy electrons from glucose. 4. It transports electrons to the Electron Transport Chain (ETC), where ATP is ultimately generated. 123 Harvesting Energy by Extracting Electrons Electrons are harvested step-by-step for controlled energy release. NAD⁺ plays a crucial role in transferring electrons efficiently. Sets up the next stages of cellular respiration (Krebs Cycle & ETC). 124 125 Figure 2-86 Molecular Biology of the Cell (© Garland Science 2008) 126 Figure 2-85 Molecular Biology of the Cell (© Garland Science 2008) Electron Transport Chain 127 Chemiosmosis 128 Figure 12.26 Structure of ATP synthase (the F0F1 complex) in the bacterial plasma membrane and mechanism of proton translocation across the membrane. 129 Experimental Figure 12.23 Synthesis of ATP by ATP synthase depends on a pH gradient across the membrane. 130 Mitochondria 131 Theoretical ATP Yield of Aerobic Respiration 132 Regulating Aerobic Respiration Glucose catabolism is tightly regulated at key steps to ensure energy efficiency. If a reaction has a highly negative ΔG, it is likely a regulated step. 133 Regulating Aerobic Respiration Hexokinase Converts glucose → glucose-6-phosphate to maintain low glucose levels in cells. Facilitates passive glucose transport into cells. Phosphofructokinase (PFK) Controls the committed step of glycolysis. Regulation: Upregulated by fructose-6-phosphate and AMP (indicates low energy, needing more ATP). Downregulated by ATP & glucagon (signals high energy state). Pyruvate Kinase Converts PEP → pyruvate, driving ATP production. Regulation: 1.Upregulated by PEP & fructose-6-phosphate. 134 Regulating Aerobic Respiration Regulation prevents unnecessary ATP production & conserves energy. ATP levels control glycolysis through feedback inhibition These regulatory steps ensure glucose is metabolized efficiently. 135 Regulating Aerobic Respiration Key Inhibitors in Aerobic Respiration: Acetyl-CoA Inhibits Pyruvate Dehydrogenase When Acetyl-CoA levels are high, pyruvate dehydrogenase is inhibited, preventing excessive conversion of pyruvate into Acetyl-CoA. This helps conserve resources and directs pyruvate toward alternative pathways when energy demand is low. NADH Inhibits Multiple Steps in the Citric Acid Cycle High NADH levels signal sufficient energy and inhibit key enzymes in the Citric Acid Cycle, slowing down ATP production. 136 This feedback regulation prevents excessive Regulating Aerobic Respiration Negative feedback loops regulate energy production. When ATP or NADH is high, respiration slows down to conserve energy. When energy is low, respiration is upregulated to meet demand. 137 Control of Glucose Catabolism 138 Catabolism of Proteins and Fats When glucose is scarce, proteins and fats serve as alternative energy sources. Protein Catabolism Proteins are broken down into amino acids. Deamination removes the amino group (NH₂), producing ammonia (NH₃) as a byproduct. The remaining carbon skeleton is metabolized through the Krebs Cycle or converted into glucose (gluconeogenesis). Fat Catabolism (Beta-Oxidation) Lipids (fats) are broken down into glycerol and fatty acids. 139 Glycerol can enter glycolysis. Catabolism of Proteins and Fats Fat Catabolism (Beta-Oxidation) Lipids (fats) are broken down into glycerol and fatty acids. Glycerol can enter glycolysis. Fatty acids undergo beta-oxidation, producing Acetyl-CoA, which enters the Krebs Cycle for ATP generation. More ATP is produced from fats than carbohydrates, making them an efficient energy source. 140 Getting energy from Carbohydrates and Fats 141 Figure 2-80 Molecular Biology of the Cell (© Garland Science 2008) 142 Figure 2-78 Molecular Biology of the Cell (© Garland Science 2008) 143 Figure 2-81b Molecular Biology of the Cell (© Garland Science 2008) Cellular Extraction of Chemical Energy 144 Evolution of Cellular Respiration degradation glycolysis anaerobic photosynthesis oxygen-forming photosynthesis nitrogen fixation aerobic respiration 145 Evolution of Cellular Respiration Evolution of Cellular Respiration Cellular respiration evolved gradually as life adapted to Earth's changing environment. Key Evolutionary Steps: Degradation (Primitive Metabolism) Early life forms broke down organic molecules for energy without oxygen. Glycolysis (~3.5 billion years ago) First major metabolic pathway. Anaerobic process that breaks glucose into ATP and pyruvate. Still used by all living organisms today. 146 Evolution of Cellular Respiration Anaerobic Photosynthesis (~3.2 billion years ago) Some bacteria developed the ability to use light energy to fix carbon without producing oxygen. Oxygen-Forming Photosynthesis (~2.7 billion years ago) Cyanobacteria evolved, producing oxygen as a byproduct of photosynthesis. Oxygen accumulated in the atmosphere (Great Oxygenation Event). Nitrogen Fixation (~2.5 billion years ago) Some organisms evolved enzymes to convert atmospheric nitrogen into usable forms. Aerobic Respiration (~2 billion years ago) 147 Evolution of Cellular Respiration Life started with simple energy extraction methods. The evolution of oxygen-producing photosynthesis changed Earth's atmosphere. Aerobic respiration made energy production much more efficient, supporting complex life. 148 Anabolic Pathways Cells build molecules from a subset of precursors Many come from Glycolysis Glucose-6-phosphate, Fructose-6-Phosphate, dyhydroxyacetone phosphate, 3-phosphoglycerate, phosphoenolpyruvate, pyruvate Some come from the Citric Acid Cycle Citrate, a-ketogluterate, succinyl CoA, Oxaloacetate The anabolic use of these intermediates allows cells to grow, repair, and store energy. 