Lippincott's Biochemistry Chapter 22 - Nucleotide Metabolism PDF
Document Details
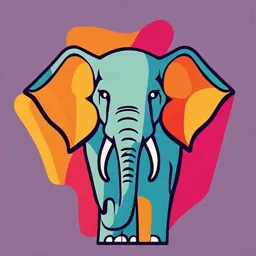
Uploaded by PrizeMeerkat
Tags
Summary
This chapter details nucleotide metabolism, covering topics such as the structure and function of nucleotides, purines, and pyrimidines. It also touches upon aspects of synthesis, degradation, and regulation. The summary emphasizes the importance of nucleotides in cellular processes.
Full Transcript
Nucleotide Metabolism I. OVERVIEW Ribonucleoside and deoxyribonucleoside phosphates (nucleotides) are essential for all cells. Without them, neither ribonucleic acid (RNA) nor deoxyribonucleic acid (DNA) can be produced, and, therefore, proteins cannot be synthesized or cells proliferate. Nucleotid...
Nucleotide Metabolism I. OVERVIEW Ribonucleoside and deoxyribonucleoside phosphates (nucleotides) are essential for all cells. Without them, neither ribonucleic acid (RNA) nor deoxyribonucleic acid (DNA) can be produced, and, therefore, proteins cannot be synthesized or cells proliferate. Nucleotides also serve as car- riers of activated intermediates in the synthesis of some carbohydrates, lipids, and conjugated proteins (for example, uridine diphosphate [UDP]- glucose and cytidine diphosphate [CDP]-choline) and are structural components of several essential coenzymes, such as coenzyme A, fla- vin adenine dinucleotide (FAD[HiJ), nicotinamide adenine dinucleotide (NAD[H]), and nicotinamide adenine dinucleotide phosphate (NADP[H]). Nucleotides, such as cyclic adenosine monophosphate (cAMP) and cyclic guanosine monophosphate (cGMP), serve as second messengers in signal transduction pathways. In addition, nucleotides play an impor- tant role as energy sources in the cell. Finally, nucleotides are impor- tant regulatory compounds for many of the pathways of intermediary metabolism, inhibiting or activating key enzymes. The purine and pyrimi- dine bases found in nucleotides can be synthesized de novo or can be obtained through salvage pathways that allow the reuse of the preformed bases resulting from normal cell turnover. [Note: Little of the purines and DNA and RNA Purtnes pyrimidines supplied by diet is utilized and is degraded instead.] 0 C::> NHa II. STRUCTURE N H ~Oc> N H Nucleotides are composed of a nitrogenous base; a pentose mono- Adenine (A) Guanlne(G) saccharide; and one, two, or three phosphate groups. The nitrogen- RNA Pyrimidines containing bases belong to two families of compounds: the purines and the pyrimidines. 0 A. Purine and pyrtmldlne bases HN~ J__H) Both DNA and RNA contain the same purine bases: adenine (A) and H guanine (G). Both DNA and RNA contain the pyrimidine cytosine (C), Uracil (U) but they differ in their second pyrimidine base: DNA contains thymine (1), whereas RNA contains uracil (U). T and U differ in that only T has DNA Pyrlmldlnas a methyl group (Fig. 22.1 ). Unusual (modified) bases are occasionally found in some species of DNA (for example, in some viral DNA) and Figure22.1 RNA (for example, in transfer RNA [tRNA]). Base modifications include Purines and pyrimidines commonly methylation, glycosylation, acetylation, and reduction. Some examples found in DNA and RNA. 291 292 22. Nucleotide Metabolism of unusual bases are shown in Figure 22.2. [Note: The presence of an Common Bue Unusual 811.ee unusual base in a nucleo1ide sequence may aid in its recognition by specific enzymes or protect it from being degraded by nucleases.] e. Nucleoeldas The addition of a pentose sugar to a base through an N-glycosidic bond (see p. 86) produces a nucleoside. If the sugar is ribose, a ribonucleoside is produced, and if the sugar is 2-deoxyribose, a deoxyribonucleoside is produced (Fig. 22.3A). The ribonucleosides 0 of A, G, C, and U are named adenosine, guanosine, cytidine, and H, II H2 uridine, respectively. The deoxyribonucleosides of A, G, C, and T have J) H1 H I the added prefix deoxy- (for example, deoxyadenosine). [Note: The compound deoxythymidine is often simply called thymidine, with the deoxy- prefix being understood, because it is incorporated into DNA Dlhydrouracll only.] The carbon and nitrogen atoms in the rings of the base and CHa, ,CHa the sugar are numbered separately (see Fig. 22.38). [Note: Carbons N in the pentose are numbered 1' to 5'. Thus, when the 5'-carbon of a (X) H nucleoside {or nucleotide) is referred to, a carbon atom in the pen- tose, rather than an atom in the base, is being specified.] C. Nucleotldes N8,N'·Dlmethyl· adenine The addition of one or more phosphate groups to a nucleoside pro- duces a nucleotide. The first phosphate group is attached by an ester Flgure22.2 Examples of unusual bases. linkage to the 5'-0H of the pentose, forming a nucleoside 5'-phos- phate or a 5'-nucleotide. The type of pentose is denoted by the pre- fix in the names 5'-ribonucleotide and 5'-deoxyribonucleotide. If one phosphate group is attached to the 5'-carbon of the pentose, the structure is a nucleoside monophosphate, like