Cell Biology Part 2 July 2023 PDF
Document Details
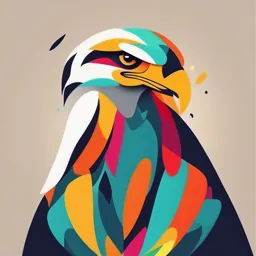
Uploaded by MemorableMoldavite5605
2023
Andrey Nikitin and Brandon Kim
Tags
Summary
This document is a handout on cell biology, specifically covering cellular respiration and photosynthesis. It includes detailed explanations of key concepts, such as important molecules, energy production, and various processes. The handout emphasizes understanding of the steps and processes involved.
Full Transcript
Cell Biology Part 2 Andrey Nikitin and Brandon Kim July 2023 Table of Contents 1 Introduction...
Cell Biology Part 2 Andrey Nikitin and Brandon Kim July 2023 Table of Contents 1 Introduction 3 1.1 Important Molecules................................... 3 1.2 Tips + Tricks for Understanding Reactions....................... 4 1.2.1 Naming...................................... 4 1.2.2 Important Enzymes................................ 4 2 Cellular Respiration 5 2.1 Glycolysis......................................... 5 2.1.1 Energy Investment................................ 6 2.1.2 Energy payoff................................... 7 2.1.3 Result....................................... 7 2.2 Intermediate Step..................................... 7 2.3 Krebs Cycle........................................ 8 2.3.1 Result....................................... 10 2.4 Electron Transport Chain (ETC)............................ 10 2.4.1 ATP synthase................................... 11 2.4.2 The Chain..................................... 13 2.4.3 When things go wrong.............................. 14 2.4.4 Result....................................... 15 2.5 Fermentation....................................... 16 2.5.1 Alcohol Fermentation............................... 16 2.5.2 Lactic Acid Fermentation............................ 17 2.5.3 Anaerobic Respiration.............................. 17 2.6 Gluconeogenesis...................................... 18 3 Photosynthesis 19 3.1 Photosystems....................................... 19 3.2 Light Reactions...................................... 21 3.2.1 Linear Electron Flow............................... 21 3.2.2 Cyclic Electron Flow............................... 22 3.3 Calvin Cycle/Light-Independent Reactions/Dark Reactions.............. 23 3.4 Photorespiration..................................... 25 3.4.1 C4......................................... 26 1 USABO Guide Andrey Nikitin 3.4.2 CAM........................................ 28 4 Conclusion 28 2 USABO Guide Andrey Nikitin 1 Introduction Cells are always performing activities—moving, eating, communicating, transporting solutes, etc. When performing these activities, cells require energy. To obtain energy, cells must harvest us- able energy from their available surroundings effectively. The process of cellular respiration and photosynthesis facilitate the process of obtaining and storing energy to keep a cell alive. For this handout, obscure info will be in gray. 1.1 Important Molecules Since the goal of cellular respiration and photosynthesis is to produce energy, we should pay careful attention to any energy-rich molecules. These molecules are color-coded throughout the notes to help you notice when they come into play: ATP (adenosine triphosphate) is the cell’s main unit of energy. Its ability to be quickly broken down and formed allows it to be used on demand wherever it is needed in the cell. Due to its convenience, the goal of cellular respiration is going to be to produce as much ATP as possible. ATP is made up of a nitrogenous base (adenine), the sugar ribose, and 3 phosphates linked together. To utilize the energy from ATP, the bonds between phosphates are broken, con- verting ATP into ADP (adenosine diphosphate) and Pi (inorganic phosphate). This can be broken down even furter to form AMP (adenosine monophosphate) and Pi. A single phosphate on its own is called orthophosphate, while two phosphates together is called pyrophosphate. – When ATP is made as a result of a reaction catalyzed by an enzyme, it is known as substrate-level phosphorylation. – When ATP is made as a result of the ETC (which uses O2 ), it is known as oxidative phosphorylation. – When ATP is made during photosynthesis, it is known as photophosphorylation. NAD+ (Nicotinamide adenine dinucleotide) is what is known as an electron carrier. This means NAD+ stores energy in the form of electrons. It will accept H− (a hydrogen with an extra electron) from molecules, turning it into NADH. The NADH will then release its electrons at the ETC (explained later) to convert that energy into ATP. Each NADH can be cashed in at the ETC for 3 ATP, although if you account for the energy it takes to transport pyruvate into the mitochondria it’s more like 2.5ATP or even 2ATP depending on who you ask. When NAD+ turns into NADH, we say it is reduced, since it accepts electrons. NADH is the reduced form, NAD+ is the oxidized form. Vitamin B3 (niacin) is important for the production of NAD+. FAD (Flavin adenine dinucleotide) is very similar to NAD+ except it accepts 2H to become FADH2. Like NADH, FADH2 is used in the ETC to generate ATP. Each FADH2 can be cashed in at the ETC for 2 ATP, although some sources will say it’s more like 1.5ATP. Vitamin B2 (riboflavin) is important for the production of FAD. 3 USABO Guide Andrey Nikitin NADP+ is very important in photosynthesis. It serves the same function as NAD+ , except its energy is used to generate glucose in the Calvin cycle. 