149 Representation of the anabolic pathways, showing how key precursor molecules are derived from Glycolysis and the Citric Acid Cycle. 150 Photosynthesis Primary Energy Source: Most living cells derive energy from the sun. Energy Capture: Plants, algae, and bacteria capture sunlight through photosynthesis. Focus: This discussion will emphasize photosynthesis in multicellular eukaryotic plants. 151 Leaf Structure The cuticle is transparent. Chloroplasts in the mesophyll cells are what makes leaves look green The stomata can open to let CO2 in and O2 out. 152 153 Figure 1-35 Molecular Biology of the Cell, Fifth Edition (© Garland Science 2008) Chloroplasts Thylakoid Membranes: Internal membranes in chloroplasts, organized into grana. Function: Thylakoid membranes contain pigments for capturing light and producing ATP. Photosystems: Clusters of pigments that act as antennas to collect light energy efficiently. 154 Chloroplasts 155 Two major processes in Photosynthesis Light-Dependent Reactions Occur in the thylakoids of the chloroplast. Capture sunlight using pigments. Convert sunlight into ATP and NADPH for energy. Light-Independent Reactions (Calvin Cycle) Occur in the stroma of the chloroplast. Use CO₂ to build organic molecules. Utilize ATP and NADPH from the light reactions. 156 Energy in Photons The energy of a photon is inversely proportional to its wavelength. Gamma rays have the highest intensity and shortest wavelength. Ultraviolet (UV) light has more energy than visible light and can disrupt DNA. 157 Electromagnetic Spectrum 158 Absorption Spectra Photon absorption depends on wavelength and molecular composition. Each molecule has a unique absorption spectrum, which defines: The range of photons it can absorb. The efficiency of absorption at different wavelengths. 159 Pigments Pigments are molecules that absorb light in the visible range. Green plant photosynthesis uses two main types of pigments: Carotenoids Chlorophyll Chlorophyll a – main pigment Chlorophyll b – accessory pigment 160 Chlorophyll Chlorophylls absorb photons through an excitation process. Photon absorption excites electrons in the pigment’s ring structure. Excited electrons are channeled away through an alternating carbon-bond system. Wavelengths absorbed depend on the energy levels available for electron excitation. 161 Figure 12.33 Structure of chlorophyll a, the principal pigment that traps light energy. Pigments typically have ring structures that capture specific wavelengths of light 162 Absorption Spectra 163 Light and Reducing Power Light-dependent reactions of photosynthesis use light energy to: Reduce NADP to NADPH Synthesize ATP Reducing power from water splitting helps convert CO₂ into organic molecules during carbon fixation. 164 Figure 12.35 A 165 166 166 The cytochrome complexes of mitochondria and chloroplasts have evolutionarily related proteins in common Mitochondria and chloroplasts share evolutionarily related proteins in their cytochrome complexes. Descent with modification is a recurring theme in evolution. Homologous genes exist due to a common ancestor. Comparing the electron transport chains of mitochondria and chloroplasts reveals homologous genes. 167 CO2 incorporation Summary: CO₂ Incorporation (Calvin- Benson Cycle) The Calvin-Benson cycle is responsible for incorporating CO₂ into organic molecules. This process requires a massive energy input. For every 6 CO₂ molecules incorporated: 18 ATP and 12 NADPH are consumed. Glucose is not directly produced; instead, precursor molecules are formed. 168 168 The Calvin cycle was determined by isotope labeling methods ¹⁴C-labeled CO₂ was introduced into green algae cultures. Incubation periods varied to track carbon assimilation over time. Two-dimensional paper chromatography was used to separate radiolabeled molecules. Autoradiography helped visualize ¹⁴C-labeled compounds as dark spots on film. Scientists identified the sequence in which labeled molecules appeared. Melvin Calvin was awarded the Nobel Prize in 1961 for this discovery. 169 Figure 12.47 CO2 fixation and photorespiration. 170 Calvin Know these steps what goes in and Cycle out, the three parts, names, Rubisco 171 171 Light-Independent Reactions 172 Figure 12.46 The pathway of carbon during photosynthesis. 173 Figure 12.48 Leaf anatomy of C4 plants and the C4 pathway. C4 photosynthesi s Faster, less efficient 174 Summary Cellular Energy Harvest Cellular Respiration Glycolysis Fermentation Oxidation of Pyruvate Krebs Cycle Electron Transport Chain Evolution of Metabolism Photosynthesis Energy harvesting Calvin Cycle 175