adenosine monophos- RNA DNA phate (AMP, or adenylate). If a second or third phosphate is added 0 {7 to the nucleoside, a nucleoside diphosphate (for example, adenos- ine diphosphate [ADP] or triphosphate, for example, ATP) results (Fig. 22.4). The second and third phosphates are each connected to HOfltv~ HOHaCv~ the nucleotide by a "high-energy bond' (a bond with a large, nega- 1 '"r---('J>H ~0H tive change in free energy [-AG, see p. 70] of hydrolysis). [Note: The OHOH OHH phosphate groups are responsible for the negative charges associ- ated with nucleotides and cause DNA and RNA to be referred to as Rlboae 2-IJ9oxyr1boM nucleic acids.] Ill. PURINE NUCLEOTIDE SYNTHESIS The atoms of the purine ring are contributed by a number of compounds, including amino acids {aspartate, glycine, and glutamine), carbon diox- ide (C02), and N10-tormyltetrahydrofolate (N10-tormyl-THF), as shown in OH OH Figure 22.5. The purine ring is constructed primarily in the liver by a series of reactions that add the donated carbons and nitrogens to a preformed Cytldlne Deoxyadenoalne ribose 5-phosphate. [Note: Synthesis of ribose 5-phosphate from glucose 6-phosphate by the pentose phosphate pathway is discussed on p.147.] Flgure22.3 A. Pentoses found in nucleic acids. A. 5-Phosphortbosyl-1-pyrophosphate synthesis B. Examples of the numbering systems for purine- and pyrimidina- 5-Phosphoribosyl-1-pyrophosphate (PAPP) is an activated pen- containing nucleosides. tose that participates in the synthesis and salvage of purines and Ill. Purine Nucleotide Synthesis 293 pyrimidines. Synthesis of PRPP from ATP and ribose 5-phosphate is catalyzed by PRPP synthstase (Fig. 22.6). This X-linked enzyme is activated by inorganic phosphate and inhibited by purine nucleotides (end-product inhibition). [Note: Because the sugar moiety of PRPP is ribose, ribonucleotides are the end products of de novo purine syn- oo·o-~ I I I -O- P-O- P- O- P-0- CHz 0 U II II thesis. When deoxyribonucleotides are required for DNA synthesis, 0 0 0 the ribose sugar moiety is reduced (seep. 297).] H H H H OH OH B. 5-Phoaphoribosylamine synthesis Albonucleoalde monophosphata Synthesis of 5-phosphoribosylamine from PRPP and glutamine is Rlbonucleosld shown in Figure 22.7.The amide group of glutamine replaces the pyro- diphosphate phosphate group attached to carbon 1 of PAPP. This is the committed Rlbonucleo81da step in purine nucleotide biosynthesis. The enzyme 1hat catalyzes the lrlpho11Phlde reaction, glutamine:phosphoribosylpyrophosphate amidotransferase (GPA1), is inhibited by the purine 5'-nucleotides AMP and guanosine Flgure22.4 monophosphate (GMP, or guanylate), the end products of the path- Ribonucleoside monophosphate, way. The rate of the reaction is also controlled by the intracellular con- diphosphate, and triphosphate. centration of PAPP. [Note: The concentration of PAPP is normally far below the Michaelis constant (Km) for the GPAT. Therefore, any small change in the PRPP concentration causes a proportional change in rate of the reaction (seep. 59).] C. lnoaine monophosphate synthesis The next nine steps in purine nucleotide biosynthesis leading to the synthesis of inosine monophosphate [IMP] whose base is hypoxan- thine) are illustrated in Figure 22.7. IMP is the parent purine nucleo- tide for AMP and GMP. Four steps in this pathway require ATP as an energy source, and two steps in the pathway require N 10-formyl-THF as a one-carbon donor (seep. 267). [Note: Hypoxanthine is found in tRNA (see Fig. 32.9 on p. 453).] Flgure22.5 Sources of the individual atoms D. Synthetic Inhibitors in the purine ring. The order in which the atoms are added Is shown by the Some synthetic inhibitors of purine synthesis (for example, the sul- numbers in the black boxes (see fonamides) are designed to inhibit the growth of rapidly dividing Fig. 22.7). CO:!= carbon dioxide. microorganisms without interfering with human cell functions (see Fig. 22.7). Other purine synthesis inhibitors, such as structural analogs ACTIVATOR INHIBITORS P1 @-OHzC~~ '\--(0H 1 ) ®-01-tzco o-®-® OH OH OH OH Rlboee 5-phosphlde 5-Ph08phorlboeyl-1-prrophoaphate (PAPP) Flgure22.6 Synthesis of PRPP. showing the activator and inhibitors of the reaction. [Note: This is not the committed step of purine synthesis because PRPP is used in other pathways such es salvage (see p. 296).] ® = phosphate; Pi inorganic = = = phosphate; AMP adenosine monophosphate; Mg magnesium. 294 22. Nucleotide Metabolism ~POH~Q Glutamine Glutamate ~POH2~Ntii! 0I 0II +HzO +PP1 o-p-o-p- o- \_.. __..?