Bio Module 1-2-2 PDF
Document Details
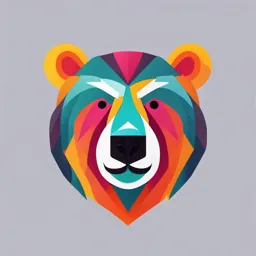
Uploaded by LionheartedCantor
Toronto Metropolitan University
Tags
Related
- Cellular Level of Organization 1 Lecture PDF
- Eukaryotic Cell - Cell Membrane PDF
- Fiche 3 : La membrane plasmique (partie 1) - Biologie Cellulaire - ECUE MS 2.2 - 2023/2024 PDF
- ANP1111 Lecture 11 Membrane Transport & Excitable Cells Part 1 PDF
- ALSHAWABKEH Introduction to Cell Biology & Genetics PDF
- The Human Organization: The Cell (I) PDF
Summary
This document is a study guide on cell membranes, focusing on their structure, how various substances move across the membrane and different factors impacting membrane fluidity. It details the structure and function of phospholipids and proteins involved, along with types of movement.
Full Transcript
THEME 1 MODULE 1: The Composition and Structure of Membranes Unit 1: Cells in Our Body 1) We began as a single cell, the result of the union of the egg and sperm 2) Mitotic cell divisions create 2 cells, then 4 cells, then eventually we have 10 trillion cells as an adult human...
THEME 1 MODULE 1: The Composition and Structure of Membranes Unit 1: Cells in Our Body 1) We began as a single cell, the result of the union of the egg and sperm 2) Mitotic cell divisions create 2 cells, then 4 cells, then eventually we have 10 trillion cells as an adult human a) Each of the cells vary and differ in function, there may muscle cells, skills cells kidney cells, and neurons b) All of these cells are eukaryotic cells: meaning they have a nucleus that contains most of the genetic material in the cell If we wanted to count the number of cells within our body what do we have to do? 1) There must be a common definition of the term cell 2) What is a cell? Do we mean prokaryotic cells, eukaryotic? 3) We carry a lot of passenger cells within our body, namely prokaryotic cells Eukaryotic cells: - Plant cells, animal cells, fungi Prokaryotic cells: - Bacterial cells There are an estimated 10 times as many bacterial cells in the human body as our own eukaryotic cells - Make up two to three % of our body mass - 150 pound person = 3-4.5 pounds of this weight comes from bacterial cells - Most of these cells are harmless and they are an important part of our bodies - Provide essential functions throughout our physiological systems - Some of these cells are potentially harmful but are contained or managed by our immune system Microbiome: a population of microorganisms or microbes - Organisms not visible to the naked eye, but only visible under a microscope - Prokaryotic bacteria, small eukaryotic organisms - Bacterial cells are found in every part of our body, they are on our skin, our teeth, in our digestive system and respiratory tract - 10 000 distinct species of microbes on and in a healthy human body Streptococcus Aureus (found on money): Able to cause skin and respiratory infections Streptococcus Salivarius: a normal inhabitant of the upper respiratory tract and oral cavity - A member of a collection of bacteria that contribute to the formation of dental plaque - One of the first microbes to colonize a germ-free newborn’s oral cavity and gastrointestinal tract - It resides on our skin and is pretty harmless if it stays there, but if it gets inside the body it can be pathogenic and lead to the activation of the immune system Staphylococcus haemolyticus - Resides on skin Bacteroides thetaiotaomicron: a predominant intestinal bacteria - Makes enzymes that are useful in the breakdown of plant material that we can ingest such as oat fiber Unit 2: Phospholipids are the Building Blocks of Membranes 1) All cells are surrounded by a membrane the contains phospholipids Cell: A membrane-bounds structure containing macromolecules 1) Nucleic acids (DNA & RNA): Hereditary material of the cell and are found in the DNA; chromosome/nucleoid. The information encoded in the DNA is converted into functional products of cells (RNAs and eventually proteins) 2) Proteins: Make up the structural elements of the cell (flagellum on bacterium) and perform metabolic activities (through ribosomes that synthesize enzymes) 3) Polysaccharides/carbohydrates: Important structural components of many cells (bacterial capsule or plant cell wall) and sources of stored energy (foods made from wheat or rice) 4) Phospholipids: Essential part of the cell membrane - an essential component of our definition of a cell Cell Membrane: Membranes separate an internal environment from the external environment. This allows these distinct environments to have different chemical composition. 1) This important to protect the cell from damaging toxins 2) To ensure the cell allows entry of important compounds 3) Disposal of metabolic wastes What components form the structure of a cell membrane? 