Gene Expression 2: RNA Translation and Genetic Code PDF
Document Details
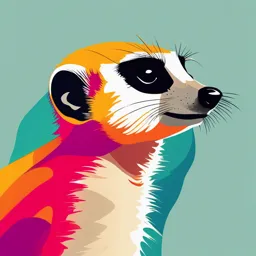
Uploaded by SpiritualHonor
College of Medicine
Tags
Related
- Lecture 14a: General Principles of Gene Expression Regulation in E. Coli PDF
- Gene Expression Regulation: Lecture Notes PDF
- BIOCHEMISTRY REGULATION OF GENE EXPRESSION & MUTATIONS PDF
- Gene Expression Biochemistry BUCM 2024-2025 PDF
- Molecular Biology And Genetics Lecture Notes PDF
- Regulation Of Gene Expression 2022-2023 PDF
Summary
This document discusses the rules of the genetic code, the function of different types of RNA in translation, and how translation plays a role in health and disease.
Full Transcript
17 GENE EXPRESSION 2. RNA TRANSLATION AND GENETIC CODE ILOs By the end of this lecture, students will be able to 1. Discuss the rules of genetic code 2. Correlate the function of different RNAs to the process of translation 3. Describe the process of translation 4. Interpret r...
17 GENE EXPRESSION 2. RNA TRANSLATION AND GENETIC CODE ILOs By the end of this lecture, students will be able to 1. Discuss the rules of genetic code 2. Correlate the function of different RNAs to the process of translation 3. Describe the process of translation 4. Interpret role of translation and post translational modification in health and disease What is translation? It is the translation of the nucleotide sequence of a mRNA (Codons) into an amino acid sequence of a protein, in order to synthesize proteins. Each codon consists of a sequence of 3 nucleotides i.e. it is a triplet code. Collection of these codons makes up the genetic code. Protein biosynthesis is called translation because it involves translation of information from the 4- letter language and structure of nucleic acid into the 20-letter language and structure of proteins Requirements of translational process m RNA as a carrier of genetic information. tRNA as an adapter molecule, which recognizes an amino acid on one end and its corresponding codon on the other end. At least one specific type of tRNA is required for each amino acid. Ribosomes as the molecular machine coordinating the interaction between mRNA, tRNA, the enzymes and the protein factors required for protein synthesis. Genetic code (Figure 1) It is the relationship between nucleotide sequence in DNA or mRNA AND amino acids in a polypeptide chain. Each amino acid can be specified by more than one codon. There is one start codon: AUG (METHIONINE) There are 3 STOP codons (UAA, UAG, UGA) The genetic information along mRNA is read from 5’ to 3’ direction. Figure 1.genetic code 1 Characteristics of genetic code 1- Genetic code is degenerate i.e. multiple codons can code for the same amino acid except tryptophan and methionine.(both are coded by only one codon) Wobble theory: The 3rd nucleotide in a codon is less important than the other two in determining the specific amino acid to be incorporated. (i.e if an amino acid has several codons, all such cosons will usually have the first 2 letters in common but the third is different, which means the 3 rd is of less importance) 2- Genetic code is unambiguous i.e. each codon specifies no more than one amino acid. 3- Genetic code is non-overlapping and Commaless meaning that the code is read from a fixed starting point as a continuous sequence of bases, taken three at a time without any punctuation between codons. For example, AGCUGGAUACAU is read as AGC UGG AUA CAU. 4-Genetic code is universal i.e. the same code words are used in all organisms (pro- and eukaryotes) Protein Biosynthesis stages 1- Initiation 2- Elongation 3- Termination Stage 1: Initiation For initiation of protein biosynthesis, there must be:- - tRNA - rRNA - mRNA - Eukaryotic initiation factors (eIFs). - GTP, ATP and different amino acids. In this stage, The 80 S eukaryotic ribosome is dissociated into 40 S and 60 S subunits. eIF – 3 and eIF-1 bind to 40 S subunit thus preventing re-association between the 2 subunits. GTP and eIF-2 bind, in addition to binding of mRNA (accompanied by hydrolysis of ATP to ADP+Pi)and Methionine– tRNA (a tRNA specifically involved in binding to the initiation codon AUG). This This is followed by re-association of both ribosomal units, with dissociation of initiation factors and hydrolysis of GTP. This is termed the initiation complex. (Can you enumerate its components?) (Figure2) Figure 2. Initiation of translation 2 N.B: t RNA charging (Figure 3) It means recognition and attachment of the specific amino acid to the 3` hydroxyl adenosine terminus (to the sugar) of tRNA in an ester linkage. Figure 3.tRNA charging Stage 2: Elongation It is a cyclic process involving 3 steps I. Binding of aminoacyl – tRNA to the A site The ribosome has three binding sites for tRNA molecules: the A, P, and E sites.(Figure 4) In the complete 80S ribosome subunit, A site is free (N.B. A=aminoacyl binding site) Binding of aminoacyl t-RNA to A site needs activation of aminoacyl tRNA by binding of eukaryote elongation factor - 1 (e EF-1) and GTP. When aminoacyl tRNA binds to A site, GTP is hydrolysed and e EF-1 is released. Figure 4..Binding sites of tRNA in ribosome N.B: Anticodon: Each tRNA molecule contains a three-base nucleotide sequence, the anticodon, which pairs with a specific codon on the mRNA within the ribosome. This codon specifies which aminoacid will be inserted in the growing peptide sequence. The first codon on mRNA always codes for methionine. II. Peptide bond formation (Figure 5) The alpha amino group of the new amino acid carried by t-RNA in the A site attacks the carboxylic group of the peptidyl-tRNA in the P site. This reaction needs peptidyl transferase enzyme (RIBOZYME) The reaction results in attachment of the growing peptide chain to the tRNA in the A site. III. Translocation (Figure 5) Upon removal of the peptide from t-RNA in the P site, the discharged t-RNA quickly dissociates. eEF-2 