Body Fluids & Electrolytes PDF
Document Details
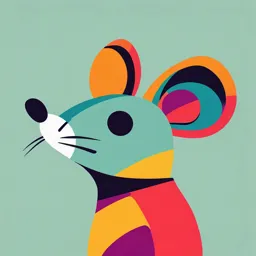
Uploaded by ResoundingDatePalm6640
Ms. Yeama Singh
Tags
Summary
This document provides an overview of body fluids, specifically focusing on water purification methods and electrolytes. It explains the importance of purity in laboratory settings and discusses various purification processes and their implications for different types of research. It also discusses the importance of electrolytes and their variations based on body composition.
Full Transcript
BODY FLUIDS PREPARED BY: MS.YEAMA SINGH TOPICS ◼ Water ◼ Electrolytes ◼ Acid Base Balance ◼ Anion Gap ◼ Osmolality ◼ Laboratory Considerations ROUTINE TESTING OF WATER ◼ Several methods are available to test for water purity. ◼ In the presence of inorganic ionized materials, as the purity of the...
BODY FLUIDS PREPARED BY: MS.YEAMA SINGH TOPICS ◼ Water ◼ Electrolytes ◼ Acid Base Balance ◼ Anion Gap ◼ Osmolality ◼ Laboratory Considerations ROUTINE TESTING OF WATER ◼ Several methods are available to test for water purity. ◼ In the presence of inorganic ionized materials, as the purity of the water increases, the amount of dissolved ionized substances decreases, and the ability of the water to conduct an electrical current decreases. ◼ This principle is used in commercially available resistance test analyzers for water purity. As the ability of the water to conduct an electrical current decreases, the resistance increases. the presence of ionizable contaminants in distilled water or deionized water is most easily determined by measuring the conductance, or electrical resistance, of the water. ◼ This is the basis for having purity meters or conductivity warning lights on distillation and deionization apparatus. TESTING OF WATER ◼ Water of the highest purity will vary with the method of preparation and may be referred to as nitrogen-free water, double-distilled water, or conductivity water, depending on the actual method used. ◼ A measure of conductance does not consider the presence of non-ionized substances (organic contaminants) such as dissolved gases. Especially important in the clinical laboratory is dissolved carbon dioxide (CO,). ◼ Water free of such dissolved gases may be obtained by boiling it immediately before use and is often referred to as gas-free water or CO,-free water. Such water may be necessary for the preparation of strongly alkaline solutions. ◼ Another contaminant of water may be substances dissolved from the storage container. TESTING OF WATER ◼ The classification of and specifications for water purity are designed, for example, to enable laboratory personnel to specify the quality of the water needed for particular laboratory analyses and reagent preparation. ◼ Each test performed in the laboratory must be evaluated as to the type of water needed, to avoid potential interference with specificity, accuracy, and precision. ◼ It is well known, for example, that water contaminated with metal, when used in analyses of enzymes, can have a dramatic effect on the values obtained. STORAGE OF REAGENT WATER ◼ It is important to store reagent water appropriately. ◼ Type I water must be used immediately after its production to prevent CO 2 from being absorbed into it. ◼ Types II and III water can be stored in borosilicate glass or polyethylene bottles but should be used as soon as possible to prevent contamination with airborne microbes. ◼ Containers should be tightly stoppered to prevent absorption of gases. It is also important to keep the delivery system for the water protected from chemical or microbiological contamination. PURIFICATION OF WATER PROCESS ◼ The original source of water varies greatly with the health care facility. Water originating from rivers, lakes, springs, or wells contains a variety of inorganic, organic, and microbiological contaminants. ◼ No single purification system can remove all the contaminants. For this reason, a variety of methods in differing combinations are used to obtain the particular types of water used in a laboratory facility. ◼ Two general methods are employed to prepare water for laboratory use: deionization and distillation. Sometimes it is necessary to treat distilled water further with a deionization process to obtain water with the degree of purity needed. DISTILLED WATER ◼ In the process of distillation, water