Magnetic Resonance Principles PDF
Document Details
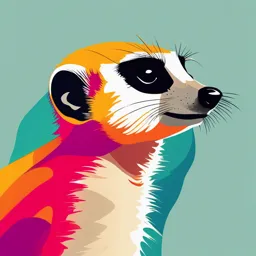
Uploaded by WillingPixie4700
Vinschool Grand Park
Tags
Summary
This document provides an introduction to nuclear magnetic resonance (NMR) and how it is used in magnetic resonance imaging (MRI). It discusses the interaction of atomic nuclei with magnetic fields, focusing on hydrogen atoms for MRI applications. The key concept of resonance is highlighted as crucial in generating the MR signal.
Full Transcript
Introduction to Nuclear Magnetic Resonance (NMR) Nuclear magnetic resonance (NMR) is a technique that uses magnetic fields and does not rely on ionizing radiation. Magnetic Field Interaction: NMR works by detecting the interaction of atomic nuclei (especially hydrogen) with an...
Introduction to Nuclear Magnetic Resonance (NMR) Nuclear magnetic resonance (NMR) is a technique that uses magnetic fields and does not rely on ionizing radiation. Magnetic Field Interaction: NMR works by detecting the interaction of atomic nuclei (especially hydrogen) with an external magnetic field. Comparison with Other Imaging: ○ Unlike CT or ultrasound, NMR imaging provides unique information. ○ Magnetic Resonance Imaging (MRI) differs from transmission tomographic images by showing molecular and chemical details. Hydrogen Atoms: ○ MRI primarily focuses on hydrogen atoms. ○ It captures how the configuration and chemistry of hydrogen influence the NMR signal. Applications:MRI creates clear images of soft tissues by using the principles of nuclear magnetic resonance (NMR), offering insights into body structures unavailable in CT or ultrasound imaging. Magnetic Field Originally “magnetism”. A magnetic field is a region around a magnet or a current-carrying wire where magnetic forces can be observed. Key Points 1. Origin of Magnetism: ○ Initially referred to natural or man-made magnets and their interactions, independent of electricity. 2. Discovery by Hans Oersted (1820): ○ Found that an electric current in a wire deflects a compass needle (causes the compass needle to move or change direction), proving the link between electricity and magnetism. 3. Earth as a Bar Magnet: ○ Earth acts like a large bar magnet with magnetic poles near its North and South Poles. ○ A magnetic field causes moving charges or magnets to experience force, e.g., compass needle deflection. Units of Magnetic Field (B): Tesla (T): SI unit for magnetic field strength. Gauss: cgs unit for measuring magnetic fields. ○ 1 gauss = 10^−4T = 0.1 mT. Earth’s Magnetic Field: Ranges from 0.25 to 0.65 gauss = 25 to 65 T. Magnetic fields are crucial in MRI as they align the nuclei for imaging. Nuclear Spin (otto stern gerlach) and Magnetic Moment Nuclear Spin: Protons have a property called "nuclear spin" due to their subnuclear structure. Protons (+ charge) and neutrons (neutral) both have intrinsic spins of S=1/2 Magnetic Moment: The magnetic moment (μ) of a particle is related to its spin (S) by the equation: μ=γS where γ is the gyromagnetic constant, specific to each particle. Nuclear Magnetic Moment: Since the proton is in the nucleus, its magnetic dipole moment is called the nuclear magnetic moment. Magnetic moment, 𝜇⃗ , is related to spin 𝑆⃗ : 𝜇⃗ = 𝛾𝑆⃗ In short, nuclear spin creates a magnetic moment, which is a key property in magnetic resonance. Magnetization (M) Random Orientation: In a sample, the magnetic moments of nuclei are usually randomly oriented. Alignment in Magnetic Field: When placed in a strong magnetic field (B0), the moments align slightly more parallel or antiparallel to the field. Magnetization: The total vector sum of these magnetic moments is called magnetization (M). Axes: ○ The z-axis (longitudinal axis) aligns with B0. ○ The x and y axes make up the transverse plane. The Proton in a Magnetic Field Magnetic Moment Interaction: Nuclei with a magnetic moment, when placed in a strong magnetic field, interact with the field and may undergo nuclear magnetic resonance (NMR). Common Nucleus in MRI: In MRI, hydrogen nuclei (protons) are most commonly used because hydrogen has only a proton and an electron. Nuclear Spin Precession Precession: When a nucleus' magnetic moment is not aligned with the applied magnetic field, it precesses (or rotates) around the magnetic field. In short, protons interact with magnetic fields and, if not aligned, rotate around the field in a motion called precession. Resonance Resonance occurs when a system oscillates at its natural or resonance frequency. This frequency depends on the system and its components. Example: Like pushing a swing at the right moment to increase its motion (the swing’s natural frequency), a small push at each cycle increases the amplitude, causing resonance. At Resonance The system has its highest energy. Resonance in MR Imaging In MR Imaging: Resonance frequency is crucial. The system (e.g., protons in the body) needs to be taken into resonance to create images. This means the protons’ natural frequency matches the applied magnetic field to produce a signal that can be captured for imaging. The Larmor Frequency (or Resonance Frequency) Definition: The Larmor frequency (or resonance frequency) is the processional frequency, w0, of a nucleus in a magnetic field, B0. It is directly proportional to the magnetic field strength and the gyromagnetic ratio, g. Formula: The relationship is given by the Larmor equation. Importance: This frequency is essential for generating the MRI signal. NMR Signal - Stage 1: Equilibrium System Setup: consider a system of protons in a magnetic field B0, is in equilibrium Magnetization: The magnetization M points along the z-axis. Magnetization Components: ○ Mz is at its maximum, called M0. ○ Mxy is zero. Stage 2: Applying the RF Pulse RF Pulse: A small magnetic field B1 is applied in the transverse plane (90° to the main magnetic field B0). RF Frequency: Radiofrequency (RF) EM waves oscillate at MHz frequencies. Stage 3: Magnetization Tips Away Effect of RF Pulse: The RF pulse tips the magnetization away from the z-axis at an angle α\alpha (flip angle). Precession: After tipping, the magnetization starts to precess around the magnetic field. Signal Generation: As precession occurs, Mz decreases, and Mxy increases. The NMR signal strength depends on the size of Mxy.. Stage 4: Return to Equilibrium Relaxation: After the RF pulse is turned off, the magnetization returns to its equilibrium state. Equilibrium State: Mz goes back to maximum (M0) and Mxy returns to zero. Stage 5: Inducing an Electric Current Precessing Magnetization: As the magnetization precesses, it changes the magnetic flux (magnetic field lines). Faraday’s Law: This changing magnetic flux induces an electric current in a coil. Signal Detection: The induced current is picked up by a coil/loop of wire and measured. NMR Signal and FID (Free Induction Decay) Primary NMR Signal: is the signal measured in the time domain as an oscillating, decaying electric signal induced by the magnetization in free precession, known as FID free induction decay. Free induction decay (FID) is a time-based electrical signal that is detected in a nuclear magnetic resonance (NMR) spectrometer after a radio-frequency (rf) pulse. RF Coil: An RF coil is placed around the sample to transmit an RF pulse. The RF pulse frequency is close to the sample's Larmor frequency. Precession: The RF pulse causes the magnetization in the sample to precess (rotate), creating a varying magnetic flux in the RF coil. Induced Current: According to Faraday’s law, the changing magnetic flux induces a current or voltage in the RF coil, generating the NMR signal. Signal Processing: The NMR signal is processed by a computer to create an image or spectrum of the sample's composition. RF Pulse Influence: The RF pulse will only influence the magnetization M if it oscillates at the Larmor frequency. RF Coil Function: The RF coil both transmits the RF pulse and receives the NMR signal. Note that for