and GTP are responsible for translocation of the newly formed peptidy t-RNA from A site to P site 3 The A site is now free to receive a new aminoacyl-RNA Stage 3: Termination (Figure 6) After many cycles of elongation, the non-sense or stop codon of mRNA (UAA, UAG or UGA) appears in the A site. Normally, there is no tRNA with an anticodon capable of recognizing such a termination signal. Releasing factors (eRFS) can recognize the termination signals in the A site Releasing factors(eRFs), GTP and peptidyl transferase promote the hydrolysis of the bond between the peptide chain and t-RNA at P site 80S subunit dissociates and all the factors , tRNA , mRNA, GDP and Pi are released Figure 5. Process of elongation Figure 6.Termination of translation N.B. The formation of one peptide bond requires energy resulting from hydrolysis of 4 high energy phosphate bonds:- Charging of tRNA with amino acyl moiety requires hydrolysis of an ATP to an AMP. (2 high energy bonds) The entry of amino tRNA into the A site requires one GTP hydrolysis to GDP. The translocation of the newly formed peptidyl – tRNA in the A site into the P site results in hydrolysis of one GTP to GDP N.B. Errors in translation will result in faulty proteins, which will be either targeted for degradation in proteasome or will be non- functioning or abnormally functioning. Protein maturation Aim: Activation of protein to a functional form 4 Localization in subcellular compartment Secretion from the cell Protein maturation involves the following: I- Protein folding: Folding to 3D structures, aided by molecular chaperone. (Refer to protein structure lecture). Misfolded proteins are targeted for destruction II- Post – translational processing: a- Proteolysis: It means removal of amino terminal, carboxy terminal or internal sequences. Examples: 1-Removal of amino terminal methionine residues 2-Removal of signal peptides by signal peptidases (signal peptides help translocate the proteins to its final destination and hence are removed after protein transport) (figure 7) b- Modifications of individual amino acids Figure 7.Proteolysis Hydroxylation: of proline and lysine for collagen synthesis Phosphorylation of serine, threonine or tyrosine (Important in cell signaling) γ-carboxylation of glutamic acid in prothrombin and osteocalcin (this helps Ca 2+ binding which is necessary for blood clotting and bone ossification in both proteins respectively) c- Addition of certain groups Glycosylation: Attachment of CHO side chain to form glycoproteins. Glycosylation can help stabilize glycoproteins against degradation or provide proper conformation for protein function. CHO can be either N linked( to asparagine), or O-linked( to serine and threonine) Acylation: Addition of fatty acids to various amino acid side chains. Acylation has several functional effects on proteins, especially to help anchor them to membranes. Clinical implications: I) Many antibiotic work by altering the translation of bacterial DNA and are generally classified as bacterial protein synthesis inhibitors as Tetracyclines and Macrolides like Erythromycin. II) Some toxins can cause death by inhibiting eukaryotic translation: Shiga toxin / ricin (produced by E-coli bacteria and causes bloody diarrhea) inhibits tRNA binding by acting on 60S subunit Diphtheria toxin (produced by bacteria and causes difficult breathing, heart failure, paralysis, and even death) inhibits translocation through binding to Eukaryotic- EF-2 5 19 GENETIC VARIATIONS AND MUTATIONS ILOs By the end of this lecture, students will be able to 1. Recognize the different types of mutation. 2. Predict the effect of types of mutation on amino acid sequence and protein structure. 3. Correlate the phenotypic outcome with type of mutation What is meant by mutations? Mutations are permanent changes in a DNA sequence. This altered DNA sequence can be reflected by changes in the base sequence of mRNA, and, sometimes, by changes in the amino acid sequence of a protein. Mutations can cause genetic diseases. Types of mutation I- Point mutation (single base substitution): (Figure 1) This entails the substitution of the original base in the gene by another. This can take either of two forms: 1- Transition: In which one purine is replaced by another purine or one pyrimidine is replaced by another pyrimidine. e.g. A >>>G or C>>>T 2- Transversion: In which a purine is replaced by a pyrimidine or a pyrimidine is replaced by a purine. e.g. T>>>> A, C >>>> A, T>>>>> G, C>>>> G Figure 1. point mutation Single-base substitutions may have no physiologic effect if they occur in a DNA region that is not part of the coding or regulatory regions of a gene. Mutations may alter regulatory sequences, eg, in promoter or enhancer regions, which can affect gene expression. Single-base changes that occur within a coding region of a gene may produce disease alleles Consequences of point mutation (Figure 2) 1- Silent mutation: The codon containing the changed base may code for the same amino acid. 1 For example, if the serine codon UCA is given a different third base (to become, say, UCU), it still codes for serine. This usually happens if the mutation happens in the 3 rd codon.(refer to wobble’s theory in translation lecture) Therefore, this is termed a “silent” mutation without any effect on protein structure. 