( ) Glycine +ATP OP~~ ~DP+P1 , I OH OH o- o- OH OH 5-Pl'toaphorlbollyl- 1-P'froPhoaphate 0 5-Phoephortboeytamlne GAR syn1hel:as8 INHIBITORS: yH2- NH2 AMP,GMP O =C, NH ~POH~~ ACTIVATOR: 10 PRPP N -Formyl- Tetrehydrofolate tetrahydrofol9le ( ~ _/ Rlbose 5-phosphate N.f'onnylglyclnamlde rlbollde (FGAR) Glyclnamlde rlbollde (GAR) ATP ~ '-...._ ~~~=----~~> ADP+ P1 ( H-N/-.....N ~· N I > PABA ANALOGS AIR.9)'fllllet8SB Sulfonamides aN structural analogs of Ribose 5-phosphate Ribose 5-phosphate para-aminobenzoic acid (PABA) that competltlvely Inhibit becterlal ayntheela N-Formylglyclnamldlne 5-AmlnolmldllZDle o1 follc acid. Becauee purine ayntlleala rlbollde (FGAM) rlbollde (AIR) require telrahydrotolate ae a coenzyme, co,i"- the auHa drugs alow down tllla pathway In baclBrla.. Humans cannot synthesize follc acid coo- a11 and must rely on extemal sources of this I ATP vttamln. Therefore, sulfa drugs do not HC - NH· Cx 9H2 coo- Hi!N I > N N OOC..............-N H~./'---N 1> Interfere wlllt human purine syntheele. FOLIC ACID ANALOGS Methonxate and related compounds I I Inhibit the reduction of dlhydrofolate Ribose 5-phosphate A ibose 5-phosphate to tetrahydrotolate, catalyzed by 5-Amlnolmldazole- C.rboxyamlnolmldllzole dlhydrotoltm! reductaae (aee p. 304). 4-(N-aucclnylocarboxamlde) rlbotlde (CAIR) rlbotlde (SAICAR) These drugs llmH the amount of telnhydrofolate avallabl for UH In purine synthesis and, thus, slow down DNA repllcatlon In mammallan cells. Therefore, Adenylosuoc/nBJBf-00:[:, these compounds are uaeful in treating rapldly growing cancers but are fyBSB toxic to all dividing celle. Furnande NH2 - C XN 0 I > \-,, N1D.f'ormyl tetrahydrololale AICAR Telnlhydrotalllte d ) Hi!N 0 ll ,...C~N 11 > IMP "20 ,2 ) l.~ > H 0 ~N HN N fDrmyfrransferaN HC- HNA N Cyclohyclrolase N N 2 I 0 I I Ribose 5-phosphllle Ribo&e 5-phosphate Ribose 5-phosphate 5-Amlnalmldazole- 5-Formamldalmldazole- lnoslne 5'-monophosphate 4-alrboxlmlde rlbollde 4-alrboxamlde rlbotlde (IMP) (AICAR) (FAICAR) Figura 22.7 De novc synthesis of purine nucleotides, showing the inhibitory effect of some structural analogs. AMP and ADP = adenosine mono- and diphosphates; GMP = guanosine monophosphate; PRPP = 5-phosphoribosyl-1-pyruphosphate; P1 = inorganic phosphate; PPi = pyrophosphate; C°'1 = carbon dioxide. Ill. Purine Nucfeotide Synthesis 295 of folic acid (for example, me1hotrexate), are used pharmacologically to control the spread of cancer by interfering with the synthesis of nucleo- tides and, therefore, of DNA and RNA (see Fig. 22.7). Inhibitors of human purine synthesis are extremely toxic to tis· sues, especially to developing structures such as in a fetus, or to cell types that normally replicate rapidly, including those of bone marrow, skin, gastrointestinal (GI) tract, Immune system, or hair follictes. As a result, individuals taking such anticancer drugs can experience adverse effects, including anemia, scaly skin, GI tract disturbance, immunodeficiency, and hair loss. E. Adenoalne and guanoslne monophoaphate synthesis The conversion of IMP to either AMP or GMP uses a two-step, energy- and nitrogen-requiring pathway (Fig. 22.8). [Note: AMP syn1hesis requires guanosine triphosphate (GTP) as an energy source and aspartate as a nitrogen source, whereas GMP synthesis requires ATP and glutamine.] Also, the first reaction in each pathway is inhibited by the end product of that pathway. This provides a mechanism for diverting IMP to the synthesis of the purine present in lesser amounts. "'OOC- CH2- yH-COO- O 0 JY) GDP+~ 7~jl_N ( '\.. GTP-..!. /2~POHV~ "(X>I "'--- \._ H,o - NADH+H+ ~ ;(~ x> ~le IAIPdehJlf/roglJtlstlB ) 2-0sPO~C 0 2 -0:,POH29 0 ~.r '" r---( rJ : OHOH 1 : OHOH OH OH ': lnoelne monophoephate (IMP) i X.nthoelna monophOllPflete Adanyloeucclnate ~ i Glutam;{ne.ATP ~ : GMP S)flllretaBe +... Glutamate Fumarate A~i. O MYCOPHENOLIC ACID AMP+ pp 0 :.X> 1 ~ NH2 This drug ls a rwverslble, o d Inhibitor of lnolJ/ne l7JOll()o ~dtlh~ H~ I ~ It deprtvea rapldty prollfarallng H-.. Z'""' A:::::.N N d 2-0sPO~C 0 TandBcellsofkey components of nucltlc acld8. 2-0sPO~C It I an lmmunoauppntA8nt ueed to prevent graft rejection. OH OH OH OH Adenoelne 111onophoepham (AMP) Ouanoelne monophoephllte (GllP) Figure22.8 Conversion of IMP to AMP (or, adenylate) and GMP {or, guanylate} showing feedback inhibition. NAD(H) nicotinamide = adenine dinucleotide; GDP and GTP =guanosine di- and triphoaphates; Pi =inorganic phosphate; PPi =pyrophosphate. 296 22. Nucleotide Metabolism Ben-llpecltlc nucleo$lde If both AMP and GMP at'9 present in adequate amounts, the dt novo monophosphste klnasss pathway of purine nucleotide synthesis is inhibited at the GPATstep. Adenyfafle kinase AMP+ ATP 2 ADP F. Nucleoslde di· and tr1phosphate synthesis GMP + ATP GU8J'J)'fele ldnllSe GDP +.ADP Nucleoside diphosphates are synthesized from the corresponding Nucleoslde dlphosphate kinase nucleoside monophosphates by base-specific nucleoside monophos- phate kinases (Fig. 22.9). [Note: These ldnases do not discriminate GDP+ATP ==~ GTP+ADP between ribose or deoxyribose in the substrate.] ATP is generally the CDP+ATP =====- CTP +ADP source of the transferred phosphate because it is present in higher concentrations than the other nucleoside triphosphates. Adenylate Flgure22.9 kinase is particularly active in the liver and in muscle, where the turn- Conversion of nucleoside over of energy from ATP is high. Its