1) It is made up of lipid macromolecules. Each of these macromolecule has hydrophobic (water hating) core and peripheral hydrophilic (water loving) properties that allow stacked lipid bilayers to form a) Water molecules interact with the head regions of the phospholipid, and as a result the tals will interact with tails to form hydrophobic cores and the hydrophilic heads will interact with water in the extracellular or intracellular environment b) Phospholipids can aggregate and form lipid micelles. These kinds of structures are important for i) Absorption of fat-soluble vitamins and complex lipids in the human body ii) The lipid aggregates form spontaneously without the use of any energy 2) The cell membrane is not thick and rigid, rather they are very thin, from 5-10 nm in thickness, so thin that 100 membranes stacked on top of each other is a piece of paper - Different lipids can be in cells - - Phospholipids consists of a glycerol molecule linked to a phosphate and 2 fatty acids, often called fatty acid tails - These hydrophobic tails make up the hydrophobic core of the bilayer - The hydrophilic head groups (glycerol + phosphate) form the hydrophilic surface that faces the aqueous environments outside and inside the cell. - Phospholipids are said to be amphipathic because they have both a hydrophobic and hydrophilic part. 1) Phospholipid tails consist of a pair of hydrocarbon chains a) There are 16 or 18 carbons in a single chain b) The bonds connection the carbons may be single bonds (saturated) or double bonds (unsaturated) c) These properties change the shape, size and behavior of the phospholipids 2) Steroids: Lipids characterized by a 4-hydrocarbon ring structure. Contain a hydrophilic head group and a hydrophobic tail. How do these components “behave” when they form membranes? What kind of barrier is the cell membrane: What can pass through the cell membrane and what can not? Unit 3: Organization of Cell Membranes Phospholipids are not stationary because membranes are fluid - They can move laterally within one layer of the lipid bilayer, left, right, backward, forward. - However, phospholipids cannot flip easily from one layer to the other in the bilayer without the use of a great amount of energy The fluidity of membranes can be affected by various factors 1) NUMBER OF CARBONS IN THE FATTY ACID TAILS : The number of carbons in the hydrocarbon chain varies: typically there are 16 or 18 carbons. The longer chains pack together more tightly than the shorter chains, reducing the fluidity of the membrane 2) UNSATURATED OR SATURATED FATTY ACIDS Double bonds within a hydrocarbon tail produce kinds or bends in the chain. This has the effect of pushing neighboring phospholipids further apart and increasing fluidity. Unsaturated fatty acid tails can lead to kinds in the hydrophobic tails that affect overall permeability 3) TEMPERATURE External environmental factors such as temperature can also influence membrane permeability. Higher temperatures promote fluidity, while lower temperatures decrease fluidity. Cold-adapted organisms, interestingly tend to have more UNSATURATED phospholipids in their membranes that help to maintain fluidity 4) THE PRESENCE OR ABSENCE OF CHOLESTEROL Steroids such as cholesterol are found in the membranes of every cell in our body, making up about 50% of the molecules found in the lipid membranes. a) A lipid membrane containing just phospholipids is actually TOO fluid. Cholesterol molecules constrain fluidity of the membrane by packing closely to neighboring phospholipids. i) At low temperatures, phospholipid bilayers behave like many other fats; they begin to solidify ii) At these lower temperatures cholesterol helps to maintain fluidity by keeping the phospholipids apart from one another Membrane fluidity: ability of phospholipids to move. The fluidity of the cell membrane allows for transmembrane movements which includes movement of substances from exterior to the interior of the cell and the reverse. - Regions of the membrane high in saturated fatty acids are less permeable than regions of a membrane that contain high amounts of unsaturated fatty acids Region of lower fluidity: Lipid raft, it can sequester or hold macromolecules together in the membrane. These rafts are found to gather proteins involved in the same metabolic pathway or a collection of receptors on the surface of the cell. If the region of the lipid raft is taller - Longer phospholipid hydrocarbon tails - Are straight rather than kinked because they are saturated, allowing phospholipids to pack together lowering the fluidity, making it possible to hold the macromolecules in the raft - A high concentration of cholesterol decreases fluidity within the microdomain of the lipid raft Unit 4: Movement Across Cell Membranes Macromolecules can move laterally within the cell membrane. - The fluidity of the cell membrane allows for transmembrane movements which includes movement of substances form the exterior to the interior of the cell and the reverse - Changing the fluidity will alter how much and how quickly a substance will move across the membrane. Regions of the membrane high in saturated fatty acids (lipid raft) are less permeable than regions of a membrane that contain a high amount of unsaturated fatty acids. Cell Membranes - The ability of cell membranes to control the traffic of substances into and out of the cell and its organelles makes them selectively permeable - Small molecules and ions can cross the membrane along a concentration gradient (from high to low concentration) through a process called diffusion - Small and nonpolar molecules or hydrophobic molecules can pass through the phospholipid bilayers relatively quickly - Larger POLAR substances have greater difficulty moving across the lipid bilayer of the cell membrane if at all How do lipid insoluble molecules and ions get across the cell membrane? 