2. Missense mutation: (Figure 2) The codon containing the changed base may code for a different amino acid. The substitution of an incorrect amino acid may result in variable effects on protein structure (e.g. hemoglobin β- chain). This type of mutation can result in one of the following: a- Acceptable missense mutation AAA>>>>>>>>> AAU (codons) Lysine>>>>>>>> Asparagine (amino acid) 61 This produces apparently normal functional hemoglobin. b- Partially acceptable missense mutation GAA>>>>>>>>>> GUA (codons) Figure 2. Consequences of point mutation Glutamic acid>>> Valine (amino acid) 6 This produces Hb S; it can bind and release O2 although abnormal, but doesn’t function in low O2 saturation. C- Unacceptable missense mutation: CAU>>>>>>>> UAU (codons) Histidine >>>>> Tyrosine (amino acid) 58 This produces Hb M; it cannot transport O2 and the only treatment is repeated blood transfusions. Clinical implications Hereditary hemochromatosis (HH) is one of the most common genetic diseases. It is associated with two well-known missense mutations in the HFE gene (Human homeostatic iron regulator protein). These mutations are used to screen ‘‘at-risk populations’’ for this disorder of iron metabolism, which results in liver damage (cirrhosis), diabetes, skin pigmentation, and heart failure. (refer to cardiovascular module) 2 3) Non-sense mutations The codon containing the changed base may become a termination codon. For example, if the Serine codon UCA is changed at the second base and becomes UAA, the new codon causes premature termination of translation at that point and the production of a shortened (truncated) protein II) Frame shift mutation: (Figure3) It results from deletion (removal) or insertion (addition) of one or more nucleotides in DNA that generates altered m RNAs with different effects on protein structure. The insertion or deletion results in shifting in the way the codons are read, the thing that produces totally different aminoacids. Figure 3. Frameshift mutations III) Trinucleotide repeat expansion: (Figure 4) Occasionally, a sequence of three bases that is repeated in tandem will become amplified in number so that too many copies of the triplet occur. If this happens within the coding region of a gene, the protein will contain many extra copies of one amino acid. For example, expansion of the CAG codon in exon 1 of the gene for Huntington protein leads to the insertion of many extra glutamine residues in the protein, causing the neurodegenerative disorder Huntington disease. The additional glutamines result in an abnormally long protein that is cleaved, producing toxic fragments that aggregate in neurons. 3 Also, Fragile X syndrome, the most common cause of mental disability in males results from a similar mechanism Figure 4.Trinucleotide repeat expansion IV). Splice site mutations: Mutations at splice sites can alter the way in which introns are removed from pre-mRNA molecules, producing abnormal proteins. Gene silencing can result from splicing alterations leading to lack of protein production. Polymorphisms A polymorphism is a change in genotype that can result in no change in phenotype or a change in phenotype that is harmless, causes increased susceptibility to a disease, or, rarely, causes the disease. It is traditionally defined as a sequence variation at a given locus (allele) in >1% of a population. Polymorphisms primarily occur in the 98% of the genome that does not encode proteins (that is, in introns and intergenic regions). Types (Figure 4) 1. Single-base changes: About 90% of human genome variation comes in the form of single nucleotide polymorphisms (SNPs, pronounced “snips”), that is, variations that involve just one base. 2. Tandem repeats: Polymorphisms in chromosomal DNA can also arise from the presence of a variable number of tandem repeats (VNTR). These are short sequences of DNA at scattered locations in the genome, repeated in tandem (one after another). The number of these repeat units varies from person to person but is unique for any given individual and, therefore, serves as a molecular “fingerprint.” Clinical implications Paternity tests (to prove the parenthood of a father to a baby) rely on fingerprinting (VNTR) Polymorphisms can increase the susceptibility to diseases such as cancer and cardiovascular diseases. Polymorphisms can be used for screening people at high risk of certain diseases 4 Figure 4. Types of polymorphism 5 20 REGULATION OF GENE EXPRESSION & ITS MODIFICATION ILOs By the end of this lecture, students will be able to 1. Deduce different mechanisms of regulation of gene expression 2. Correlate how intracellular signaling molecules affect gene expression 3. Outline how drugs can be used to modify gene expression & nuclear signaling What is meant by regulation of gene expression? It is the control on the amount of protein that is being expressed (transcription and translation) from the genetic DNA. Types of gene expression 1- Constitutive gene expression: It is unvarying expression of a gene (i.e. expressed at all times). It is responsible for expression of House Keeping genes, which are needed to maintain viability of cell (e.g. genes expressing glycolysis enzymes). 2- Regulated gene expression: It is the expression of genes whose products (proteins) level changes in response to molecular signals (for example consumption of nutrients, stress, infection…etc).They can be inducible genes or repressible genes. ▪ Inducible genes: They are the genes whose products increase in concentration under particular molecular circumstances, i.e.; positive regulation. ▪ Repressible genes: They are the genes whose products decrease in concentration in response to molecular signals. Regulation of gene expression If you remember the sequence of central dogma of life, you can deduce that regulation of gene expression can occur at different levels: I) At the level of chromatin (Chromatin remodeling) ▪ In eukaryotes, DNA is found complexed with histone and nonhistone proteins to form chromatin. Transcriptionally active, decondensed chromatin (euchromatin) differs from the more condensed, inactive form (heterochromatin) in a number of ways: 1- Active chromatin contains histone proteins that have been covalently modified at their amino terminal ends by reversible acetylation, or phosphorylation. Such modifications increase the negative charge of histones, thereby decreasing the strength of their association with negatively