is boiled and the resulting steam is cooled; condensed steam is distilled water. ◼ Many minerals are found in natural water, most often iron, magnesium, and calcium. Water from which these and other minerals have been removed by distillation is known as distilled water. ◼ The process of distillation also removes microbiological organisms, but volatile impurities such as CO,; chlorine, and ammonia are not removed. Water that has been distilled meets the specifications for type II and type III water. DOUBLE-DISTILLED WATER. ◼ Distilled or deionized water is not necessarily pure water. ◼ There may be contamination by dissolved gases, by nonvolatile substances carried over by steam in the distillation process, or by dissolved substances from storage containers. ◼ For example, in tests for nitrogen compounds (such as urea nitrogen, a common clinical chemistry determination), it is important to use ammonia-free (nitrogen-free) water. ◼ This may be specially purchased by the laboratory for such determinations or prepared in the laboratory by a specific method, double distillation, to remove the contaminating ammonia. DEIONIZED WATER ◼ In the process of deionization, water is passed through a resin column containing positively (+) and negatively (—) charged particles. These particles combine with ions present in the water to remove them; this water is known as deionized water. ◼ Only substances that can ionize will be removed in the process of deionization. Organic substances and other substances that do not ionize are not removed. ◼ Further treatment with membrane filtration and activated charcoal is necessary to remove organic impurities, particulate matter, and microorganisms to produce type I water from deionized water. WATER Combinations of Deionization and Distillation ◼ Water of higher purity is also produced by special distillation units in which the water is first deionized and then distilled, eliminating the need for double distillation. ◼ Other systems may first distill the water then deionize it. Reverse Osmosis ◼ The process of reverse osmosis passes water under pressure through a semipermeable membrane made of cellulose acetate or other materials. ◼ This treatment removes approximately 90% of dissolved solids, 98% of organic impurities, insoluble matter, and microbiological organisms. ◼ Reverse osmosis does not remove dissolved gases and removes only about 10% of ionized particles. OTHER PROCESSES OF PURIFICATION ◼ Other processes for water purification include ultrafiltration, ultraviolet oxidation, and sterilization (used after other purification processes), and ozone (used primarily in industrial settings). ◼ Filtration of water through semipermeable membranes will remove insoluble matter, pyrogens, and microorganisms if the pore size of the membrane is small enough. ◼ Adsorption by activated charcoal, clays, silicates, or metal oxides can remove organic matter. ◼ Type I water can be processed through a combination of deionization, filtration, and adsorption. OSMOLALITY & OSMOLARITY ◼ Osmolality and osmolarity are units of solute concentration that are referred to in the analysis of body fluids. ◼ An osmole is related to osmosis and is used in reference to solution where osmotic pressure is important, such as blood and urine. ◼ Both osmolality and osmolarity are defined in terms of osmoles. ◼ An osmole is a unit of measurement that describes the number of moles of a compound that contribute to the osmotic pressure of a chemical solution. OSMOLALITY ◼ Osmolality is defined as the number of osmoles of solute per kilogram of solvent. ◼ It is expressed as osmol/kg or Osm/kg. ◼ It is a more exact measurement of urine concentration than the urine specific gravity test. ◼ Urine osmolality is a measure of urine concentration based on the number of particles dissolved into the urine and is measured in milliosmols/kg. OSMOLALITY ◼ Osmolality is based on the number of dissolved particles in a solution. Osmolality measures the total concentration of all the ions and molecules present in serum or urine. ◼ Na+ glucose, and urea are major contributors to the total osmolality of serum. ◼ The reference range of osmolality for adults is 275 to 295 mOsm/kg. ◼ In contrast, in the calculation of the osmolal gap is the difference between the calculated osmolality and the measured osmolality. ◼ Elevation in the gap