Control of Gene Expression – Ch 33 PDF
Document Details
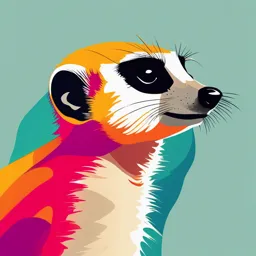
Uploaded by EffectualJubilation
UMC
2023
Dr. Maryam Syed
Tags
Related
- Lecture 14a: General Principles of Gene Expression Regulation in E. Coli PDF
- Chapter 29: Transcription and the Regulation of Gene Expression PDF
- Gene Expression Regulation: Lecture Notes PDF
- BIOCHEMISTRY REGULATION OF GENE EXPRESSION & MUTATIONS PDF
- Gene Expression Biochemistry BUCM 2024-2025 PDF
- Molecular Biology And Genetics Lecture Notes PDF
Summary
This presentation details control of gene expression, covering topics like the lac operon, the trp operon, and eukaryotic gene regulation. It includes concepts from prokaryotic and eukaryotic organisms.
Full Transcript
Control of Gene Expression – Ch 33 CMB 704/DENT 604 – Fundamental Biochemistry Dr. Maryam Syed [email protected] Fall 2023 1 Learning Objectives • Explain the role of the lac operon and its regulatory elements in lactose metabolism under the 3 different conditions (lac +, glu -; lac -, glu+; lac +,...
Control of Gene Expression – Ch 33 CMB 704/DENT 604 – Fundamental Biochemistry Dr. Maryam Syed [email protected] Fall 2023 1 Learning Objectives • Explain the role of the lac operon and its regulatory elements in lactose metabolism under the 3 different conditions (lac +, glu -; lac -, glu+; lac +, glu+). Include the roles of allolactose, CAP, and cAMP. • Describe the role of the Stringent Factor in the Stringent Response. • Explain the regulation of the trp operon under the 3 different conditions (trp high, trp high and initiates transcription, trp low). Include the role of trp as a corepressor and the role of attenuation. • Identify the three structural motifs proteins use to bind to the DNA. • Explain the role of STF in coordinate regulation using the example of the Galactose circuit in Yeast. • Explain the role of intracellular receptors in gene regulation using the example of the glucocorticoid receptor. • Describe the role of mRNA editing in the production of the apo-B-48 protein. • Explain the role of mRNA stability in iron metabolism. • Describe how miRNAs function as posttranscriptional regulators of gene expression. • Explain the role of acetylation and methylation of the DNA in terms of access to the DNA or gene regulation. 2 Regulation of gene expression • DNA RNA = primary site of regulation (Transcription) • Steps regulated in eukaryotes to control when and which gene products produced: 1. modifications to access regions of DNA 2. posttranscriptional modifications 3. posttranslational modifications 3 Regulation of gene expression • Not all genes are tightly regulated • Constitutive genes = expressed at constant levels products required for basic cellular functions • “housekeeping genes” • i.e. ribosomes • • Regulated genes: • only expressed when needed • expressed in all cells or subset of cells • i.e. hepatocytes 4 Regulation of gene expression • All cells have the same DNA that codes for all genes • Gene regulation determines: 1. What gene product is made? How much product is made? 3. When gene product is made? 4. Where gene product is made? 2. 5 Regulatory Sequences and Molecules 6 Regulatory sequences and molecules • Transcription is controlled by regulatory sequences of DNA embedded in noncoding region of genome • i.e. Enhancer and Silencer • Induce or repress transcription machinery • Influence what and how much product is made 7 Regulatory sequences and molecules • Regulatory DNA sequences are cis-acting • • Regulatory molecules are trans-acting • • • Influence expression of genes on same chromosome Travel from site of synthesis to DNA-binding site i.e. TF produced from gene on chromosome 11, acts on genes in chromosome 6 Proteins bind DNA through structural motifs in protein: 1. 2. 