charged DNA. This relaxes the nucleosome, allowing transcription factors access to specific regions on the DNA and hence more protein expression. The opposite is true. Page 1 of 5 2-Methylation can occur on cytosine bases in CG-rich regions (CpG islands) in the promoter region of many genes. Transcriptionally active genes are less methylated (hypomethylated) than their inactive counterparts, suggesting that DNA hypermethylation silences gene expression. Clinical implication Some drug can affect gene expression by inducing DNA methylation or histone modification as the use of histone deacetylase inhibitors (HDACi); to kill cancer cells by inducing cell cycle II) At the level of DNA (genes) 1) Gene copy number: ▪ An increase or decrease in the number of copies of a gene can affect the amount of gene product produced. ▪ An increase in copy number (gene amplification) can lead to increased gene expression. An example of this is amplification of the gene coding for the enzyme dihydrofolate reductase (DHFR) (required for the synthesis of thymidine triphosphate (TTP), which is essential for DNA synthesis), leading to increased production of the enzyme. ▪ Another example is gene deletion, with the famous example of RBCs. During development of RBCs, immature erythroblasts contain nuclei that produce mRNA for synthesis of the globin chain of hemoglobin. As the cells maturate, the nuclei are extruded, so that the fully mature red blood cells have no genes, so they can no longer produce mRNA and proteins. Clinical implication Gene amplification is the mechanism by which many tumour cells develop resistance to anticancer drugs. E.g., The anticancer drug, methotrexate, acts by inhibiting the enzyme dihydrofolate reductase. Amplification of DHFR genes by cancer cells makes them less responsive (resistant) to methotrexate. 2. Re-arrangement of DNA: A single gene of immunoglobulins (Antibodies) can produce from 10 9−1011 different immunoglobulins, providing the diversity needed for the recognition of an enormous number of antigens. This diversity is due to the process of DNA rearrangement. The chains of Igs contain segments called constant (C ), variable (V), diversity (D), and joining (J). Each time an IG is produced, re-arrangement of different segments (Except the constant segment) occur to produce a different Ig. (Figure 1) Figure 1.Gene re-arrangement to produce different Igs Page 2 of 5 3. Mobile DNA elements (Transposons (Tn): These are mobile segments of DNA that move in a random manner from one site to another on the same or a different chromosome. Movement is mediated by transposase, an enzyme encoded by the Tn itself. Transposition has contributed to structural variation in the genome and the potential to alter gene expression and even to cause disease. III) At the level of transcription For transcription factors to work, they have to interact in harmony with DNA sequences acting as regulatory regions as follows: 1- Basal expression elements: (Figure 2) It consists of the proximal element of TATA box that direct RNA polymerase II to the correct start site(+1) and the upstream element e.g. CAAT box or GC box that specify the frequency of initiation. Figure 2.Basal expression elements 2-Regulated expression elements: (Cis-acting elements): They are specific DNA sequences that are present on the same gene, so termed Cis-elements, and are responsible for regulation of expression. They can exert their effect on transcription even when separated by thousands of base pairs from a promoter. They include Enhancers and Silencers (Refer to gene expression 1: transcription lecture Regulatory proteins Activators and inhibitors (called trans- factors as they are produced by other genes than this being transcribed (Refer to gene expression 1: transcription lecture )(Figure 3) Figure 3.Interaction between basal and regulated expression elements Page 3 of 5 3- Response elements They are sequences on DNA to which signaling molecules bind, causing change in gene expression. a. Signaling molecules binding to intracellular receptors Members of the nuclear receptor superfamily include the steroid hormone (glucocorticoids, mineralocorticoids, androgens, and estrogens), vitamin D, retinoic acid, and thyroid hormone receptors. These molecules, in addition to some metals such as iron, have intracellular receptors (either cytoplasmic or nuclear) When such molecules diffuse inside the cell, they bind to their receptors. The receptor-ligand complex translocates to DNA to bind to its specific response element, which is a pre-defined sequence on DNA. (for e.g hormone response element (HRE), or metal response element). This binding can alter rate of expression of certain genes (for example, binding of steroid to steroid response element, causes increased expression of gluconeogenesis enzymes, binding of vitamin D to its response element causes increased expression of calcium binding protein) b. Signaling molecules binding to cell surface receptors These receptors include those for insulin, epinephrine, and glucagon. This extracellular signal is then transduced to intracellular 2nd messengers to end by phosphorylation and activation of different kinases. One of these is cAMP response element–binding [CREB] protein that can bind to a specific responsive element sequence on DNA and result in transcription of some target genes of metabolism or of growth. Clinical implications: Pharmacological modulation of certain gene transcription is a common mechanism of action of many classes of drugs either: A) Directly by targeting nuclear receptor superfamily (that are ligand activated transcriptional factors), where the drug receptor complex, itself induces activation or suppression of gene transcription. For e.g., all steroid hormones, as Glucocorticoids or related steroid drugs; dexamethasone, are used in treatment of many inflammatory and autoimmune