1.2 Tips + Tricks for Understanding Reactions Both cellular respiration and photosynthesis contain many steps in their chemical reactions, all of which are helpful to know. As a result, it can be daunting to try and tackle them all, especially for those with no background in chemistry. Here are some general rules that can help make the process easier. 1.2.1 Naming If you’ve dabbled in organic chemistry, then you already know how to name compounds. However, anyone can understand compound names by following these two general rules: 1. If you add something to a compound, then you just add it to its name! For example, hexokinase adds a phosphate onto glucose, turning it into glucose-6-phosphate. See that second part of the name simply shows where we added the phosphate. 2. Enzymes are often named by their substrate. For example, phosphofructokinase adds a phosphate to fructose-6-phosphate. Keep in mind that many reactions catalyzed by enzymes are reversible. This means that sometimes we’ll see reactions happen in reverse. For example, the enzyme pyruvatekinase takes a phosphate from PEP to make pyruvate. If we consider the reverse reaction (adding a phosphate to pyruvate), then the name of the enzyme makes a lot more sense. 1.2.2 Important Enzymes There are a few major types of enzymes you need to know. By being able to identify the key words, it will be a lot easier to understand which steps produce which products: Kinase: An enzyme that adds a phosphate. Therefore, whenever you see a kinase being used, ATP will be either used or produced. Dehydrogenase: As the name suggests, this enzyme takes away a hydrogen (de-hydrogen) from its substrate. This hydrogen is then given to an electron carrier. Therefore, whenever you see a dehydrogenase being used, NADH or FADH2 will be produced. Isomerase: As the name suggests, this enzyme converts a molecule to its isomer. For exam- ple, phosphoglucoisomerase converts glucose-6-phosphate to its isomer fructose-6-phosphate. 4 USABO Guide Andrey Nikitin 2 Cellular Respiration The collection of all chemical reactions that occur within an organism is known as that organism’s metabolism. Many of these reactions require energy in the form of ATP. Thus, cellular respi- ration, or the harvesting of ATP from glucose, is an important process. Cellular respiration can be summarized in the following reaction: C6 H12 O6 + 6O2 → 6CO2 + 6H2 O + energy Here, glucose (C6 H12 O6 ) is broken down using oxygen (O2 ), producing carbon dioxide (CO2 ) and water (H2 O) as by-products. This process occurs over several distinct steps, with each step having their own pathways to be memorized: 1. Glycolysis: glucose is broken into 2 pyruvate, yielding a net of 2 ATP and 2 NADH in the process. Since 2 pyruvate are produced, each subsequent step happens twice. 2. Intermediate step: pyruvate is converted to acetyl CoA, producing 1 CO2 and 1 NADH. 3. Krebs Cycle: acetyl CoA is put into a cycle of reactions to farm as much NADH from it as possible. Each acetyl CoA makes 1 ATP, 3 NADH and 1 FADH2. 4. Electron Transport Chain (ETC): Now we cash in all the NADH and FADH2 we made. Each NADH is worth 3 ATP, so 3(2 + 2(1 + 3)) = 3(10) = 30 ATP. Each FADH2 is worth 2 ATP, so 2(2(1)) = 2(2) = 4 ATP. Putting this all together, we have 30 ATP from NADH, 4 ATP from FADH2 , 2 ATP from glycolysis, and 2(1) = 2 ATP from the Krebs cycle. 30 + 4 + 2 + 2 = 38 ATP! Note that if we plug in our adjusted values, 10(2.5) + 2(1.5) + 2 + 2 = 32 ATP, or 10(2) + 2(2) + 2 + 2 = 30 ATP. Thus, even though the cell could theoretically generate 38 ATP per glucose, realistically it is more like 30-32 ATP. In other words, cellular respiration is an incredibly efficient method of extracting energy from glucose. As we will discuss later, sometimes fermentation is used as an alternative pathway when O2 is not available. For each step, we will go through the pathway together. It’s a lot of reactions to remember, so I will put a * next to the most important steps. In addition, I will put a * next to the steps that are irreversible, this will become important when we talk about gluconeogenesis. 2.1 Glycolysis Glycolysis occurs in the cytoplasm of all cells! Refer to the diagram below as we go through all of its steps: 5 USABO Guide Andrey Nikitin Figure 1: Steps of glycolysis. (Source: Pediaa.com) 2.1.1 Energy Investment Glycolysis is split into two phases, energy investment (top) and energy payoff (bottom). The energy investment phase uses ATP to convert glucose to 2 G3P (glyceraldehyde 3-phosphate). In the energy payoff phase, G3P will be broken down to make ATP. 1. ** Hexokinase uses 1 ATP to add a phosphate to glucose, converting it to glucose-6- phosphate. Glucose is brought into the cell using GLUT transporters. Recall from Cell Bio pt 1, GLUT is an example of facilitated diffusion. Free glucose flows from high concentrations outside the cell to low concentrations inside the cell. However, in order to keep a steady flow of glucose and to prevent any glucose from leaving the cell, the cell needs to do something to the glucose coming in. This is why hexokinase is so important: it traps glucose inside the cell by adding a charged phosphate to it. This keeps free glucose concentrations inside the cell low, maintaining the concentration gradient. 2. Phosphoglucoisomerase converts glucose-6-phosphate to fructose-6-phosphate. 3. ** Phosphofructokinase (PFK-1) uses 1 ATP to add a phosphate to fructose-6-phosphate, converting it to fructose-1,6-bisphosphate. Note that “bis” is used in the name instead of “bi” because the phosphates are not adjacent. This step is known as the rate-limiting step and serves as the main control point for cellular respiration. Compounds that indicate that the cell already has a lot of energy, such as ATP and citrate, inhibit PFK-1 while compounds that indicate that the cell needs more energy, such as ADP, activate PFK-1. 