function is to maintain equilibrium monophosphates to di- and among the adenine nucleotides (AMP, ADP, and ATP). Nucleoside trlphosphates. AMP and ADP= diphosphates and triphosphates are interconverted by nucleoside adenosine mono- and diphosphates; diphosphate kinase, an enzyme that, unlike the monophosphate GMP, GDP, and GTP ::= guanosine kinases, has broad substrate specificity. mono-, di-, and triphosphates; CDP and CTP =cytidine di- and triphosphates. G. Purtne salvage pathway Purines that result from the normal turnover of cellular nucleic acids, or the small amount that is obtained from the diet and not degraded, can be converted to nucleoside triphosphates and used by the body. This is referred to as the salvage pathway for purines. [Note: Salvage is particularly important in the brain.] 1. Purine base salvage to nucleo1ldes: Two enzymes are involved: adenine phosphoribosyttransferase (APR1) and X-linked hypo- xanthin&-guanine phosphoribosyltransferase (HGPRT). Both use PRPP PP1 PAPP as the source of the ribose 5-phosphate group (Fig. 22.10). Hypounttilne \.. I /( ) IMP lhe release of pyrophosphate and its subsequent hydrolysis by pyro- Hypoanrhlf».gwnlne phosphatass makes these reactions irreversible. [Note: Adenosine ~ is the only purine nucleoside to be salvaged. It is phosphorylated to (HGPRT) AMP by adenosine kinase.] PRPP PP1 \.. I /( ) GMP 2. Lesch-Nyhan syndrome: This is a rare, X-linked recessive dis- Hypoan~ order associated with a virtually complete deficiency of HGPRT. phollphollbosylsletue The deficiency results in an inability to salvage hypoxanthine (HGPR1) or guanine, from which excessive amounts of uric acid, the end product of purine degradation, are then produced (see p. 298}. In Adenine addition, the lack of this salvage pathway causes increased PRPP levels and decreased IMP and GMP levels. As a result, GPAT (the regulated step in purine synthesis) has excess substrate and decreased inhibitors available, and de novo purine synthesis is Figure 22.10 increased. The combination of decreased purine reutilization and Salvage pathways of purine increased purine synthesis results in increased degradation of nucleotide synthesis. [Note: Virtually purines and the production of large amounts of uric acid, making complete deficiency of HGPRT results In Lesch-Nyhan syndrome. HGPRTdeficiency an inherited cause of hyperuricemia. In patients Partial deficiencies of HGPRT are with Lesch-Nyhan syndrome, the hyperuricemia frequently results known. As 1he amount of functional in the formation of uric acid stones in the kidneys (urolithiasis) and enzyme increases, 1he severity of the the deposition of urate crystals in the joints (gouty arthritis) and symptoms decreases.] IMP. GMP, soft tissues. In addition, the syndrome is characterized by motor and AMP =inosine, guanosine, and adenosine monophosphates; dysfunction, cognitive deficits, and behavioral disturbances that PAPP= 5-phosphorlbosyl-1· include self-mutilation (for example, biting of lips and fingers), as pyrophosphate; PP1 =P'frophosphate. shown in Figure 22.11. IV. Deoxyribonucleotide Synthesis 297 IV. DEOXYRIBONUCLEOTIDE SYNTHESIS The nucleotides described thus far all contain ribose (ribonucleotides}. DNA synthesis, however, requires 2'-deoxyribonucleotides, which are produced from ribonucleoside diphosphates by the enzyme ribonuclso- tide rsductase during the S-phase of the cell cycle (seep. 423}. [Note: The same enzyme acts on pyrimidine ribonucleotides.] A. Rlbonucleotlde reductase Ribonucleotids reductase (ribonuc/eoside diphosphats reductase) is a dimer composed of two nonidentical subunits, R1 (or, a) and the smaller R2 (or,~), and is specific for the reduction of purine nucleo- side diphosphates (ADP and GDP) and pyrimidine nucleoside diphos- phates (CDP and UDP) to their deoxy forms (dADP, dGDP, dCDP, and dUDP). The immediate donors of the hydrogen atoms needed for the reduction of the 2'-hydroxyl group are two sulfhydryl (-SH) groups on the enzyme itself (A1 subunit), which form a disulfide bond during the reaction (see p. 19). [Note: R2 contains the stable tyrosyt radical Figure 22.11 required for catalysis at R1.] Lesions on the lips of a patient with Lesch-Nyhan syndrome. 1. Reduced enzyme regeneration: In order for ribonucleotide reductase to continue to produce deoxyribonucleotides at R1, the disuHide bond created during the production of the 2'-deoxy carbon must be reduced. The source of the reducing equivalents is thiore- doxin, a protein coenzyme of ribonucleotide reductase. Thioredoxin contains two cysteine residues separated by two amino acids in the peptide chain. The two -SH groups of thioredoxin donate their hydrogen atoms to ribonucleotide reductase, forming a disuHide bond in the process {Fig. 22.12). CJ o- -o-fi=o -o-p=O 2. Reduced thloredoxln regeneration: Thioredoxin must be 6 6 converted back to its reduced form in order to continue per- -O- ~= O -o-P=O forming its function. The reducing equivaJents are provided CH2 9 CH2 by NADPH + W, and the reaction is catalyzed by thiore- doxin reductase, a selenoprotein (see p. 268}. B. Deoxyrlbonucleotlde syn1hesls regulation OH OH ATP OH H Ribonucleotids reductase is responsible for maintaining a Rlbonuclaoelde 0 dATP Deoxyrlbonucleoelde balanced supply of the deoxyribonucleotides required for dlphoephate 0 dlphoephate DNA synthesis. Consequently, the regulation of the enzyme is complex. In addition to the catalytic site, R1 contains two )>~remaw{ distinct allostenc sites involved in regulating enzymic activity ThloNdoxln (2 SH) HaO Thloredoxln (S-S) (Fig. 22.13). 