is usually caused by factors other than Na+ glucose, or blood urea nitrogen. Clinically, the presence of ketones or alcohol in the plasma can elevate the osmolal gap. OSMOLALITY ◼ Serum osmolality is primarily ordered to investigate hyponatremia, or low sodium in the blood. ◼ Hyponatremia may be caused by sodium loss through the urine or by increased fluid in the bloodstream. ◼ Increased fluid may be caused by drinking excessive amounts of water, water retention, decreased ability of the kidneys to produce urine, or the presence of osmotically active agents such as glucose. ◼ Marathon runners can be affected by hyponatremia. OSMOLALITY ◼ The average osmolal gap is 0 to 10 mOsm/kg H,O. Osmolality is important because it is the condition to which the hypothalamus responds. ◼ If a calculated osmolality is elevated above the reference range, the patient has dehydration. ◼ The regulation of osmolality affects the Na* concentration in plasma mainly because Na* and associated anions account for approximately 90% of the osmotic activity in plasma. REGULATION OF PLASMA NA+ The following three processes are important to the regulation of plasma Na+ concentration: ◼ Intake of H2O in response to thirst, which is stimulated or suppressed by plasma osmolality ◼ Excretion of H2O, which is primarily influenced by antidiuretic hormone (ADH) released in response to changes in either blood volume or osmolality ◼ Regulation of blood volume, which affects Na+ excretion through aldosterone, angiotensin II, and atrial natriuretic peptide OSMOLALITY CALCULATION ◼ https://www.youtube.com/watch?v=JqVeSdYD-LQ OSMOLALITY ELECTROLYTES ◼ Electrolytes are substances that form or exist as ions or charged particles when dissolved in H2O. ◼ Electrolytes are either negatively charged anions or positively charged cations. Cations move toward the cathode and anions toward the anode in an electrical field. ◼ Electrolytes include sodium (Na+), potassium (K+), calcium (Ca2+), magnesium (Mg2+), chloride (Cl), bicarbonate (HCO3), sulfate (SO2 ), and phosphate GROG): ELECTROLYTES ◼ The chief positively charged constituents (cations) are Na+ and K+. The chief negatively charged constituents (anions) are Cl and HCO3. Because Na+, K+, Cl, and HCO3, represent the major electrolytes, they are most likely to show variation when an electrolyte problem exists. ◼ Changes in the concentration of one are almost always accompanied by changes in the concentration of one or more of the others. Although these electrolytes are found throughout the body, their concentrations or activities vary from one body compartment to another. Assays are usually done on plasma or serum. ELECTROLYTES ◼ It is essential that the positively charged particles balance, or electrically neutralize, the negatively charged particles. ◼ The kidneys and lungs are the organs that exert the most control over electrolyte concentration. ◼ If the body is unable to maintain normal control over acceptable concentrations of electrolytes, either by excretion or conservation, an electrolyte imbalance occurs. ◼ Having an abnormal concentration of one or more of the electrolyte constituents is extremely harmful to the patient and can be fatal because most of the body’s essential metabolic processes are affected by or dependent on electrolyte balance. SODIUM ◼ Na+ is the major cation, or positively charged particle, and is found in the highest concentration in extracellular fluid. ◼ It is important in maintaining the osmotic pressure and in electrolyte balance. ◼ Na* is associated with the levels of Cl—and HCO3, ions and therefore has a major role in maintaining the acid-base balance of the body cells. ◼ A low serum Na+ level is called hyponatremia. Low Na+ levels are found in a variety of conditions, including severe polyuria, metabolic acidosis, Addison disease, diarrhea, and some renal tubular diseases. ◼ A high level of Na+ is called hypernatremia. Clinical conditions resulting in excess body Na+ include cardiac failure (congestive heart failure), liver disease (ascites), and renal disease (nephritic syndrome). SODIUM ◼ Clinical conditions resulting in excess body Na+ include cardiac failure (congestive heart failure), liver disease (ascites), and renal disease (nephritic syndrome). ◼ An increased Na+ level is found in Cushing syndrome, severe dehydration caused by primary H2O loss, certain