6 USABO Guide Andrey Nikitin 4. Fructose bisphosphate adolase chops the 6-carbon fructose-1,6-bisphosphate into two 3-carbon molecules: DHAP and G3P. The top half becomes DHAP (dihydroxyacetone phosphate). DHAP is useful in regu- lating glycolysis, as it can be used to make triglycerides, but it is often ignored. The bottom half becomes G3P (glyceraldehyde 3-phosphate). In the energy payoff phase, we will break down G3P to get ATP from it. Triose phosphate isomerase can convert between DHAP and G3P. Most of the time, it turns DHAP into another G3P. This means that we now have 2 G3P. Therefore, all steps that follow will occur twice. 2.1.2 Energy payoff 1. Glyceraldehyde 3-phosphate dehydrogenase replaces a H− with a phosphate, resulting in 1,3-bisphosphoglycerate. The H− reduces a NAD+ to NADH! 2. Phosphoglyceratekinase takes a phosphate (making 1 ATP!) from 1,3-bisphosphoglycerate, turning it into 3-phosphoglycerate. 3. Phosphoglycerate isomerase moves the phosphate on 3-phosphoglycerate, turning it into 2-phosphoglycerate. 4. Enolase takes 1 H2 O from 2-phosphoglycerate, turning it into phosphoenolpyruvate, better known as PEP. 5. Pyruvate kinase takes a phosphate (making 1 ATP!) from PEP, resulting in pyruvate. 2.1.3 Result As we have seen, glycolysis converted glucose into 2 pyruvate. A net of 2 ATP was made since 4 (2 × steps 2,5 of energy payoff) - 2 (steps 1, 3 of energy investment) = 2. Furthermore, 2 NADH was made (2 × step 1 of energy payoff). 2.2 Intermediate Step Glycolysis occurred in the cytoplasm of all cells. However, the steps after glycolysis focus on extracting ATP from NADH, which is only possible when O2 is present. All of these steps occur in the matrix of the mitochondria, and thus are only found in eukaryotes. The intermediate step takes pyruvate from the cytoplasm and brings it into the mitochondria, converting it into acetyl CoA in preparation for the Krebs Cycle. 7 USABO Guide Andrey Nikitin Figure 2: The intermediate step. (Source: SlidePlayer) *Pyruvate dehydrogenase is actually a complex of multiple enzymes, allowing it to accomplish three things at once: 1. removs a CO2 from the top of pyruvate (this is the first time CO2 is produced!) 2. produces 1 NADH 3. adds a Coenzyme A to pyruvate, converting it to Acetyl CoA Vitamin B5 (pantothenic acid) is an important component of Coenzyme A. 2.3 Krebs Cycle Now, the cell takes the acetyl CoA it made and milks as much NADH as it can from it. This is known as the Krebs Cycle, or Citric Acid Cycle, or TCA cycle (Tricarboxylic acid cycle)... yeah, it has a lot of names. The important thing is that it is a cycle, which means it will start and end with the 4-carbon OAA (Oxaloacetate). The naming of the intermediates is not as intuitive as it was for glycolysis, but there are plenty of mnemonics out there to help you remember (my [AN] personal favorite is “susy baka is not Obama’s friend ”... trust me... it works, like really well). 8 USABO Guide Andrey Nikitin Figure 3: The Krebs Cycle. (Source: Wikipedia) 1. Citrate synthase makes 6-carbon citrate by adding 4-carbon OAA to 2-carbon acetyl CoA. 2. Aconitase converts citrate into D-isocitrate, using cis-Aconitase as an intermediate. 3. Isocitrate dehydrogenase converts D-isocitrate to α-ketoglutarate. Since it is a dehy- drogenase, 1 NADH is produced in the process. Notice that 1 CO2 is removed. This means that α-ketoglutarate is a 5-carbon molecule. 4. α-ketoglutarate dehydrogenase converts α-ketoglutarate to Succinyl-CoA. Since it is a dehydrogenase, 1 NADH is produced in the process. Notice that 1 CO2 is removed. This means that Succinyl-CoA is a 4-carbon molecule. 5. Succinyl-CoA synthetase removes a CoA, from Succinyl-CoA synthetase, turning it into Succinate. This also produces 1 GTP in the process, which is quickly converted into 1 ATP! 6. Succinic dehydrogenase removes 2H from succinate producing FADH2 (FAD is Q in the diagram) and Fumarate. 7. Fumarase turns fumarate into Malate. 8. Malic dehydrogenase turns malate into OAA, producing 1 NADH in the process. And with that final step, we are back to where we began, completing the cycle. 9 USABO Guide Andrey Nikitin 2.3.1 Result Just to make it clear, let’s review what was made during the Krebs cycle. 3 NADH was produced (steps 3,4,8) and since we have 2 acetyl CoA that means we produced 3×2 = 6 NADH. Combining that with the 1×2 = 2 NADH produced during the intermediate step and the 1×2 = 2 NADH produced during glycolysis brings us to a total of 6 + 2 + 2 = 10 NADH! 1 FADH2 was produced (step 6) and since we have 2 acetyl CoA that means we produced 1×2 = 2 FADH2. Since this is the only step in cellular respiration where FADH2 is produced, that means our total is 2 FADH2 ! 1 ATP was indirectly produced (step 5) and since we have 2 acetyl CoA that means we produced 1×2 = 2 ATP. If we include the net 2 ATP from glycolysis, that brings us to a total of 4 ATP! 2 CO2 was produced (steps 3,4) and since we have 2 acetyl CoA that means we produced 2×2 = 4 CO2. Combining this with the 1×2 = 2 CO2 released during the intermediate step, that brings us to a total of 6 CO2 ! This is where the 6 CO2 in the equation for cellular respiration comes from. 2.4 Electron Transport Chain (ETC) As the name suggests, the Electron Transport Chain is a chain of proteins that transport electrons from one to the next like a game of hot potato. Each of these proteins has their own electronegativity, or how strongly they want an electron. As an electron moves down the chain, it is held by proteins with stronger and stronger electronegativity until in the end, the most electronegative is oxygen, the final electron acceptor. 