1. Ac11vlty sites: The binding of dATP to allosteric sites (known as activity sites) on R1 inhibits the overall cata- lytic activity of the enzyme and, therefore, prevents the NADp+ > (reduced) ~~ 90% of individuals with hyperuri- cemia, the cause is underexcretion of uric acid. Underexcretion Figure 22.14 can be primary, because of as-yet-unidentified inherent excre- Digestion of dietary nucleic acids. tory defects, or secondary to known disease processes that Pi"' inorganic phosphate. affect how the kidney handles urate (for example, in lactic acidosis, lactate increases renal urate reabsorption, thereby decreasing its excretion) and to environmental factors such as the use of drugs (for example, thiazide diuretics) or exposure to lead (saturnine gout). b. Uric acid overproduction: A less common cause of hyperuri- cemia is from the overproduction of uric acid. Primary hyperuri- cemia is, for the most part, idiopathic (having no known cause). However, several identified mutations in the gene for X-linked PRPP synthetase result in the enzyme having an increased max- imal velocity ([Vmax] seep. 57) for the production of PRPP, a lower Km (see p. 59) for ribose 5-phosphate, or a decreased sensitivity to purine nucleotides, its allosteric inhibitors (seep. 62). In each case, increased availability of PRPP increases purine production, 300 22. Nucleotide Metabolism ADENOSINE DEAMINASE (ADA) DEFICIENCY '11'1i aloltoeomal·l"8088aive deficiency caueee a type of SGV8l9 combined lmmunodellclency (SCID), Involving T-oell, B-eell, and ntdural klller-cell depletion (lymphocytopenla). Untreated ADA-dtficitnt children ueuaJty die before age 2 yeara from overwhelming Infection; PURINE NUCLEOSIDE tretdments Include BMT, ERT, and gene therapy. PHOSPHORYLASE (PNP) DEFICIENCY Thi autosomaJ..ruc:ealve deficiency Is rarer and lea& aevere than ADA dallclency. It atlecta T cell development, prtmartly. PNFLdDftclent Individual have recurrent lnfecdons and nauradevelopmental delay·~·------~ GOUT Thia disorder I chmactarlzed by hyperurtcemla with recurrent attack8 of acute arttlrHlc folnt Rlboee lnftammatlon, caueed by depollHlon of mono- 1-phoephate eadlum ulllte crystals. Gual1081ne monophoephall8 ~T"'° In gout, the hyperurlcemla reeuHe prtmartly from 0 the underexcretlon of uric acid. Overproduction of uric acid i.... common, and known cauM8 HN~N> Involve certain Inborn errore of metabollem or lncl'9Med avallablllty of purines. ~N~N ~P1 [2J H Cryabll dapoelllon (tophl) may be nen In 8oft Hypounthlne tlssuee and In the kfdney& (urolHhlaels). Guanoslne Treatment wHh allopurlnol Inhibits Dnthhw oJddan, resuHlng In accumulation of hypoxanthlne and xantltlne, compound more aoluble than uric acid. ==;J:::_ ~ 1·phoeph 0 HNAy N~ HtN~N~N/ H Guanine Figure 22.15 The degradation of purine nucleotides to uric acid, illustrating some of the genetic diseases associated with this pathway. [Note: The numbers in brackets refer to the corresponding numbered citations in the text.] BMT =bone marrow transplantatton; ERT =enzyme replacement 1herapy; P1 = Inorganic phosphate; H20:? = hydrogen peroxide; NHs =ammonia. resuHing in elevated levels of plasma uric acid. Lesch-Nyhan syn- drome (see p. 296) also causes hyperuricemia as a resuH of the decreased salvage of hypoxanthine and guanine and the subse- quent increased availability of PAPP. Secondary hyperuricemia is typically the consequence of increased availability of purines (for example, in patients with myeloprolifarative disorders or who are undergoing chemotherapy and so have a high rate of call turnover). Hyperuricemia can also be the result of seemingly V. Purine Nucleotide Degradation 301 unrelated metabolic diseases, such as von Gierke disease (see Fig.11.8 on p.130) or hereditary fructose intolerance (see p. 138). A diet rich In meat, seafood (particularly shellllsh), and ethanol Is associated with Increased risk of gout, whereas a diet rich 11 in low-fat dairy products is associated with a decreased risk. c. Treatment: Acute attacks of gout are treated with anti-inflam- matory agents. Colchicine, steroidal drugs such as predni- Figure 22.16 sone, and nonsteroidal drugs such as indomethacin are used. Tophaceous gout. [Note: Colchicine prevents formation of microtubules, thereby decreasing the movement of neutrophils into the affected area. Like the other anti-inflammatory drugs, it