disorders by suppressing the transcription of inflammatory mediators and cytokines. B) Indirectly by targeting cell surface receptors to affect their downstream signalling cascades to finally affect their kinases involved in activation e or inhibit of transcriptional factors. For e.g., Insulin (targeting tyrosine kinase receptors) used in treatment of diabetes mellitus by affecting the genes involved in metabolism. C) Indirectly by interacting with enzymes that activate or inhibit transcriptional factors. For e.g., Calcineurin inhibitors, cyclosporine, inhibit calcineurin to inhibit transcription factors involved in transcription of a cytokine mediator (IL-2) so can be used to suppress increased immune activity during treatment of many autoimmune disorders and in graft rejection. (Refer to cytokines lecture) Page 4 of 5 III-Post-Transcriptional Regulation: Regulation can occur during processing of the primary transcript (hn RNA) and during the transport of mRNA from nucleus to the cytoplasm. 1- Alternative splicing and polyadenylation sites In certain cases, the use of alternative splicing and polyadenylation sites causes different proteins to be produced from the same gene. For example, in parafollicular cells of the thyroid gland, the calcitonin gene produces mRNA that codes for calcitonin. In the brain, the transcript of this gene undergoes alternative splicing and polyadenylation to produce a different protein called calcitonin gene-related protein (CGRP). 2-RNA editing It is change in a single nucleotide on mRNA after it has been transcribed. The nucleotide is changed either by insertion, deletion or substitution. An example of RNA editing occurs in the production of β apoprotein (apoβ) that is synthesized in liver and intestinal cells and serves as a component of the lipoproteins (carriers of lipid in circulation). Although these apoproteins are encoded by the same gene, the version of the protein made in the liver (B-100) contains 4563 amino acid residues, while the (B-48) made in intestinal cells has only 2152 amino acid. This is due to RNA editing in intestinal cells results in a change of a single nucleotide causing the creation of a stop codon earlier than usual and hence a shorter protein. 3-Stability of mRNA Developmental or environmental stimuli like nutrient levels, cytokines, hormones and temperature shifts as well as environmental stresses like hypoxia, hypocalcemia, viral infection, and tissue injury affect stability of mRNA and hence amount of protein produced In addition, a group of RNAs called microRNA act as post-transcriptional regulators of their mRNA causing mRNA degradation and/or translational repression. IV- Regulation at the level of Translation: Most eukaryotic translational controls affect the initiation of protein synthesis. The initiation factors for translation, particularly eukaryotic initiation factor 2 (eIF2), are the focus of these regulatory mechanisms. The action of eIF2 can be inhibited by phosphorylation. V- Post-Translational Regulation: After proteins are synthesized, their lifespan is regulated by proteolytic degradation. Proteins have different half-lives, some last for hours or days, others last for months or years. Some proteins are degraded by lysosomal enzymes, other proteins are degraded by proteases in the cytoplasm. Clinical Implications: Post translationally, many drugs can affect certain protein level. For e.g., Protease inhibitors; antivirals that inhibit post-translational processing of precursor viral proteins and is used in treatment of HIV and Hepatitis C infection. Page 5 of 5 22 Cytosolic Respiration : ILOs By the end of this lecture, students will be able to 1. Correlate carbohydrate intake to production of energy 2. Deduce how energy production differs among different cells and different cellular compartments 3. Correlate regulation of glycolysis to energy production ❖ What is Glycolysis? And why is it important? - Is a sequence of reactions occuring in the cytoplasm for the oxidation of Glucose to two molecules of pyruvic acid (3-carbon molecule) under aerobic conditions; or lactate under anaerobic conditions providing energy (as ATP) and intermediates for other metabolic pathways. - The unique ability to function in presence or absence of oxygen makes glycolysis the only source of energy in RBCs (as they lack mitochondria) and when performing physically- demanding tasks, the anaerobic glycolysis serves as the primary energy source for the muscles. - The glycolytic pathway may be considered as the preliminary step before complete oxidation. - It provides carbon skeletons for amino acid synthesis and the glycerol portion of fat. - It occurs as follows: Page 1 of 4 Net gain of 8 ATP produced Under anaerobic conditions NADH+H+ (at step 6) is re oxidized via lactate formation. This allows glycolysis to proceed in the absence of oxygen. Page 2 of 4 Clinical Implications: Pyruvate kinase deficiency: As mature RBCs are completely dependent on glycolysis for ATP production. Pyruvate kinase deficiency leads to decreased ATP production. This results in hemolytic anemia, with the severe form requiring regular transfusions. Severity depends both on the degree of enzyme deficiency. ❖ Regulation of the Glycolytic Pathway The regulatory enzymes of the glycolytic pathway are the 3 irreversible enzymes: hexokinase, phosphofructokinase (PFK-1), and pyruvate kinase (PK). A- Hormonal regulation: 1- Covalent Modification: - Insulin secreted in fed state activates key enzymes of glycolysis by dephosphorylation. - Glucagon secreted in fasting inhibits key enzymes of glycolysis by phosphorylation. 2- Induction/Repression: - Insulin leads to induction of key enzymes of glycolysis while glucagon represses them B- Allosteric Regulation: Page 3 of 4 Inhibitors of glycolysis: 1. Mercury inhibits glyceraldehyde-3-P dehydrogenase by binding to the enzyme’s active site. This will inhibit glycolysis to proceed leading to cell death. The most common cause of mercury poisoning is from eating polluted or wrongly preserved seafood. 