1) Cell membranes are not exclusively made up of lipid macromolecules 2) Rather cell membranes are made up of lipids, proteins, and carbohydrates a) Most have carbohydrates that can be attached to lipids or proteins b) The lipid bilayer is attached with sugar residues, glycolipids, and interspersed steroid molecules such as cholesterol, and many protein channels Membrane-associated proteins can be attached to the cell membrane on the interior or the exterior of the cell, or actually embedded in the phospholipid bilayer 1) When considering movement of molecules in and out of the cell, it is the transmembrane proteins (or integral membrane) embedded within the cell membrane that enable the transport of hydrophilic molecules across the cell membrane The fluid mosaic model states that membranes consist of proteins and carbohydrates embedded in a fluid phospholipid bilayer. - The ability of membrane components to move laterally was found through a scientific model. A testable idea about the way a system works, where the predictions are consistent with the experimental observations made over the last 30 years. Unit 5: Mechanisms of Transport Across the Cell Membrane Passive Diffusion: from areas of high to low concentration (along a gradient) - Movement of small molecules in the direction of a concentration gradient - Molecule comes in contact with and crosses the phospholipid bilayer into or out of the cell Involves the movement of solute molecules 1) Lipid soluble molecules 2) Gasses 3) Uncharged polar molecules and even some water across the cell membrane without the need of any energy 4) Molecule comes in contact with and crosses the phospholipid bilayer into or out of the cell 5) Substances are moving from an area of higher concentration to an area of lower concentration 6) Even some water molecules 7) NO ENERGY REQUIRED Passive Transport (facilitated diffusion): from areas of high to low concentration (along a gradient) - Movement of small molecules with the concentration gradient but involves proteins embedded in the cell membrane and does not require energy - Transport proteins provide a hydrophilic core that allows large hydrophilic molecules most water to move across the cell membrane - Most water crosses the membrane through this way Most common way for substances to move passively across the cell membrane. 1) Generally requires the help of transmembrane proteins that span the width of the phospholipid bilayer 2) Is useful because the phospholipid bilayer is relatively impermeable to ions and most hydrophilic molecules 3) Requires no energy PASSIVE TRANSPORT OSMOSIS: The transport of water across membranes (NO USE OF ENERGY) 1) Water diffuses from a less concentrated solution (hypotonic, chamber A) to a more concentration solution (hypertonic, chamber B) 2) Focus on the concentration of solutes in the solution on either side of the selectively permeable membrane 3) The net movement of water is the process of equilibrating the osmotic concentrations on either side of the membrane PASSIVE TRANSPORT (FACILITATED) 1) The movement of water across selectively permeable biological membranes can go at a much faster rate due to aquaporin transmembrane proteins. 2) These protein channels exclusively allow water to pass through hydrophilic protein cores through the process of osmosis at over 10 times the rate water takes to move directly through the phospholipid bilayer Active Transport: molecules move against the concentration gradient (fueled by energy from the hydrolysis of ATP. - Movement of molecules against a concentration gradient and involves proteins embedded in the cell membrane which require energy from ATP to drive transport ISOTONIC ENVIRONMENT: Scenario One (Same extracellular fluid concentration with the same osmolarity as its interior) - No net movement of water - The cell retains its cell shape and optimal cellular activities - Red blood cells in isotonic solutions retain their concave disk-like shapes HYPOTONIC ENVIRONMENT: Scenario 2 (Lower solute concentration than the inside of the cell). - The net movement of water down its concentration gradient - Results in excessive movement of water into the cell and leads to the cell swelling or even nursing, when