has no effect on uric acid levels.] Long-term therapeutic strategies for gout involve Arthrocentula: Joint uplratlon le a proc:edunt wll ntby a.terlle lowering the uric acid level below its saturation point (6.5 mgfdl), needle and sydnge are used thereby preventing the deposition of MSU crystals. Uricosuric to drain tluld from a Joint. agents, such as probenecid or sulfinpyrazone, that increase renal excretion of uric acid, are used in patien1s who are under- excretors of uric acid. Allopunnol, a structural analog of hypo- xanthine, inhibits uric acid synthesis and is used in patients who are overproducers of uric acid. Allopurinol is oxidized to oxypu- rinol, a long-lived inhibitor of XO. This results in an accumula- tion of hypoxanthine and xanthine (see Fig. 22.15), compounds more soluble than uric acid and, therefore, less likely to initi- ate an inflammatory response. In patients with normal levels of HGPRT, the hypoxanthine can be salvaged, reducing the levels of PRPP and, therefore, de novo purine synthesis. Febuxostat, Figure 22.17 a nonpurine inhibitor of XO, is also available. [Note: Uric acid Analysis of joint fluid can help to levels in the blood normally are close to the saturation point. define causes of joint swelling and One reason for this may be the strong antioxidant effects of arthritis, such as infection, gout, and uric acid.] rheumatoid disease. 2. Adenosine deaminase deficiency: ADA is expressed in a vari- ety of tissues, but, in humans, lymphocytes have the highest activ- ity of this cytoplasmic enzyme. A deficiency of ADA results in an accumulation of adenosine, which is converted to its ribonucleotide or deoxyribonucleotide forms by cellular kinases. As dATP levels rise, ribonucleotide rsductase is inhibited, thereby preventing the production of all deoxyribose-containing nucleotides (seep. 297). Consequently, cells cannot make DNA and divide. [Note: The dATP and adenosine that accumulate in ADA deficiency lead to devel- opmental arrest and apoptosis of lymphocytes.] In its most severe form, this autosomal-recessive disorder causes a type of severe combined immunodeficiency disease (SCIO), involving a decrease Figure 22.18 in T cells, B cells, and natural killer cells. ADA deficiency accounts Gout can be diagnosed by the for -14% of cases of SCIO in the United States. Treatments include presence of negatively birefringent bone marrow transplantation, enzyme replacement therapy, and monosodium urate crystals in gene therapy (see p. 501 ). Without appropriate treatment, children aspirated synovial fluid examined with this disorder usually die from infection by age 2 years. [Note: by polarized light microscopy. Here, crystals are seen within Purine nuclsosids phosphory/ass deficiency results in a less polymorphonuclear leukocytes. severe immunodeficiency primarily involving T cells.] 302 22. Nucleotide Metabolism Amide nitrogen VI. PYRIMIDINE SYNTHESIS AND DEGRADATION (R-troup) of glutamlne Unlike 1he synthesis of the purine ring, which is constructed on a pre- ~ c ~/ '? existing ribose 5-phosphate, 1he pyrimidine ring is synthesized before being attached to ribose 5-phosphate, which is donated by PRPP. The c, , c J" N sources of the atoms in the pyrimidine ring are glutamine, C02, and aspartate (Fig. 22.19). COii A. Csrbamoyl phosphate synthesis Figure 22.19 Sources of the individual atoms in the The regulated step of this pathway in mammalian cells is the syn- pyrimidine ring. C02 =carbon dioxide. thesis of carbamoyl phosphate from glutamine and C02, catalyzed by carbamoyl phosphate synthetase (CPS) II. CPS II is inhibited by uridine triphosphate (the end product of this pathway, which can be converted into the other pyrimidine nucleotides) and is activated by PRPP. [Note: Carbamoyl phosphate, synthesized by CPS I, is also a precursor of urea (seep. 253). Defects in ornithine transcarbamylase of the urea cycle promote pyrimidine synthesis because of increased availability of carbamoyl phosphate. A comparison of the two enzymes Variable CPSI CPS II is presented in Figure 22.20.] Cellular Mitochondria Cytoeol locatlon B. Orotlc acid synthesis Pathway Ureaeycle Pyrimidine The second step in pyrimidine synthesis is the formation of carbamo- Involved ayntheal ylaspartate, catalyzed by aspartate transcarbamoylase. The pyrimi- Source of Ammonia -r-Amlde dine ring is then closed by dihydroorotase. The resulting dihydroorotate nitrogen group of glutamlne is oxidized to produce orotic acid (orota.te}, as shown in Figure 22.21. The human enzyme that produces orotate, dihydroorotate dehydroge- Regullltonl Activator: Activator. nase, is a flavin mononucleotide-containing protein of the inner mito- N-acetyl· PRPP glutamate chondrial membrane. All o1her enzymes in pyrimidine biosynthesis Inhibitor: are cytosolic. [Note: The first three enzymic actMties in this pathway UTP (CPS II, aspartate transcarbamoylase, and dihydroorotase) are actu- ally three different catalytic domains of a single