2. Fluoride combines with Mg2+ as Mg fluoride, Mg is essential for the activity of enolase enzyme, therefore fluoride interferes with enolase activity. For this reason fluoride has been used for years as a rodenticide (to kill rodents) and a pesticide. It also explains why the FDA requires that all fluoride toothpastes should carry a warning that If more than used for brushing is accidentally swallowed, medical help should be seeked right away. ❖ What happens after the 2 pyruvates are produced? Pyruvate produced from glycolysis (under aerobic conditions) is then transported to the mitochondria via a special transporter where it is converted to Acetyl Co-A by “Pyruvate Dehydrogenase Complex”. (PDH) (see Aerobic VS Anaerobic). Page 4 of 4 24 Aerobic VS Anaerobic respiration : ILOs By the end of this lecture, students will be able to 1. Clarify conditions and end products of aerobic vs anaerobic respiration. 2. Discuss organs and cells utilizing each type of respiration 3. Describe the shuttles linking cytosolic and mitochondrial energy production - As we discussed before, glycolysis pathway is unique in that it occurs in both aerobic and anaerobic conditions. But definitely there are many differences between glycolysis occurring in aerobic and anaerobic cells. To know these differences we should answer the following questions ❖ Which cells utilize the aerobic respiration and which utilize the anaerobic? - Anaerobic respiration occurs in tissues that are poorly perfused either under normal conditions for example, the lens and cornea of the eye and the kidney medulla, or due to pathological causes. - Exercising muscles: During exercise, demand for oxygen by working muscle increases in proportion to the level of work performed. This results in a higher demand to deliver necessary oxygen to these active tissues; the oxygen supplied is not enough for these active cells therefore they utilize anaerobic respiration - Cells lacking mitochondria as RBCs utilize anaerobic respiration even in presence of good oxygen supply. - Under normal conditions in the presence of good oxygen supply all other tissues utilize aerobic respiration. ❖ What happens if glycolysis occurs in cells utilizing aerobic respiration? - If glycolysis occurs under aerobic conditions the 2 NADH+H+ molecules produced by the action of glyceraldehyde 3 phosphate dehydrogenase will be shuttled to the inner mitochondrial membranes where they will be fully oxidized producing 3 ATP each. - The net energy production from aerobic glycolysis will therefore be 8 ATP. - The 2 Pyruvate molecules produced by the end step of glycolysis by the action of pyruvate kinase enzyme will then be transported to the mitochondria via a special transporter where it is converted to Acetyl Co-A - The conversion of pyruvate to acetyl CoA is an irreversible reaction performed by the action of “Pyruvate Dehydrogenase Complex” (PDH). - The Pyruvate Dehydrogenase Complex is actually 3 enzymes complexed together responsible for the combined dehydrogenation and decarboxylation (oxidative decarboxylation) of pyruvate to acetyl-CoA (which enters the CAC). - For this enzyme complex to function it needs the action of five different coenzymes which are: Page 1 of 5 - Thiamine pyrophosphate (TPP) - Lipoic Acid - Coenzyme A - Flavin adenine dinucleotide (FAD) - Nicotinamide adenine dinucleotide (NAD). Regulation of Pyruvate Dehydrogenase: Allosteric Inhibition by elevated acetyl CoA and NADH+H Covalent modification: dephosphorylated form is the active one by the action of insulin Mercury can inhibit PDH. Arsenic poisoning is due to inhibition of lipoic acid which is essential for PDH action. Clinical implications: Pyruvate dehydrogenase deficiency is a rare condition with severe health effects. Therefore, many affected individuals do not survive past childhood. However, alteration in the action of PDH is more commonly due deficiencies of thiamine (TPP) or niacin (NAD) these conditions are usually due to severe nutritional deficiencies or alcohol abuse The condition is characterized by lactic acidosis, which can cause severe respiratory problems. It also affects the neurological and mental ability since the brain cells are unable to produce sufficient ATP (via the CAC cycle) if the PDH activity is decreased. - Therefore, under aerobic conditions complete glucose oxidation can produce up to 38 ATP, 8 ATP from aerobic glycolysis, 6 ATP from the 2 NADH+H+ produced by PDH enzyme complex acting on 2 pyruvates and 24 ATP from oxidation of 2 Acetyl CoA in CAC. - Another fate for Pyruvate produced by glycolysis under aerobic conditions might be Carboxylation of pyruvate to oxaloacetate by pyruvate carboxylase - Pyruvate carboxylase is a biotin-dependent reaction. This irreversible reaction is important because it replenishes the CAC cycle intermediate Page 2 of 5 Clinical Implications: Biotin deficiency (vitamin B7) even in mild cases would affect the energy production by the cells leading to several manifestations including hair loss, thin nails, conjunctivitis or even some neurological manifestations. Biotin deficiency usually occurs due to dietary absence of the vitamin or consuming raw egg whites over months. ❖ What if glycolysis reactions occurred in anaerobic cells? - In this case glycolysis will produce only 2 ATP molecules and the 2 pyruvate molecules produced will not be converted to acetyl CoA alternatively they will be converted to Lactate by the action of Lactate Dehydrogenase enzyme (LDH) in a reversible reaction. - N.B: NADH produced by glycolysis will be used up by the LDH enzyme, there will be no energy production from NADH oxidation in ETC. Therefore, the net energy production in anaerobic respiration is only 2 ATP