types of brain injury, and diabetic coma after therapy with insulin, as well as after excess treatment with Na+ salts. ◼ The kidneys can conserve or excrete large concentrations of Na+ depending on the Na+ concentration of the extracellular fluids and the blood volume. POTASSIUM ◼ K+ is the major intracellular cation, but it is also found extracellularly. ◼ K+ has an important influence on the muscle activity of the heart. Hypokalemia (abnormally low K+ levels) can result from prolonged diarrhea or vomiting, or from inadequate intake of dietary K+. ◼ Even in conditions of K+ deficiency, the kidney continues to excrete K+. The body has no effective mechanism to protect itself from excessive loss of K+, so a regular daily intake of K+ is essential. POTASSIUM ◼ An elevated K+ level in serum is called hyperkalemia. ◼ K+ is primarily excreted by the kidney, it becomes elevated in kidney dysfunction or urinary obstruction. ◼ As with Na+, K+ is influenced by the presence of the adrenocortical hormones and is associated with acid-base balance. ◼ In renal tubular acidosis, there is increased retention of K+ in the serum. One important purpose of renal dialysis is the removal of accumulated K+ from the plasma. SODIUM AND POTASSIUM IN BODY FLUIDS ◼ Urinary Na+ excretion varies with dietary intake, but for individuals on an average diet containing 8 to 15 g/day, a range of 40 to 220 mmol/day is typical. ◼ Diurnal variation exists in Na+ excretion, with reduced levels at night. ◼ After K+ is absorbed by the gastrointestinal tract, it is rapidly distributed, with most excreted by the kidneys. ◼ K+ filtered through the glomeruli is reabsorbed almost completely in the proximal tubules and then secreted in the distal tubules in exchange for Na+ under the influence of aldosterone. SODIUM AND POTASSIUM IN BODY FLUIDS Factors that regulate distal tubular secretion of K* include the following: ◼ Na+ and K+ intake ◼ H2O flow rate in the distal tubules ◼ Plasma level of mineralocorticoids ◼ Acid-base balance ◼ Renal regulation of K+ excretion is influenced by renal tubular acidosis and by metabolic and respiratory acidosis and alkalosis. Retention of K+ is present in patients with chronic renal failure. SODIUM AND POTASSIUM IN BODY FLUIDS ◼ The Na+ concentration of CSF is 138 to 150mmol/L. ◼ Mean fecal Na+ excretion generally is considered to be less than 10mmol/day. ◼ In cases of severe diarrhea, fecal loss of K+ may be as much as 60 mmol/day. ◼ CSF values for K+ are approximately 70% of plasma values. ◼ Urinary excretion of K+ varies with dietary intake, but typically ranges from 25 to 125 mmol/day. CHLORIDE ◼ Cl is found in serum, plasma, CSF, tissue fluid, and urine. ◼ Physiologically, only the concentration of Cl in the extracellular fluid is important. ◼ The chief extracellular anions are Cl and HCO3, and there is a reciprocal relationship between them: a decrease in the amount of one produces an increase in the amount of the other. ◼ The CI ion is the most important anion of the extracellular fluids in the body. It is the major anion that counter balances the major cation, Na+. This means that the sum of all the cations equals the sum of all the anions. ◼ In the blood, two thirds of Cl is found in the plasma and one third in the RBCs. Because of the difference in Cl concentration between the RBCs and the plasma, the test for CI is routinely performed on plasma (or serum) and not on whole blood. CHLORIDE Cl has an important role in two main functions in the body: 1. determining the osmotic pressure, which controls the distribution of H2O among cells, plasma, and interstitial fluid 2. maintaining electrical neutrality ◼ CI plays an important role in the buffering action when carbon dioxide (CO 2) exchange takes place in the RBCs. This activity is known as the chloride shift. When blood is Oxygenated, CI travels from the RBCs to the plasma, and at the same time, HCO3 leaves the plasma and enters the RBCs. CHLORIDE ◼ Another important function of Cl is to regulate the fluid content of the body and its influence on the kidney. The kidney maintains the electrolyte concentration of the plasma within very narrow limits. ◼ Renal function is set to regulate the composition of the extracellular fluid first and then the volume. Consequently, if the body loses salt (NaCl), H2O is lost. ◼ High serum or plasma Cl values are seen in