3. Zinc finger Leucine zipper Helix-turn-helix 8 Regulation of Prokaryotic Gene Expression 1. mRNA transcription from bacterial operons 2. Operators in bacterial operons 3. Lactose operon 4. Tryptophan operon 5. Coordination of transcription and translation 9 Regulation of prokaryotic gene expression • Regulation occurs primarily at level of transcription • Mediated by binding of trans-acting proteins to cis-acting regulatory elements • Regulation of gene expression at transcription = saved energy • Transcriptional control: 1. Initiation of transcription 2. Premature termination of transcription 10 Messenger RNA transcription from bacterial operons • Structural genes that encode a protein involved in the same pathway are sequentially grouped on the same chromosome • Includes cis-acting element (promoter) • Allows coordinated regulation of genes • Genes are all turned “on” or “off” as a unit • Coordinated unit = operon 11 Operators in bacterial operons • Bacterial operons contain an operator • Operator: • Sequence on DNA • Regulates structural gene activity • Binding site for repressor protein • 3 possible Scenarios: 1. Operator site free 2. Operator site bound by repressor 3. Operator site bound by repressor + repressor bound by inducer 12 Operators in bacterial operons Scenario 1 Operator site free RNA pol: 1. Binds promoter 2. Passes operator 3. Reaches protein-coding gene 4. Transcribes DNA to mRNA 13 Operators in bacterial operons Scenario 2: Operator site bound by repressor RNA pol: 1. Binds promoter 2. Blocked at operator 3. No mRNA produced no proteins produced 14 Operators in bacterial operons Scenario 3: Operator site bound by repressor + repressor bound by inducer 1. Repressor changes shape 2. Repressor not bound to operator site 3. RNA pol passes operator site 4. RNA pol initiates transcription 15 Lactose (Lac) operon in E. coli • Involved in catabolism of lactose • Contains genes for 3 proteins 1. 2. 3. • lacZ gene: β-galactosidase hydrolyzes lactose to galactose + glucose lacY gene: permease moves lactose into the cell lacA gene: thiogalactoside trasacetylase acetylates lactose Genes max produced only when lactose is present and glucose is absent • Glucose is preferred fuel source for bacteria 16 Lactose (Lac) operon in E. coli • Regulatory elements are upstream of 3 structural genes 1. Promoter region binds RNA pol Operator (O) binds regulatory proteins 3. Catabolite activator protein (CAP) binds regulatory proteins 2. 17 Lactose (Lac) operon in E. coli Scenario A: lactose is absent and glucose is present • Promoter site RNA pol not bound • O site bound by lacI (repressor protein) • CAP site empty • Outcome: Lac operon repressed (turned off) 18 19 Lactose (Lac) operon in E. coli Scenario B: lactose is present and glucose is absent 1. Some lactose converted to allolactose (inducer) 2. Allolactose binds lacI (repressor) lacI conformation change cannot bind O site 3. Adenylyl cyclase active cAMP produced binds CAP • • • • Promoter site bound by RNA pol O site empty CAP site bound by complex of cAMP + CAP/CRP Outcome: Max production of 3 proteins lactose used for energy production 20 21 Lactose (Lac) operon in E. coli Scenario C: lactose is present and glucose is present • Adenylyl cyclase inhibited in presence of glucose no cAMP-CAP complex Promoter site ineffective binding of RNA pol • O site empty • CAP site empty • • Outcome: Lac operon uninduced; very low expression of 3 proteins 22 23 Tryptophan (trp) operon in E. coli • Involved in synthesis of aa tryptophan • Contains 5 structural genes • Subject to negative control • Trp is a corepressor • Also regulated by attenuation when repression is incomplete 24 Tryptophan (trp) operon in E. coli Attenuation • Transcription initiated BUT terminated before completion • Attenuator = hairpin structure in mRNA • Similar MOA as rho-independent termination • Transcriptional attenuation occurs in prokaryotes only • mRNA translation begins before mRNA synthesis is complete 25 Tryptophan (trp) operon in E. coli 1. Trp levels high • Operator bound by repressor + repressor bound by Trp • Outcome: operon repressed 26 Tryptophan (trp) operon in E. coli 2. Trp levels high BUT transcription