10 USABO Guide Andrey Nikitin Figure 4: In this three-step figure, an analogy for the electron transport chain is depicted. A protein “drops” an electron through a proton pump, decreasing the electron’s energy level and using that released energy to pump a proton into the intermembrane space. The next protein down the chain does this as well, and the process repeats until the electron reaches the end of the chain. It is important to note that in this figure, height represents potential energy. (Source: Andrew Ung) Each time an electron is exchanged, there is a change in energy due to the difference in elec- tronegativity. The ETC uses this energy to pump protons (H+ ) from the matrix to the intermem- brane space. This accumulation of H+ in the intermembrane space results in an electrochemical gradient (both the positive charges and the high concentration will cause protons to diffuse from the intermembrane space to the matrix). Therefore, if there was an open channel in the inner membrane, then protons would freely move across that membrane down their concentration. This movement is called chemiosmosis. The cell utilizes this chemiosmosis by using a very special channel: ATP synthase. The movement of H+ creates a proton-motive force, which causes ATP synthase to rotate, making ATP from ADP and Pi. 2.4.1 ATP synthase ATP synthase (sometimes called Complex V) is made of multiple subunits. One of them is F0 (subunit c), the part that is in the inner membrane and acts a rotor (along with γ and ϵ subunits). The other part is in the matrix and is called F1. This is the part that actually makes ATP (specifically subunits α and β). In addition, this part is also called the stator because it is stationary and holds the rotor in place. 11 USABO Guide Andrey Nikitin Figure 5: (left) diagram showing components of ATP synthase (Source: Research Gate), (right) diagram showing how ATP synthase uses the proton-motive force to generate ATP (Source: Campbell’s 9th Edition) Example 2.1 (USABO Opens 2018) Solution: This question is asking about what occurs during the ETC (oxidative phosphorylation). Remember, a molecule is oxidized when it loses electrons, and a molecule is reduced when it gains electrons (you can remember this as OIL RIG → oxidation is losing, reduction is gaining). In the ETC, NADH and FADH2 lose their electrons, which is passed along the chain until it is eventually gained by oxygen. Therefore, the answer is B. 12 USABO Guide Andrey Nikitin 2.4.2 The Chain Now that we understand how the ETC works, let’s look at its components (I shortened Complex to “Com”, but this is not standard notation): Com I (ubiquinone oxidoreductase): the largest, most complex, and most important com- plex in the ETC. Accepts electrons from NADH. Com II (succinate dehydrogenase): accepts elec- trons from FADH2. This is the only Com- plex that does NOT pump protons. Q (Ubiquinol): this is one of the mo- bile electron acceptors in the ETC. As a lipid, Q can move freely within the inner membrane, shuttling electrons from Complex I and II to Complex III. Com III (ubiquinol-cytochrome c oxidoreductase / cytochrome bc1 complex) Cytochrome c: this is the other mobile electron acceptor in the ETC. This one is a water-soluble protein and moves around the intermembrane space, shuttling electrons from Com- plex III to Complex IV Com IV (cytochrome c oxidase): the electrons from Complex IV are accepted by oxygen, result- Figure 6: This diagram orders the ing in the formation of water. components of the ETC by free energy. (Source: Campbell’s 9th Edition) Example 2.2 (USABO Opens 2018) 13 USABO Guide Andrey Nikitin Solution: These questions require a deep understanding of the components of the ETC. Question 7 is False (B) since ubiquinone (the oxidized form of ubiquinol) is a lipid, not a protein. Therefore, it will not have a heme group. Question 8 is False (B) since FADH2 gives its electrons to complex II. Question 9 and 10 are tricky, as you would have had to pay attention to the components of each complex in Figure 6. Cytochrome b is a part of complex III and Cytochrome a is a part of complex IV. We know that the ETC goes complex III → cytochrome c → complex IV. In addition, we know that the parts later in the ETC are more electronegative, with oxygen being the most electronegative. Therefore, 9 and 10 are both True (A). 2.4.3 When things go wrong Many questions like to test your understanding of the ETC by asking you what would happen if something didn’t go as expected. Let’s run through a few scenarios: No O2 : This can happen during strenuous exercise, as your muscle cells use up all the O2 they have access to. Since O2 is the final electron acceptor, without it, the electrons in the ETC have no where to go to, halting the chain. Thus, the cell will stop at glycolysis and not proceed any further, since the Krebs cycle generates NADH that is now useless. In the next subsection, we will talk more about the alternative pathways taken in such an event. This can also happen as a result of drugs, such as cyanide, that inhibit components of the ETC. A channel for H+ forms: Some drugs, such as dinitrophenol (DNP), create a new channel in the inner membrane for H+ to pass through. As a result, H+ will flow out of the intermem- brane space without powering ATP synthase. This increases the pH of the intermembrane space (since there is less H+ it is less acidic) and the subsequent loss of the electrochemical gradient drastically decreases the rate of ATP production. Since the cell is not produc- ing ATP when it should, the mitochondria may freak out and respond by increasing heat production. ATP synthase is blocked: In this case, the ETC functions as normal, pumping H+ to the intermembrane space. However, with ATP synthase blocked, those H+ have no where to go to. As a result, the pH of the intermembrane space decreases (since more H+ makes it more acidic). If the effects are really bad, the H+ can even leak out of the outer membrane, decreasing the pH of the cytoplasm. 