dehydration and conditions that cause decreased renal blood flow, such as congestive heart failure. CHLORIDE ◼ Excessive treatment with or dietary intake of Cl also results in high serum levels. Low serum or plasma Cl values may be seen when salt is lost, such as in chronic pyelonephritis. ◼ A low Cl value may also be seen in metabolic acidotic conditions that are caused by excessive production or diminished excretion of acids, such as diabetic acidosis and renal failure. ◼ Prolonged vomiting from any cause may ultimately result in a decrease in serum and body CI levels. BICARBONATE ◼ HCO3, after CI is the other major extracellular anion in body fluids. ◼ As the blood perfuse the lungs, CO2, and H2O are formed. During the metabolic processes, carbonic acid (H2CO3) dissociates and forms HCO3. This is a reversible reaction, and depending on body tissue requirements, HCO3 may be reconverted to H2CO3, followed by the formation of H2O and CO2. The reactions are: BICARBONATE ◼ HCO3 is filtered by the kidney, but little or no HCO3 is found in the urine. ◼ The proximal tubules reabsorb 85% of HCO3, and the remaining 15% is reabsorbed by the distal tubules. ◼ HCO3 is most often measured with other combined forms of CO2(CO2, HCO3, carbamino groups) as total CO2. Because about 90% of all the CO2 in serum is in the form of HCO3, this combined form approximates the actual HCO3 concentration very closely. ◼ Total CO2 is the total of H2CO3, dissolved CO2 gas, and HCO3. Assay methods for HCO3 are actually a measure of total CO2. BICARBONATE ◼ Along with pH and CO2 pressure (Pco2) determinations, the total CO2 concentration is a useful measurement in evaluating acid-base disorders. ◼ The HCO3 or CO2 value in itself is not as significant as the value in the context of the other electrolytes assayed. ◼ Assays for total CO2 are performed by using a Pco2 electrode to measure the rate of released CO2 being formed. ACID- BASE BALANCE ◼ https://www.youtube.com/watch?v=5_S5wZks9v8 ◼ https://www.youtube.com/watch?v=VzEEs00v-JU ANION GAP ◼ Anion gap is a calculation that measures the difference between the levels of positively and negatively charged electrolytes in your blood. It's a way to determine your blood's acid-base balance, or pH level, and check for electrolyte imbalances ◼ It is the calculation of the mathematical difference between the anions (Cl and HCO2) and the cations (Na+ and K+). ◼ If Cl and HCO2 are summed and subtracted from the sum of Na+ and K+ concentrations, the difference should be less than 16mmol/L, with a range of 10 to 20mmol/L. ANION GAP ◼ If the anion gap exceeds 16mmol/L, this is usually an indication of increased concentrations of the unmeasured anions (PO2 , SO2 , protein ions). ◼ Increased anion gaps can also result from ketotic states, lactic acidosis, salicylate and methanol ingestion, uremia, or increased plasma proteins. ◼ Decreased anion gaps of less than 10mmol/L can result from either an increase in unmeasured cations (Ca2+ , Mg2+ ) or a decrease in the unmeasured anions. ANION GAP ANION GAP ◼ The anion gap is also useful as a quality control measure for electrolyte results. ◼ If an increased anion gap is found for electrolytes in a healthy person, one or more of the test results may be erroneous, and the tests should be repeated. SPECIAL CONSIDERATIONS FOR SPECIMENS ◼ Plasma can be assayed for electrolyte concentration after use of lithium or sodium heparin as the anticoagulant, except in testing for Na+ in which case sodium heparin cannot be used. ◼ Electrolyte testing can also be done on serum. ◼ Capillary samples can be collected into microcontainers or capillary tubes. ◼ Centrifugation should be done using the unopened primary collection tubes, and the plasma or serum should be separated from the RBCs promptly. Each assay has specific requirements and technical factors relating to the specimen collection and handling. SPECIAL CONSIDERATIONS FOR SPECIMENS Sodium ◼ Lithium heparinized plasma, serum, urine, and other body fluids are suitable specimens. ◼ Na+ Sodium heparin should not be used, as already noted, because the presence of Na+ will interfere with the assay for Na+. ◼ Cells must be separated from serum or plasma as soon as possible. Na+ is stable in serum for at least 1 week at room or refrigerator temperature, or it can be frozen for up to 1 year. ◼ Na+ can be measured in 