higher Larmor frequencies, higher-power RF pulses are required to influence the magnetization in the nuclei. Chemical Shift The precise resonance (Larmor) frequency of a proton is determined by its local magnetic field. Shielding Effects: of the Electrons orbitals around the proton can influence the local magnetic field. This is called shielding (denoted as σ). ○ Formula: B= B0(1−σ) Small changes in the Larmor frequency or chemical shifts can be represented in a spectrum (nuclear magnetic resonance spectroscopy). It is measured in ppm (parts per million) and Common range for physiological molecules: 0 ppm < δ < 10 ppm. Example: If a free hydrogen proton has a Larmor frequency of 42.6 MHz at 1 T, hydrogen in water or fat will have a different Larmor frequency due to shielding effects. Application: Chemical shifts are important in nuclear magnetic resonance spectroscopy and are widely used in chemistry and material sciences. Chemical Shift for Water: 4.7ppm = 4.7x 10^6 Chemical Shift for fat: 3.5 ppm In this diagram chemical shift, or change in Larmor frequency is shown for the hydrogens in water versus those in fat (lipid). Sources of Image Contrast Relaxation Times: Key factors affecting image contrast, including T1, T2, and T2*. Flip Angle: due to the B1 RF pulse. Affects contrast by influencing how magnetization is tipped by the RF pulse. Larmor Frequency: Determines the resonance frequency, contributing to contrast. Relaxation Times Relaxation Times: The decay constants for magnetization depend on factors like tissue type, magnetic field, and environment of nuclei. T1 (Longitudinal Relaxation Time): ○ Also known as spin-lattice relaxation time. ○ Governs the exponential growth of the longitudinal magnetization (Mz). ○ Measures the rate at which energy is transferred from protons to surrounding molecules (the lattice). T2 (Transverse Relaxation Time): ○ Also called spin-spin relaxation time. ○ Governs the exponential decay of transverse magnetization (Mxy). ○ Measures how energy decays in the transverse plane without transferring it to neighboring molecules. T2* Transverse Relaxation (T2) happens faster than expected due to various processes involved in creating it. Magnetic field inhomogeneities (irregularities) cause distortions, leading to the use of T2* as the relaxation time, which is shorter than T2. T2* is often approximated as an exponential decay, although it may not strictly follow an exponential pattern. Relaxation Time Constants Relaxation times (T1, T2, T2*) depend on tissue molecular structure, chemistry, and magnetic field strength. Differences between normal and abnormal tissues can change T1 and T2 T2* values. Flip Angle The flip angle (α\alpha) depends on the magnitude of the RF pulse (B1) and the RF pulse duration (tRF). γ\gamma is the gyromagnetic ratio depends on the nucleus being studied. The flip angle can be controlled by changing the pulse time or pulse amplitude (B1). Pulse Sequences One thing that makes MRI such a good imaging method is that the subtle differences in tissue chemistry can be thoroughly explored by exposing them to different series of RF pulses and gradient pulses of different sequences, strength and time intervals. A physicist can provide a series of RF pulses and gradient pulses (called a pulse sequence) to cause the contrast in the image to depend on T1, T2, T2*, “proton” density or many other tissue characteristics. Commonly Used Pulse Sequences 1. Saturation Recovery Sequence ○ Used to measure T1 relaxation time by saturating the magnetization before recovery. 2. Spin-Echo Sequence ○ Measures T2 relaxation. ○ Uses an RF pulse and a 180° pulse to refocus spins and correct for magnetic field inhomogeneities. 3. Gradient Echo Sequence ○ Measures T2* relaxation. ○ Uses gradient pulses instead of 180° pulses, sensitive to field inhomogeneities. 4. Inversion Recovery Sequence ○ Enhances contrast based on T1. ○ Applies a 180° pulse to invert magnetization before measuring recovery.