is initiated (escaped repression) • Operator bound by repressor + repressor bound by Trp • Attenuator (hairpin in mRNA) forms • Outcome: attenuation activated and translation terminated before completion 27 Tryptophan (trp) operon in E. coli 3. Trp levels very low • Operator empty • 5’-end of mRNA contains trp codon ribosome stalls ribosomes cover region that forms the attenuator attenuator cannot form • Outcome: operon expressed 28 Tryptophan (trp) operon in E. coli 29 Coordination of transcription and translation Stringent response = regulation in response to amino acid starvation • E. coli has 7 operons to synthesize rRNA • • Binding of uncharged tRNA to ribosome A-site production of guanosine tetraphosphate (ppGpp) • • Regulated in response to environmental conditions synthesized by stringent factor (RelA), (enzyme associated with ribosomes) Elevated levels of ppGpp selective inhibition of RNA synthesis 30 Regulation of Eukaryotic Gene Expression 1. Coordinate regulation 2. mRNA processing and use 3. Regulations through variations in DNA 31 Regulation of eukaryotic gene expression • Higher complexity + nuclear membrane = more regulatory processes • Transcription is primary site of regulation • Multiple levels of regulation • Trans-acting factors bind cis-acting elements • No operons 32 Coordinate regulation How do you coordinate regulation of group of genes for a specific response when genes are located on different chromosomes? • Specific transcription factor (STF) • • • A trans-acting protein binds to cis-acting regulatory element on each gene in the group even if they are on different chromosomes STF have DNA-binding domain (DBD) and a transcription activation domain (TAD) • TAD recruits coactivators (i.e. HAT + GTF) and RNA pol to form transcription initiation complex at promoter 33 Galactose circuit • Regulatory scheme allows use of galactose when glucose is not present • Yeast genes to metabolize galactose on different chromosomes • Coordinated expression mediated by Gal4 (STF protein) • Gal4 binds upstream of each gene at upstream activating sequence (UASGal) 34 Galactose circuit Gal4 binds to UASGal in absence and presence of galactose Galactose absent A. 1. 2. Galactose present B. 1. 2. 3. • Gal80 (regulatory protein) binds Gal4 at TAD Gene transcription inhibited Galactose activates Gal3 protein Gal3 binds Gal80 Gal4 activates transcription Glucose inhibits expression of Gal4 cell cannot metabolize galactose 35 Hormone response system • Hormone response elements (HRE) = • DNA sequences that bind trans-acting proteins • regulate gene expression in response to hormonal signals • Hormones bind to 1. intracellular (nuclear) receptors 2. cell-surface receptors 36 Hormone response system Intracellular receptors • Members of the nuclear receptor superfamily function as STFs • Steroid hormones, vitamin D, retinoic acid, and thyroid hormone receptors • DBD + TAD + ligand-binding domain 37 Hormone response system Intracellular receptors 1. Hormone cortisol binds intracellular glucocorticoid receptors at ligandbinding domain 2. Conformational change in receptor receptor activated 3. Receptor–hormone complex enters nucleus, dimerizes, and binds to glucocorticoid response element (GRE) on DNA 4. Binding recruits coactivators to TAD 5. Expression of cortisol-responsive genes 38 Messenger RNA processing and use 1. Capping at 5’-end 2. Polyadenylation at 3’-end 3. Splicing • Messenger stability and variations in splicing and polyadenylation can affect gene expression 39 Messenger RNA editing • Posttranscriptional modification base in mRNA altered Apolipoprotein B (apoB) • Essential component of chylomicrons and VLDL • Made in liver and small intestine • Intestine C in CAA codon changed to U aa codon changed to stop codon UAA • Shorter protein produced apoB-48 40 Messenger RNA stability • Time mRNA is in cytosol before being degraded influences how much protein it can produce • mRNA stability important in the regulation of gene