cells burst they become “ghost cells” HYPERTONIC ENVIRONMENT: Scenario 3 (an environment with a higher solute concentration on the exterior of the cell relative to the interior of the cell can lead to the net loss of water from the cell interior towards the exterior environment) - Cell membrane shrinks and shrivels ACTIVE TRANSPORT This type of movement requires energy which is provided by the hydrolysis of ATP molecules on the intracellular side of the cell - If the transmembrane transport protein is directly affected by the energy released from ATP hydrolysis, it undergoes a conformational change to pump the substance across the membrane against a concentration gradient (PRIMARY ACTIVE TRANSPORT) - Direct expenditure of ATP-dependent - IF NEIGHBORING transmembrane transport proteins take advantage of electrochemical gradients established by their primary active transport pumps to move their own solutes against the gradient, this is SECONDARY ACTIVE TRANSPORT Sodium Potassium Pump: Embedded in many cellular membranes is a classic example of primary active transport mechanisms 1) The pump is essential in allowing the cell to establish differences in the concentration of sodium and potassium on either side of the cell membrane, which is very important for biological processes a) ENERGY IS REQUIRED AND COMES FROM THE HYDROLYSIS of ATP b) In most cells there is a greater concentration of potassium in the interior of the cell relative to the extracellular environment of a cell in our body c) More sodium on the outside d) This concentration difference is maintained by the ACTIVE role played by the sodium-potassium pump How it works: 1) For every 3 sodium ions pumped out, 2 potassium ion are pumped from the extracellular environment into the interior of the cell 2) The energy molecule ATP gives up an energetic phosphate group to the transport protein because this occurs against the concentration gradient a) This energetic phosphate group that binds and causes a conformational change in the transport protein, and therefore allows the release of the sodium ions into the extracellular fluid in exchange for potassium b) When the sodium potassium pump binds 2 potassium ions that will enter the cell, the phosphate group is released from the transport protein and the protein returns to its original shape and recess potassium ions in the cytoplasm Net loss of positive ions (resting potential of the cell is slightly more negative) THEME 1 MODULE 2: THE STRUCTURE OF THE CELL, ORGANELLES AND ENERGY Unit 1, Comparing Eukaryotic and Prokaryotic cells Life began 4 billion years ago, it was exclusively unicellular for most of Earth’s history because oxygen was absent from the oceans and atmosphere - Harsh in hospital environment The first photosynthetic cells (could create oxygen) opened up diversity that led to the variation of organisms that exist today. - One of the most interesting time-points in the history of life was the appearance of the first photosynthetic cells, especially the first photosynthetic eukaryotes that evolved in the Proterozoic eon. - Many unicellular organisms began interacting with one another, and the interactions that allowed these organisms to deal with harsh environments until these organisms became co-dependent. Both have a: An external membrane that separates the inside of the cell from the outside of the cell. Eukaryotic cells: an organisms whose cells contain a nucleus and many membrane bound organelles - Internal network of membranes - Double membrane bound nucleus that serves as an internal compartment to separate the genetic material of the cell (chromosomes) from the rest of the cell interior - True compartmentalized nucleus Prokaryotic cells: unicellular organisms that lack a nucleus and have few to no organelles 1) Life on earth was almost exclusively unicellular for most of Earth’s history with oxygen virtually absent until the first photosynthetic cells 2) Prokaryotic cells have been around the longest, eukaryotic cells appeared about 2 billion years ago Early Eukaryotes: Single-celled organisms that contained organelles and internal membrane systems with distinct structures and functions. Organelles: Membrane-bound structures inside the cell Chloroplasts: In plant cells. Photosynthetic protists and plant cells contain chloroplasts, actively engage in photosynthesis, producing chemical energy from light energy. - Has a double membrane around its exterior and an interior filled with hundred of flattened and stacked membranes called thylakoids - Thylakoid stacks are organized into piles called grana - In these membranes, pigments and enzymes participate in photosynthesis Mitochondria: In animal and plant cells. Mitochondria generate energy in the form of ATP. Convert energy from the food we eat into energy that powers most cellular functions in our bodies. - Mitochondrial malfunction = exhaustion, a common symptom of mitochondrial diseases Unit 2: The Evolution of Chloroplasts and