14 USABO Guide Andrey Nikitin Example 2.3 (USABO Semifinals 2018) Solution: This question is testing whether we understand how proton concentration (and therefore acidity) changes as a result of cellular respiration, specifically the ETC. When we add glucose, the cell performs cellular respiration and the ETC will result in a mild increase in acidity. When we add oligomycin, however, the protons will accumulate in the intermembrane space since they can’t pass through ATP synthase. As a result, they will begin leaking out of the mitochondria, increasing the outside acidity. On the other hand, if we inhibit hexokinase (the first step in glycolysis), we inhibit all of cellular respiration. Thus we would expect a rapid drop in acidity as the ETC is no longer functional. From this, we can see that our answer is A. 2.4.4 Result The ETC produces a lot of ATP, and to see exactly how much you can go back to the beginning of section 2. This step is also the only point in which O2 is used. To understand how much, remember that each electron carrier is carrying 2 electrons (H− for NADH and H2 for FADH2 ), and we have a total of 12 electron carriers that are being fed into the ETC (10 NADH and 2 FADH2 ). Each Oxygen atom has 6 valence electrons and can therefore accept 2 more. So, if we have 12 electron carriers, each holding 2 electrons, then we would need 12 Oxygen atoms, each accepting 2 electrons. 12 Oxygen atoms means 6 O2 , just like we have in our equation! When O2 is used in the ETC, H2 O is produced in the process. 6 O2 → 12 H2 O, which means that cellular respiration produces a net of... wait, that doesn’t make any sense. We’re supposed to have a net of 6 H2 O, yet the ETC produces 12 H2 O. So what’s going on? If you were paying careful attention, you may have noticed that water is also involved in some steps of glycolysis and krebs cycle. If you look at the number of Oxygen and Hydrogen in the intermediates of the Krebs cycle, then you’ll see that H2 O is used (steps 1,2,5,7) and produced (step 2), giving us a net of 2 × (1 − 4) = -6 H2 O. Meanwhile, glycolysis produces (step 4 of energy payoff) 1 × 2 = 2 H2 O. Therefore, our total is now 12 − 6 + 2 = 8... which is still not 6. Maybe step 1 of energy payoff 15 USABO Guide Andrey Nikitin also uses H2 O??? Honestly, I have no clue, which conveys an important message: the cell is full of water so don’t start counting where water is used in reactions as that’ll just drive you crazy. All you need to worry about is that the ETC produces water from O2. 2.5 Fermentation Organisms can be classified based on whether or not they use oxygen. Aerobes use O2 and will die if they lack it. Obligate Anaerobes can’t use O2 and will die if they are exposed to it. Meanwhile, Facultative Anaerobes get the best of both worlds, using O2 when it’s available and switching to other means when it’s not. If some organisms don’t use O2 , then what do they do after glycolysis? The point of all subsequent steps in cellular respiration were to generate a bunch of NADH that can be then used in the ETC to make ATP. However, if they lack oxygen, then they can’t use the ETC. So do they just stop at glycolysis? NO! Another important role of the ETC that you probably ignored is that it turns NADH back into NAD+. By regenerating NAD+ , we can continue to perform glycolysis, which needed NAD+ to make NADH in the step involving G3P dehydrogenase. Therefore, if the ETC is not an option, anaerobes need to find some other way to regenerate NAD+. This is where fermentation comes in handy! 2.5.1 Alcohol Fermentation Alcohol Fermentation converts the pyruvate from glycolysis into ethanol in 2 steps: 1. PDC (pyruvate decarboxylase) converts pyruvate to acetaldehyde, releasing 1 CO2 in the process. 2. ADH (alcohol dehydrogenase) converts acetaldehyde to ethanol, converting 1NADH to 1 NAD+ in the process. Since 2 pyruvate are produced from glycolysis, that means alcohol fermentation releases 1 × 2 = 2 CO2 and regenerates 1 × 2 = 2 NAD+. Alcohol fermentation most commonly occurs in yeast. This is why yeast is used in baking bread (the CO2 released allows the bread to rise) and brewing alcohol (the ethanol produced is an alcohol). Figure 7: Alcohol fermentation (Source: Campbell’s 9th Edition) 16 USABO Guide Andrey Nikitin Example 2.4 (USABO Opens 2015) Solution: The whole point of fermentation is to regenerate the NAD+ so that glycolysis can proceed. In this scenario, NAD+ can not be regenerated. Therefore, glycolysis can not proceed and our answer is E. 2.5.2 Lactic Acid Fermentation Lactic Acid Fermentation converts the pyruvate from glycolysis into lactic acid in 1 step and does not release CO2. This type of fermentation occurs in human muscle cells after strenuous exercise and in making foods like yogurt. Figure 8: Lactic acid fermentation (Source: Campbell’s 9th Edition) 2.5.3 Anaerobic Respiration Despite what you may have been taught, anaerobic respiration is NOT the same thing as fermen- tation. Just like how cellular respiration uses the ETC, anaerobic respiration uses a special ETC of its own, except it uses something else in place of O2. The most common example is in purple sulfur bacteria, which use sulfate (SO2− 4 ) from hydrothermal vents. As a result, instead of making H2 O, they make H2 S, better known as hydrogen sulfide. 