expression: 1. Iron metabolism 2. RNA interference (miRNAs) 41 Messenger RNA stability Iron metabolism • Transferrin (Tf): plasma protein that transports iron • Tf binds cell-surface TfR that is internalized to provide cells with iron • mRNA for TfR has cis-acting iron-responsive elements (IRE) in 3′-UTR • IRE have short stem-loop structure that can bind trans-acting iron regulatory proteins (IRP) 42 Messenger RNA stability Iron metabolism • [Iron] in cell is low: 1. IRP bind to the 3′-IRE 2. Stabilize the mRNA for TfR 3. ↑ TfR synthesis 43 Messenger RNA stability Iron metabolism • [Iron] in cell is high: 1. IRP dissociate from 3′-IRE 2. mRNA for TfR unstable and degraded 3. ↓ TfR synthesis 44 Messenger RNA stability RNA interference (RNAi) • Mechanism of gene silencing through decreased expression of mRNA 1. Repression of translation 2. Increased degradation of mRNA • Mediated by short (~22 nucleotides), noncoding RNA called microRNA (miRNA) 45 Messenger RNA stability RNA interference (RNAi) • miRNA transcribed from DNA pri-miRNA • Pri-miRNA processed by Drosha (endonuclease) pre-miRNA • Pre-miRNA exported to cytoplasm • Processed by Dicer (endonuclease) double-stranded miRNA • One miRNA strand (guide) associates with RISC • miRNA-RISC binds with complementary sequence of target mRNA • Target mRNA is translationally repressed or degraded 46 Regulation through variations in DNA Access to DNA • DNA complexed with histones to form chromatin • Transcriptionally active, decondensed chromatin (euchromatin) vs. condensed, inactive form (heterochromatin) 47 Regulation through variations in DNA Access to DNA • Histones covalently modified at amino terminal ends by reversible: 1. 2. Acetylation • Decreases positive charge on histones decreases association with negatively charged DNA relaxes nucleosome allows transcription factors to access regions on DNA Methylation 48 Regulation through variations in DNA Access to DNA • Methylation of C in CG-rich regions (CpG islands) in the promoter region 1. Transcriptionally active genes less methylated (hypomethylated) 2. Transcriptionally inactive genes more methylated (hypermethylated) 49 Summary • Prokaryotic gene expression is primarily regulated at the level of initiation of gene transcription. In general, there can be multiple proteins per gene (polycistronic). • Sets of genes that encode proteins with related functions are organized into operons. • Each operon is under the control of a single promoter. • Repressors bind to the promoter to inhibit RNA polymerase binding. • Inducers bind to the repressor to facilitate RNA polymerase binding. 50 Summary • Eukaryotic gene regulation occurs at several levels. • • • • • • • • At the DNA structural level, chromatin must be remodeled to allow access for RNA polymerase, which is accomplished, in part, by proteins with histone acetyltransferase activity. Transcription is regulated by transcription factors that either enhance or restrict RNA polymerase access to the promoter. Transcription factors have one functional domain for DNA binding and one for transcription activation. Transcription factors can be classified according to the structure of their DNA-binding domains; these include zinc finger, helix-turn-helix, and leucine zipper. Transcription factors can bind to promoter-proximal elements, certain response elements, or enhancer regions which are a great distance from the promoter. Coactivators (mediator proteins) bind to the transactivation domains of transcription factors to enhance assembly of the basal transcription complex. Specific transcription factors increase the transcription of a gene when bound to enhancers and other response elements. They are also important for coordinate gene regulation of related genes located on different chromosomes. RNA processing (including alternative splicing), transport from the nucleus to the cytoplasm, and translation are also regulated in eukaryotes. MicroRNA expression alters translation of expressed mRNAs. 51