polypeptide known Figure 22.20 as CAD from the first letter in the name of each domain. (See p. 18 Summary of the differences for a discussion of domains.) This is an example of a multifunctional between catbsmoy/ phosphate or multicata.lytic polypeptide that facilitates the ordered synthesis of synth6taS9 (CPS) I and JI. PRPP = an important compound. Synthesis of the purine nucleotide IMP also 5·phosphor1bosyl·1-pyrophosphate; UTP =uridine triphosphate. involves multifunctional proteins.] C. Pyrimidine nucleotide synthesis The completed pyrimidine ring is converted to the nucleotide oroti- dine monophosphate (OMP) in the second stage of pyrimidine nucle- ofide synthesis (see Fig. 22.21). As seen with the purines, PRPP is the ribose 5-phosphate donor. The enzyme orotate phosphoribos- yltransfsrase produces OMP and releases pyrophosphate, thereby making the reaction biologically irreversible. [Note: Both purine and pyrimidine synthesis require glutamine, aspartic acid, and PRPP as essential precursors.] OMP (orotidylate) is decarboxylated to uridine monophosphate (UMP) by orotidylate decarboxylass. The phospho- ribosyltransferase and dscarboxylass activities are separate catalytic domains of a single polypeptide called UMP synthase. Hereditary orotic aciduria (a very rare disorder) may be caused by a deficiency of one or both activities of this bifunctional enzyme, resulting in orotic acid in the urine (see Fig. 22.21). UMP is sequentially phosphorylated VI. Pyrimidine Synthesis and Degradation 303 2ADP+P1 +Glutamate -oJ? H2') 0 ~H2 A8partate P1 c, 2ATP+C0i /( ) c=o \,. /( ) H2~ yH2 2 ) HN: : rli:! +Glulamlne Carbamoyt phosphate 0' Paa~ ~ Aspattafe O=C, N,,..CH~coo- Dihydtoototase ~N &o- s~ll H H C.rba= phosp......... C.rbamoyl Dlhydroorotate OROTIC ACIDURIA REGULATION OF PYRIMIDINE SYNTHESIS Orotate phosphorlbosyttransferaee and OllP In mammalian calla, the CllTIHunoyl p/ro9phafll det:arboxylan are eaparata catalytic domalna ynllnlllJ 11 domain of trlfunctlonal CAD la lnhlblblcl by UTP and activated by PRPP. of a Ingle polypepllde, UllP ynthllae. In prokaryo'ltc calla, a.,_.,.,.ll'anBcatf.lamo,,_ la Inhibited by CTP and 18 Ille regulated trtep. Low activity of either or both domains results In poor growth, megaloblastic anemia. and the excrellon of large amounts of orotate In the urine. FMN Admlnllllnltlon of urtdlne raulbl In lmprowmant of the anemia and decl'98Hd excrellon ot oroblla. 0 HNP HNP a FMNHi 0-lNJ ol-.N) coo- ~"td cea 2-o,POH29Q PP, PRPP a ( ~ ~/ HNP OMP ~ ( Orofat8 phosp/lolfbosyf- tnmshws8 J-.N) coo- HO OH HO OH H Urldlne 5'-mon~hosphalB Orotldlna 5'-monoph09phllle Oro1ale (UMP) (OMP) Figure 22.21 ~ novo pyrimidine synthesis. ADP = adenoslne dlphosphate; P1 = Inorganic phosphate; FMN(H.2) = tlavln mononucleotlde; CTP = cytidine triphosphate; PAPP = &-phosphoribosyl-1-pyrophosphate; PP1 = pyrophosphate. to UDP and UTP. [Note: The UDP is a substrate for ribonucleotids reductase, which generates dUDP. The dUDP is phosphorylated to dUTP, which is rapidly hydrolyzed to dUMP by UTP diphosphatase (dUTPase). Thus, dUTPase plays an important role in reducing avail- ability of dUTP as a substrate for DNA synthesis, thereby preventing erroneous incorporation of uracil into DNA.] Urldlne b'lphoephate (UTP) D. Cytldlne trlphosphate synthesis Glutamlne ATP Cytidine triphosphate (CTP) is produced by amination of UTP by CTP CTP synthetase synthetase (Fig. 22.22), with glutamine providing the nitrogen. Some of this CTP is dephosphorylated to CDP, which is a substrate for ribo- Glutamate nucleotide reductase. The dCDP product can be phosphorylated to dCTP for DNA synthesis or dephosphorylated to dCMP that is deami- Cytldlne trlphoapturte (CTP) nated to dUMP. E. Deoxythymldlne monophosphate synthesis Figure 22.22 dUMP is converted to deoxythymidine monophosphate (dTMP) by thy- Synthesis of CTP from UTP. [Note: midylate synthase, which uses N5 ,N10-methylene-THF as the source of CTP, required for RNA synthesis, is converted to dCTP for DNA the methyl group (see p. 267). This is an unusual reaction in that THF = synthesis.] ADP adenosine contributes not only a one-carbon unit but also two hydrogen atoms diphosphate; P1 =inorganic from the pteridine ring, resulting in the oxidation ofTHF to dihydrofolate phosphate. 304 22. Nucleotide Metabolism dCDP NHs dUDP ([DHF], Fig. 22.23). Inhibitors of thymidyfate synthase include thy- ~P1 j ~P1 mine analogs such as 5-fluorouracil, which serve as antitumor agents. 