17 USABO Guide Andrey Nikitin 2.6 Gluconeogenesis As we have seen, cellular respiration allows a cell to break down glucose into ATP, the cell’s most usable form of energy. However, what happens when a cell runs out of glucose to use? Simple, the cell just needs to make more glucose! When a cell makes new glucose from other stores of energy, it is known as gluconeogenesis (genesis = making, neo = new, gluco = glucose). Essentially, the cell can convert its stores of energy into an intermediate in cellular respiration and since reactions are reversible, it’ll go through the steps backwards until it eventually gets turned into glucose. Depending on the source of energy it will get converted into a different intermediate: Carbohydrates can just be broken down into glucose Fats are made up of a glycerol, which can be converted into G3P, and fatty acid tails, which can be converted to acetyl CoA via beta oxidation. Beta oxidation also releases some NADH and FADH2 , which can be used in the ETC to generate more ATP. Proteins are made up of amino acids. Depending on the amino acid, it can be converted into a different intermediate in glycolysis: – Most of them are glucogenic and can be converted to: ∗ Pyruvate (CT SWAG) ∗ α-ketogluterate (REP HQ) ∗ Succinyl-CoA (MITV) ∗ Fumarate (PYD) ∗ Oxaloacetate (DN) – Some of them are ketogenic and are converted into Acetyl CoA (TWYP KIL). The Cori cycle can convert lactate (lactic acid) to glucose, which is then converted to lactate via lactic acid fermentation, which is then converted to glucose... and so on. Even though I said that gluconeogenesis was like cellular respiration but in reverse, that isn’t actually true since some steps were irreversible. To get around this issue, gluconeogenesis imple- ments some new enzymes: Instead of pyruvate dehydrogenase, PC (pyruvate carboxylase) is used. Instead of pyruvate kinase, PEPCK (PEP carboxykinase) is used. Instead of PFK, FBPase (fructose 1,6 biphosphatease) is used. Instead of hexokinase, G6Pase (glucose-6-phosphatase) is used. 18 USABO Guide Andrey Nikitin 3 Photosynthesis In many ways, photosynthesis is very similar to cellular respiration. They both occur in a special organelle, with photosynthesis occurring in the chloroplast. They both utilize electron carriers and contain an ETC of sorts, generating ATP. Even their reactions look similar, with photosynthesis simply being the reverse reaction of cellular respiration: 6CO2 + 6H2 O + energy → C6 H12 O6 + 6O2 Photosynthesis, seen in plants, phytoplankton, and some protists, captures light energy and con- verts it into glucose. It is split into the Light Reactions, which harvest the energy from light, and the Calvin cycle, which uses that energy to form glucose. 3.1 Photosystems Before we can understand the reactions of photosynthesis, we first need to understand how plants capture sunlight. They do so by utilizing pigments, molecules that can absorb light. Each pigment has an absorption spectrum, the range of wavelengths of light which it can absorb, and an action spectrum, the range of wavelengths of light which the pigment can use to drive a reaction. A pigment is useful when its absorption spectrum matches its action spectrum (that way it can do stuff with the light it is absorbing). Figure 9: The absorption spectra of the three major pigments in plants. Note that green light is one of the only wavelengths not absorbed by pigments. Instead, green light is reflected and hits our eyes, which is why most plants look green! (Source: Campbell’s 9th Edition) Most pigments are lipid-soluble and are thus incorporated into the thylakoid membrane. Here are different pigments you may see: Chlorophyll a: the universal pigment. 3/4 of pigments in green plants is chlorophyll a. Mg forms the center of the chlorophyll molecule. Accessory pigments: these are additional pigments that typically absorb wavelengths that chlorophyll a misses. Different organisms have unique accessory pigments: – Chlorophyll b: in plants, green algae, and euglenoids. – Chlorophyll c: in brown algae and diatoms. – Chlorophyll d and f: two recently discovered pigments that specialize in longer wave- lengths. 19 USABO Guide Andrey Nikitin – Bacteriochlorophyll: in purple sulfur bacteria. – Chlorobium chlorophyll: in green sulfur bacteria. – Phycobillin: in cyanobacteria and red algae. Water soluble. – Carotenoids: the red, orange, and yellow colored pigments that provide photoprotec- tion from UV light. Includes carotenes and xanthophylls. When a pigment is excited, one of 3 things can happen: 1. Flouresence: The pigment radiates light, which is functionally useless for photosynthesis. 