molecules. - Lactate formation in muscle: In exercising skeletal muscle, NADH production by glycolysis exceeds the oxidative capacity of the electron transport chain (ETC). This results in an elevated NADH/NAD+ ratio, favoring reduction of pyruvate to lactate by LDH. - Therefore, during intense exercise, lactate accumulates in muscle, causing a drop in the intracellular pH, potentially resulting in cramps. - The lactic acid then enters “Cori cycle”: Lactate which is formed during anaerobic oxidation of glucose in muscles and in RBCs diffuses to the blood then to the liver. In the liver lactate is oxidized to pyruvate (as the reaction is reversible), which can be converted to glucose again. Glucose goes back to tissues and is reutilized for production of energy. Page 3 of 5 Clinical Implications: Lactic acidosis: Elevated concentrations of lactate in the plasma, termed lactic acidosis, occur when there is generalized decrease in tissue perfusion, such as with severe hypotension, myocardial infarction and hemorrhage, or when an individual is in shock. The failure to bring adequate amounts of Oxygen to the tissues results in impaired oxidative phosphorylation and decreased ATP synthesis. To survive, the cells rely on anaerobic glycolysis for generating ATP, with accumulation of lactic acid as the end product. This will lead to fast, shallow breathing, disorientation, a general feeling of discomfort, muscle pain or cramping, and unusual sleepiness, tiredness, or weakness. The onset of lactic acidosis might be rapid and occur within minutes or hours, or gradual, happening over a period of days. If left untreated “lactic acidosis” can result in severe and life-threatening complications. ❖ How is NADH+H produced in the cytoplasm transported to the mitochondria? - The inner mitochondrial membrane lacks a NADH transporter, and NADH produced in the cytosol cannot directly enter the mitochondrial matrix. - However, NADH molecules are transported from the cytosol to the mitochondria using substrate shuttles. - In the glycerol 3-phosphate shuttle: NADH used to convert dihydroxyacetone phosphate by cytosolic glycerol 3-phosphate dehydrogenase glycerol 3-phosphate. The glycerol 3-phosphate can pass to the mitochondria where it is converted back to DHAP but producing FADH2 instead of the utilized NADH. Therefore, the glycerol 3-phosphate shuttle results in the synthesis of two ATP for each cytosolic NADH oxidized. Page 4 of 5 - This contrasts with the malate shuttle, in which oxaloacetate uses NADH+H to be converted to malate. Malate passes to the mitochondria where it is reconverted to oxaloacetate producing NADH+H, thereby yielding three ATP for each cytosolic NADH oxidized. - Both shuttles move molecules from cytoplasm to mitochondria but not the opposite. Page 5 of 5 25 Mitochondrial Electron Transport System ILOs By the end of this lecture, students will be able to 1. Describe components and mechanism of mitochondrial electron transport system 2. Deduce why mitochondrial pathways produce more energy than glycolytic ones 3. Correlate the inhibitors of mitochondrial electron transport to serious health conditions 4. Predict the effect of defects in electron transport system What is mitochondrial electron transport chain (ETC)? It is final common pathway in aerobic cells (that contain mitochondria) by which electrons derived from various compounds (NADH+H+ and FADH2) are transferred to O2 to form H2O. This is associated with formation of energy in the form of ATP. Principle of energy formation through the ETC The metabolic intermediates of oxidation of nutrients ( e.g oxidation of glucose and fatty acids) donate electrons to specific coenzymes, nicotinamide adenine dinucleotide (NAD+) and flavin adenine dinucleotide (FAD), to form the energy-rich reduced forms, NADH+H+ and FADH2. (The hydrogen atom is formed of a proton and an electron) These reduced coenzymes can, in turn, each donate a pair of electrons to a specialized set of electron carriers, collectively called the electron transport chain (ETC). As electrons are passed down the ETC, they lose much of their free energy. This energy is used to move H+ (protons) across the inner mitochondrial membrane (a process called proton pump) creating a H+ gradient in the inter-membranous space. Accumulated protons will create an electrochemical gradient, and thus will flow inside the mitochondria along their concentration gradient, through a channel in a protein complex called ATP synthase. This drives the production of ATP from ADP and inorganic phosphate (Pi). (Mitchell’s Chemiosmotic Theory). The coupling of electron transport with ATP synthesis is called oxidative phosphorylation, sometimes denoted as OXPHOS. It proceeds continuously in all tissues that contain mitochondria. The aim of this manner of stepwise oxidation is to use energy in ATP synthesis, otherwise it would be lost as heat. ATP is used in active transport, enzymatic reactions and most synthetic processes. To sum up, energy production through the ETC occurs in 3 steps: 1-Electron transport between different complexes (oxidation). 2-Proton pumping into the inter-membranous mitochondrial space. 3-Synthesis of ATP from ADP and Pi as a source of energy (Phosphorylation). 1 Components of ETC (Figure 1) The ETC consists of a series of 4 protein complexes spanning the inner mitochondrial membrane, in addition to 2 other compounds (coenzyme Q and cytochrome C). All protein complexes of ETC contain transitional metals that can oscillate between a reduced state and an oxidized one (e.g iron and copper). Thus, protein complexes have the potential to receive and donate electrons. At the end of the protein series is a 5 th complex called ATP synthase, which spans the mitochondrial membrane and helps in synthesis of ATP. Complex I: (NADH-: ubiquinone oxidoreductase, or NADH dehydrogenase.) It is formed of : ⮚ Many polypeptides ⮚ FMN coenzyme ⮚ Seven –iron sulfur centers (FeS) which are necessary for the transfer of electrons to the next member of the chain (coenzyme Q). As mentioned previously, NADH+H+ resulting from different oxidative reactions, enters the mitochondria through shuttles. Electrons or hydrogens are transferred from NADH to FMN then to different FeS centers and finally to ubiquinone (UQ) to form reduced UQH2. 