5-Fluorouracil is metabolically converted to 5-fluorodeoxyuridine mono- dCMP ---~--) dUMP phosphate {5-FdUMP), which becomes permanenUy bound to the inac- tivated thymidylate synthass, making the drug a suicide inhibitor (see p. 60}. DHF can be reduced to THF by dihydrofolate reductase (see Fig. 28.2, p. 378), an enzyme that is inhibited by folate analogs such as 00-Fluorouracll methotrexate. By decreasing the supply of THF, these drugs not only ~ S-FdUMP 0 inhibit purine synthesis (see Fig. 22.7), but, by preventing methytation of dUMP to dTMP, they also decrease the availability of this essential component of DNA. DNA synthesis is inhibited and cell growth slowed. Dlhydrofolate Thus, these drugs are used to treat cancer. [Note: Acyclovir (a purine analog} and Azr (3'-azido-3'-deoxythymidine, a pyrimidine analog) are NADPH+W used to treat infections of herpes simplex virus and human immuno- deficiency virus, respectively. Each inhibits the viral DNA po/yme.taS8.] F. Pyrimidine salvage and degradation NADp+ Unlike the purine ring, which is not cfeaved in humans and is excreted Tetrahydrofolllte as poorty soluble uric acid, the pyrimidine ring is opened and degraded to highly soluble products, f)-alanine (from the degradation of CMP and d'TNP UMP) and f)-aminoisobutyrate (from TMP degradation), with the produc- tion of ammonia and CD2. Pyrimidine bases can be salvaged to nucle- Figure 22.23 osides, which am phosphorylated to nucleotides. However, their high Synthesis of dTMP from dUMP. solubility makes pyrimidine salvage less significant clinically than purine illustrating sites of action of salvage. [Note: The salvage of pyrimidine nucleosides is the basis for antlneoplasllc drugs. using uridine in the treatment of hereditary omtic aciduria (see p. 302).] VII. CHAPTER SUMMARY Nucleotidee are composed of a nitrogenoue beae (adenine= A, guanine = G, cytoaine = C, uracil = U, and thymine= T}; a pentoee sugar; and one, two, orihree phosphate g10ups (Fig. 22.24).A and Gare purtnes, and C, U, and Tare pyrimidines. If 1he sugar is rtbo8e, 1he nudeotide is a rtbonucleoslde phosphate (for example, adenosine monophosphate [AMP]), and it can have several functions in the cell, including being a component of ANA. If the sugar is deoxyltbole, the nudeotide is a deaxyltbonuclaoslde phosphate (for example, deoxyAMP) and wlll be found almost excluslvely as a component of DNA. The committed step In purine synthesis uses 5-phospharlboayl-1-pyrophosphata ([PRPP], an activated pentose that provides 1he rlbose 5-phoaphalB for d.i ~ purine and pyrimidine synthesis and salvage) and nitrogen from glutamlne to produce phosphoribosylamine. The enzyme is glu'11rn/ne:phasphotlbosyipyruphosphllls amldotnmslBrue and is inhibited by AMP and guanosine monophosphate (the end products of the pathway) and activated by PRPP. Purine nucleotides can also be produced from preformed purine bases by using salvage raactlonscatalyzed by adenlnephosphadbosyltransfwue (APR1) and hypox.anlhlne-gu ~(HGPR1). A near-total deficiency of HGPRTcauses Leech-Nyhan eyndrome, a severe, inherited fonn of hyperuricemia acx:ompanied by compulsive self-mutilation. All deoxyribonucleotides are syn1hesized from ribooocleotides by 1he enzyme ribonudeotide reducta.11e. This enzyme is highly regulated {for example, it is strongly inhibited by deoxyadenoelne trlphoaphate [dATP], a cc:w\1)0und that is Oll'erproduced in bone marrow cells in individuals with tJdenotl/ne deemlntlse [AfM] deficiency). All4 deficiency causes MVere combined Immunodeficiency dlleese.The end product of purine degradation is urtcecld, a compound of lowsolubilitywhoseoverproduc:tion or undeisecretion causes twvPerurlCMnla that, tf accompanied by 1he deposlllon of monoaodlum urata crystals In joints and soft tissues and an Inflammatory response to those crystals, results In gout. The first step In pyrtmlclne aynthaals, the production of carbamcyt phosphate by c:srbsmoyl phosphatB synthetase H, is 1he regulated step in 1his pathway (it is inhibited by urldlne 1rlphosphate [UTP] and activated by PRPP). The lJTP produced by 1his pathway can be converted to cylidine triphosphate. Daoxyurldlne monophoephata can be COl'Nerted to deoxythymldlM monophoaphate by thym/dylale synthase, an enzyme targeted by anticancer drugs such as 5-fluoftluracll. The regeneration of 18b'ahydrofolat8 from dihydrof'olate produced in the thymicJt/ll:lte synthase reaction requires dlhydtolo/llte reductue, an enzyme 1argeted by the drug methotrexate. Pyrimidine degradation results in soluble producta. VII. Chapter Summary 305 Nucleotlde atructU1'8 Purtne mstabollam Pyrimidine ayntheelt SYNTHl!llS 2ATP+CO:! BASE BASE +SUGAR +Glutamine + PHOSPHAlE = Rlboee S-photpttate AMP GMP NUCl.EOTEE UTP 0 Regullded t Adenine dAMP S.Phoaphorbosyl-1~ (PAPP) t Guanoelne PRPP O Gip CPSll I ~lalad j AMP, GNP 0 recunaor DNA Guanine dOMP PRPP O GPAT molecules t Guanine Carbamoyt ph0Bphal8 p~~~ Glutamlna l-Aspertata Cyl:oelne dCMP I Adenoalne C8rtl8lllO'jl aapalUlte Thymine dTUP S.Phmphor!boaylamlne t Glycine t AtlA Olhydrborotata a1ye1namlda rlbalfde {GAR) N10-Formy._THF lnoalne.- IMP I Orotatll BASE BASE +SUQAR t N-Foonytglyelnamlda rlbaClda {FGAR) Glutamina t Hypounthlne +PHOSPHATE I Orotidine 6'· NUCLEOTllE )(0 mcnophoaphate (OMP) N.f'ormylglyclnamldlna rlbollde (FGAM) Adenine AMP f loUda (SAICAR) UTP -. CTP Uracil UMP I 5-AmlnolmldaZol~lda rlloUde (AICAR) Inherited deficiency I Urtoecld In UMP synthase 5-Fonnamldolmldazolll· (urtne) +