2. Resonance energy transfer: Energy is transferred to neighboring pigment 3. The electron is transferred to the ETC, leaving an electron hole in the pigment. To take advantage of resonance energy transfer, hundreds of pigment molecules are arranged into a Photosystem. A photosystem consists of an antenna complex, where the antenna pigments receive the incoming sunlight and transfer that energy via resonance energy transfer to a reaction center in the middle of the photosystem, which uses a special pair of chlorophyll a molecules to transfer an electron to the ETC. The photosystem can also have additional light- harvesting complexes, which are antenna complexes that don’t contain a reaction center. Each photosystem harvests light to excite an electron, sending it along the ETC. However, that leaves behind an electron hole that must be filled. As you will see, electrons from water fill this hole for Photosystem II while electrons from plastocyanin fill this hole for Photosystem I. Example 3.1 (USABO Opens 2016) Solution: This question is simply asking about what wavelengths certain pigments absorb best. Beta-carotene is a carotenoid and as we can see in Figure 9, it absorbs blue light best. Therefore, it will have no effect on red-light absorption and our answer is D. 20 USABO Guide Andrey Nikitin 3.2 Light Reactions As the name suggests, the light reactions absorb the energy from incoming sunlight and convert it into ATP and NADPH, which are needed for making glucose. The light reactions come in two types: linear electron flow, which produces ATP and NADPH in a 1:1 ratio, and cyclic electron flow, which produces only ATP. Since the Calvin cycle requires a 3:2 ratio of ATP to NADPH, both processes are necessarry. 3.2.1 Linear Electron Flow This process involves both of the major photosystems involved in photosynthesis. These photosys- tems go by two names: one name is based on what wavelength of light they absorb best, and the other is based on the order of their discovery. Unfortunately, Photosystem II (P680) comes before Photosystem I (P700) in linear electron flow. The ultimate goal of linear electron flow is to reduce NADP+ , forming NADPH. Where will the H− to do so come from? Water! H2 O supplies the electrons that are passed from Photosystem II, along an ETC, to Photosystem I, which then passes it to another ETC that eventually passes it to NADP+ , forming NADPH. Figure 10: Diagram of linear electron flow. Each photosystem harvests light energy to excite an electron that gets sent along an ETC. The hole left by that electron is filled by the previous step. (Source: Raven) Photosystem II, which is located mainly in the grana thylakoids, contains a special oxygen- evolving complex (which uses Mn2+ ions). When Photosystem II takes electrons from water, it splits it into O and 2H+. This splitting of water by light is known as photolysis. The O becomes O2 (this is how plants produce oxygen in photosynthesis!) while the H+ is left inside the thylakoid to contribute to the electrochemical gradient used to make ATP. From there, Photosystem II passes the electron along an ETC: 21 USABO Guide Andrey Nikitin Pheophytin → Plastoquinone (PQa ) → Plastoquinone (PQb ) → Cytochrome b6 /f complex Figure 11: The proton-motive force generated by photolysis and the cytochrome b6 /f complex is used to produce ATP. (Source: Raven) The Cytochrome b6 /f complex mimics the ETC in cellular respiration, using the electron carriers that feed into it to pump protons. However, while in cellular respiration protons were pumped from inside (matrix) to outside (intermembrane space), here protons are pumped from outside (a fluid within chloroplasts called stroma) to inside (the thylakoid lumen). Then, this electrochemical gradient is used to power ATP synthase as protons flow out of the thylakoids, producing ATP! Cytochrome b6 /f complex → plastocyanin → Photosystem I Photosystem I, which is located mainly in the stroma thylakoids, uses the electron from plasto- cyanin to fill its electron hole. This hole was created when Photosystem I absorbed light, sending an electron along the following pathway: Phylloquinone (A1 ) → Iron-Sulfur proteins → Ferredoxin (Fd) → Flavoprotein → NADPH The final step is catalyzed by the enzyme FNR (Ferredoxin-NADP+ reductase). Since our reaction for photosynthesis uses 6 H2 O (which produced 6 O2 ), and each water molecule contributes 2 electrons (one from each H), then that means 6 × 2 = 12 NADPH are produced! 3.2.2 Cyclic Electron Flow Cyclic Electron Flow utilizes only Photosystem I. Photosystem I feeds an excited electron to the ETC, following the same pathway as in the last part of linear electron flow. However, once the electron reaches ferredoxin, instead of using it to make NADPH, that electron is fed back into the Cytochrome b6 /f complex. Electrons passing through this complex power proton pumps, which create an electrochemical gradient for ATP production! In addition, since no electrons are ever fed to FNR, no NADPH is produced! 22 USABO Guide Andrey Nikitin Figure 12: Diagram of cyclic electron flow. (Source: Raven) 3.3 Calvin Cycle/Light-Independent Reactions/Dark Reactions The Calvin Cycle is the stage of photosynthesis where sugar is made. Using the products of the light reactions, carbon dioxide can now be drawn from the air to form sugar through a process called carbon fixation. The Calvin Cycle occurs in the stroma of the chloroplast. Now we will look at how sugar is ultimately produced. 1. 1 CO2 from the air attaches to the 5-carbon ribulose bisphosphate (RuBP). The en- zyme that facilitates this process is called RuBP carboxylase-oxygenase, better known as RuBisCO. Rubisco is so important that it is the most common enzyme in the world! A short term 6-carbon intermediate molecule is created that is so energetic that it immediately splits in half, forming two 3-carbon compounds. Note that the oxygen from CO2 combines with hydrogen to produce 6 H2 O. Figure 13: RuBP fixes CO2 , producing 2 PGA. (Source: Raven) 23 USABO Guide Andrey Nikitin 2. The two compounds that formed are called 3-phosphoglycerate (PGA), which you may remember from glycolysis. The next two steps are essentially the reverse of glycolysis, using the ATP and then the NADPH that we generated during the light reactions to create G3P, or as it’s known in the Calvin Cycle, PGAL. During this process NADPH gets oxidized, recycling the NADP+ that was used up during linear electron flow. Figure 