4 protons are pumped during electron transport Complex II: (Succinate: ubiquinone oxidoreductase or succinate dehydrogenase) It is formed of: ⮚ Succinate dehydrogenase. ⮚ FAD coenzyme ⮚ Two iron sulfur centers. It catalyzes the transfer of electrons or hydrogens from FADH2 released by oxidation of succinate(by succinate dehydrogenase) to UQ to form UQH2. No protons are pumped at this complex Complex III: (Ubiquinone : ferricytochrome C oxidoreductase.) It is formed of: ⮚ One FeS center ⮚ Cytochrome b ⮚ Cytochrome C1 It transfers electrons from UQ-H2 to cytochrome C. 2 protons are pumped during electron transport Complex IV: (Ferrocytochrome C: oxygen oxidoreductase). It is formed of: ⮚ Two cytochromes (a &a3) 2 ⮚ Two atoms of copper It transfers electrons from cytochrome C to oxygen which then combines with the two H+ to form H2O. 4 protons are pumped during electron transport N.B: Cytochromes, each contain a heme group made of a porphyrin ring containing an atom of iron. The cytochrome iron atom is reversibly converted from its Fe+2 to Fe+3 as a normal part of its function as a reversible carrier of electrons. *Ubiquinone (Coenzyme Q: CoQ) It is made from an intermediate of cholesterol synthesis. It is called ubiquinone because it is ubiquitous (abundant) in biologic systems. It links the flavoprotein dehydrogenases to the cytochromes. It transfers electron from both complexes I and II to complex III *Cytochrome c It is called the mobile element of ETC. It is located in the intermembrane space, loosely associated with the outer face of the inner membrane. Electrons are passed along the chain from cytochrome bc1 (Complex III), to cytochrome c, and then to cytochromes a + a3 (Complex IV) N.B: To ensure flow of electrons from the beginning to the end of ETC, components of the ETC are arranged according to their oxidation reduction potential from higher to lower. (Can you deduce how?) Coupling sites for oxidative phosphorylation & ATP synthesis These are the sites at which proton pumping occurs Coupling site I: lies between FMN(in complex I) & CO Q Coupling site II: lies between cytochrome b & c1 (in complex III) Coupling site III: lies at cytochromes a and a3 (in complex IV) 3 Figure 1.Components of ETC Clinical implication A drawback of ETC is the potential leakage of electrons to outside of the mitochondria and formation of reactive oxygen species, that have deleterious effects on all body molecules and, if in abundance, can cause diseases such as cancer. ATP synthesis (Figure 2) A 5th protein complex called ATP synthase spans the inner mitochondrial membrane. It is formed of two subunits. Accumulated protons in the mitochondrial intermembranous space will flow according to their concentration gradient through a channel in the ATP synthase. Proton flow is accompanied by binding ADP to inorganic phosphate (Pi) to form ATP. ATP flows outside the mitochondria to go to other organelles and cells that require it, in exchange with ADP through ATP/ADP antiporter, which requires no energy as it is along the concentration gradient. Formation of 1 molecule of ATP requires 4 protons. (Can you deduce why oxidation via NAD-linked dehydrogenase produces 3 ATP while that oxidized via a flavoprotein- linked dehydrogenase produces only 2 ATP?) 4 Figure 2. ATP synthase Control of Respiratory Chain. The respiratory chain is controlled by the levels of ADP and Pi as well as by the availability of ADP/ATP transporter. Inhibitors of the respiratory chain. A) Inhibitors of the respiratory chain proper. They inhibit the electron transport and thus the proton pumping. i.e Complete inhibition of the ETC. Examples include: i)Barbiturates (a medicine used to induce sleep),ii) piericidin A (antibiotic), iii)rotenone (rat poison), iv)antimycin (antifungal), v)carbon monoxide (CO) (gas leakage from gas heaters), and vi)cyanide (poison). This explains death in case of accidental or attempted (suicide) ingestion of overdose of these drugs and gases. B) Inhibitors of oxidative phosphorylation. They inhibit the transport of ADP into and the transport of ATP out of the mitochondria. Examples include Oligomycin (Antibiotic) and Atractyloside (poison present in some plants). C) Uncouplers of oxidative phosphorylation. They dissociate oxidation in the respiratory chain from phosphorylation. This means that electron transport continues, while pumped protons flow through channels other than that of ATP synthase. As a result, oxidation becomes unlimited since it is not controlled by the concentration of ADP or Pi. Most energy is released in the form of heat instead of forming ATP. Examples include Thyroxin, Ca2+, large doses of aspirin and uncoupling protein (UCP), which is present in large amounts in brown adipose tissue and 2,4 dinitrophenol (fertilizer). This explains the generation of heat when ingestion of large doses of these compounds, or in individuals and organisms with high content of brown adipose 5 tissue (which contains uncoupler protein-1) Clinical implications Inherited defects in oxidative phosphorylation Defects in oxidative phosphorylation are more likely a result of alterations in mitochondrial DNA, which has a mutation rate about 10 times greater than that of nuclear DNA. Tissues with the greatest ATP requirement (for example, the central nervous system, skeletal and heart muscle, and the liver) are most affected by defects in oxidative phosphorylation. Such diseases are usually accompanied by vision loss and mental retardation. 6