24-hour urine specimens and in CSF. SPECIAL CONSIDERATIONS FOR SPECIMENS Potassium ◼ Lithium or sodium heparin is the preferred anticoagulant for plasma specimens; an anticoagulant containing K+ cannot be used. Serum can also be tested. ◼ The collection of blood for K+ studies requires special attention and technique. Because the concentration of K+ in the RBC is about 20 times that in serum or plasma, hemolysis must be avoided. ◼ The following technical errors can contribute to elevated K+: 1. Recentrifugation of specimens in gel tubes 2. Inadequate centrifugation Centrifuging blood specimen tubes with the stoppers removed 3. Pouring blood from one tube to another 4. Delayed centrifugation - Refrigerating a specimen before K+ analysis 5. Improper venipuncture technique such as IV fluid contamination SPECIAL CONSIDERATIONS FOR SPECIMENS Potassium Con’t ◼ To avoid a shift of K+ from the RBCs to the plasma or serum, it is important to separate the cells from the plasma or serum within 3 hours of collection. ◼ When blood is collected for a K+ test, the patient should not open and close the fist before venipuncture; this muscle action can increase plasma K+ levels by 10% to 20%. K+ levels in plasma are about 0.1 to 0.2 mmol/L lower than those in serum because of the release of K+ from ruptured platelets during the coagulation process. ◼ K+ in serum promptly separated from the blood clot is stable for at least 1 week at room or refrigerator temperature. Specimens may be frozen for up to 1 year. K* levels in urine vary with dietary intake and are measured in a 24-hour collection. SPECIAL CONSIDERATIONS FOR SPECIMENS Chloride ◼ The anticoagulant used most frequently for Cl is lithium or sodium heparin. ◼ Serum separator gel tubes are also often used for specimens in Cl testing. ◼ Cl is assayed in serum, plasma, urine, or sweat, and in other body fluids. Moderate hemolysis does not significantly affect Cl concentration in the serum. SPECIAL CONSIDERATIONS FOR SPECIMENS Bicarbonate ◼ Lithium- or sodium-heparinized plasma or serum may be used for the HCO, assay. Arterial blood is generally collected. ◼ The pH and HCO3 concentrations are most accurately determined immediately when the tube is opened and as quickly as possible after collection and centrifugation of the unopened tube. ◼ A specimen to be assayed for total CO2 must be handled anaerobically to minimize losses of CO2 and HCO3(converted to CO2) into the atmosphere. ◼ A falsely low total CO2 would result if this loss has occurred. In the laboratory, the specimen can be protected by placing a stopper on the container. METHODS FOR QUANTITATIVE MEASUREMENT ◼ Four electrolytes—Na+, K+, Cl- and HCO3—are generally grouped together for testing, called an electrolyte profile. ◼ Ion-selective electrode (ISE) potentiometry uses a glass ion exchange membrane for Na+ assay and a valinomycin neutral-carrier membrane for K* assay and has been incorporated into many automated chemistry analyzers. ◼ ISE methods measure the activity of an ion in the H2O volume fraction in which it is dissolved. ISE ◼ Generally, two types of ISE measurements are made on biological samples: 1. Direct 2. Indirect ◼ Direct measurement is done on undiluted samples; indirect measurement requires pre-diluted samples for measurement of ion. ◼ Lipemia and protein cause a false decrease in indirect ISE measurements because they occupy plasma volume. REFERENCE VALUES ◼ Sodium: 136-142 mmol/L ◼ Potassium: 3.5-5.1 mmol/L ◼ Chloride: 98-107 mmol/L ◼ Bicarbonate: 22-29mmol/L EASYLYTE & I-STAT ANALYZERS ◼ https://www.youtube.com/watch?v=2HsMxzOsDIE ◼ https://www.youtube.com/watch?v=iVF6jWM-vYg SUMMARY ◼ Water of the highest purity will vary with the method of preparation. ◼ Electrolytes are substances that form or exist as ions or charged particles when dissolved in H2O. ◼ Osmolality is defined as the number of osmoles of solute per kilogram of solvent. ◼ Na+ is the major cation, or positively charged particle, and is found in the highest concentration in extracellular fluid. ◼ K+ is the major intracellular cation, but it is also found extracellularly. ◼ Cl is found in serum, plasma, CSF, tissue fluid, and urine ◼ HCO3, after CI, is the other major extracellular anion in body fluids. ◼ The anion gap is also useful as a quality control measure for electrolyte results. The End ◼ Questions or Clarifications?