14: PGA is converted to PGAL, using ATP and NADPH. (Source: Raven) 3. Two molecules of G3P are ultimately used to make 1 molecule of glucose. Remember how we needed 6 CO2 to complete photosynthesis? Each CO2 molecule produces 2 G3P. However, the Calvin Cycle is a cycle, meaning that it must end with RuBP being regenerated. To do this, 5 molecules of G3P are put back into the cycle to produce 1 RuBP, using up another 3 ATP in the process. Therefore, to have a net of 1 G3P, 6 G3P must be produced in total since 6 − 5 = 1. Since each CO2 produces 2 G3P, it takes three turns of the Calvin cycle to produce 1 G3P. Since we need 2G3P to make glucose, there are six turns in total. Figure 15: A simplified diagram of the Calvin Cycle, showing carbons and phosphates. Keep in mind that the cycle happens twice to make 1 molecule of glucose. (Source: Khan Academy) 24 USABO Guide Andrey Nikitin In total, the Calvin Cycle utilized 6 CO2 to get 2 G3P, or one glucose molecule. This process required 18 ATP and 12 NADPH, which was provided by the light reactions. Example 3.2 (USABO Opens 2017) Solution: We know that photosynthesis produces O2 gas and glucose. Looking at the chart, we see that when H2 O is labeled, the gas is also enriched, showing that the oxygen gas in photosynthesis comes from water (remember photolysis). Similarly, when CO2 is labeled, the plant tissue is also enriched, showing that the oxygen in glucose comes from carbon dioxide (remember Calvin cycle). Therefore, our answer is C. 3.4 Photorespiration The process you just learned only occurs in C3 plants (name comes from first intermediate PGA being a 3-carbon molecule), which include most plants. However, it turns out that the enzyme that was so crucial for fixing carbon is actually pretty bad at its job. Rubisco has a lower affinity for CO2 than it does for oxygen! This means that if there is a lack of CO2 and O2 starts building up, as happens when the stomata are closed and there is no gas exchange, then rubisco will start binding to O2 , forming phosphoglycolate. This is a terrible situation and so the plant cell tries to make the best out of a bad position by performing a complicated process known as photorespiration, which involves the chlorplasts, mitochondria, and peroxisomes, wasting a bunch of ATP in the process! 25 USABO Guide Andrey Nikitin Figure 16: Rubisco binding to O2 results in phosphoglycolate. (Source: Raven) Since stomata are usually closed to prevent water loss from transpiration, photorespiration tends to become a big issue in hotter climates. To deal with this, C4 and CAM plants have evolved different pathways, which separate carbon fixation and the Calvin cycle. 3.4.1 C4 C4 plants, which include corn, sorghum, sugarcane, millet, switchgrass, and crabgrass, deal with photorespiration by implementing spatial separation, which means that carbon fixation and the Calvin cycle occur in different cells at the same time. If the problem is that O2 builds up, then we simply need to keep the rubisco in special cells that are far away from any O2. Also known as the Hatch-Slack pathway, C4 plants fix carbon in mesophyll cells and use rubisco in bundle-sheath cells. To facilitate this, the mesophyll cells are often in a wreath-like arrangement known as Kranz anatomy around the bundle sheath cells. Here is how the pathway works: 1. In mesophyll cells, CO2 is added to the 3-carbon PEP using the enzyme PEP carboxylase. This creates the 4-carbon sugar oxaloacetate. 2. Oxaloacetate is reduced to malate and aspartate, which are transported to the bundle sheath cells. There, it is broken back down into CO2 and pyruvate. The CO2 goes into the Calvin cycle as normal while the pyruvate goes back to the mesophyll cells to regenerate PEP. Although this process uses more ATP, the decreased risk of photorespiration makes it worth it. 26 USABO Guide Andrey Nikitin Figure 17: Diagram of the C4 pathway. (Campbell’s 9th Edition) Example 3.3 (USABO Opens 2016) Solution: The “layer of concentric mesophyll tissue” is describing Kranz anatomy, which means we are looking for a C4 plant. Therefore, our answer is B. Many questions will simply ask you to identify whether a plant is C3, C4, or CAM, so it’s a good idea to memorize the common examples. Example 3.4 (USABO Opens 2017) 27 USABO Guide Andrey Nikitin Solution: The goal of C4 plants is to minimize the amount of photorespiration in tropical climates by initially fixing CO2 onto PEP instead of RuBP. For this reason, A through D are all true. E is false, since C3 plants do not exhibit any form of separation while C4 plants exhibit spatial separation and, as you will soon see, CAM plants exhibit temporal separation. Therefore, the answer is E. 3.4.2 CAM CAM (Crassulacean Acid Metabolism) plants, which include orchids, cacti, aloe, pineapple, span- ish moss, and agave, deal with photorespiration by implementing temporal separation, which means that carbon fixation and the Calvin cycle occur in the same cell at different times. In other words, they will fix CO2 at night when their stomata are open and O2 levels are low using the same process that C4 plants use. The difference is that malate is converted to malic acid. Then, when it’s daytime the malic acid is broken down and the CO2 is used in the Calvin cycle. Figure 18: Comparison of C4 and CAM pathways. (Campbell’s 9th Edition) 4 Conclusion In photosynthesis, we saw how light energy can be transformed into glucose, which in cellular respiration was broken down into ATP. The reactions were complex and involved many steps, but by focusing on the basic ideas and going through each process slowly, we got through it. 28