Mitochondria Photosynthesis: a process in plant cells and other organisms to convert light energy into chemical energy. Chemical energy is stored in the bonds of carbohydrate molecules - We rely on photosynthesis to produce sugars we consume in our diets Cellular respiration: a process used by plant and animal cells to release the chemical energy stored in the bonds of carbohydrate molecules and partially capture it in the form of ATP. - Chemical requirements of our bodies can be obtained from sugars we consume - We have between 50 to over a million mitochondria within one of our cells, depending on which type - Mitochondria have two membranes - Outer Membrane: Surrounds the organelle - Inner membrane: Connected to a series of sac-like structures called cristae. - It is in these membranes that most of ATP is synthesized in eukaryotic cells. How did mitochondria end up in our cells? - Dr. Lynn Margulis proposed that eukaryotic cells originated as communities of interacting entities that joined together - She thought prokaryotic cells were ingested or entered into a host cell, where over time the prokaryotes and eukaryotes developed a mutually beneficial interaction and became obligatory for host survival. - Mitochondria and chloroplasts are two distinct membrane bound compartments thought to have entered from external origins. Mitochondria - Look like bacterial cells - About the same size as bacterial cells - Have their own circular genome - Produce the enzymes necessary for protein synthesis Why is it advantageous to develop this type of endosymbiotic relationship? 1) The early atmosphere of the Earth was a hostile environment 2) Anaerobic ancestral eukaryotes had the capability of producing small amounts of ATP from carbon compounds, and the interactions with aerobic bacteria are able to produce high amounts of aTP from the same carbon compounds through a complex membrane system 3) It is likely that a mutually advantageous relationship developed when anaerobic eukaryotes engulfed aerobic bacteria a) The bacterium could supply lots of energy, while the eukaryote provide a safe environment with a supply of carbon compounds Endosymbiotic theory of organelle evolution: 1) A timeline that outlines how all cells were derived and modified from an ancestral prokaryote that contains genetic material of inheritance 2) Cell membrane allowed ancestral prokaryotes to compartmentalize the genetic information into a nucleus and obtain greater regulation of games and processing of proteins to become most ancestral eukaryotes 3) These cells containing nuclei and an endomembrane system became hosts to aerobic and photosynthetic prokaryotes (modern-day mitochondria and chloroplasts) 4) All eukaryotic cell types have mitochondria, but not all have chloroplasts Unit 3: What is the scientific evidence that supports the endosymbiotic theory of organelle evolution? A hypothesis or theory leads to a set of predictions. What predictions can we make if the endosymbiotic theory is an accurate representation of organelles such as the chloroplast and mitochondria? 1) One prediction is that there might be living examples of acquired endosymbiotic relationships which can be seen in eukaryotic organisms a) There are examples of eukaryotic organisms that acquire the ability to engage in photosynthesis only after they engulf a photosynthetic bacteria that will perform those functions (phagocytosis by an early eukaryote, first step in the origin of plastids) b) Green Algae cymbomonas is an example where living bacteria is maintained inside the protists and share their photosynthetic products with the protist host, both organisms benefit from this relationship These types of relationships are also seen in other animals. (temporary endosymbiotic relationships) 1) Corals (similar to the human microbiome) live symbiotically with microbes called dinoflagellates. They photosynthesize as long as they have light and nutrients. They don’t keep the products of photosynthesis but release them to coral. 2) Sea slugs take this relationship one step further, the sea slug consumes photosynthetic algae and harvests the chloroplast from the algal cells. These chloroplasts are maintained in the cells of the sea slug. Endosymbiotic relationships in the context of evolution 1) These temporary relationships must become permanent and heritable 2) When the host cells divides, then the organelles are distributed evenly between two daughter cells 3) Both mitochondria and chloroplasts contain their own circular genomes, such as found in bacteria, and analysis of the genes shows significant similarity to bacteria Different organelles existing within the cell allow for compartmentalization of different cellular functions. Organelles are a distinct domain within a cell with a specific function Different compartments contain: 1) A unique set of enzymes 2) Produce and contain different products Increased membrane surface area can 1) Increase the potential metabolic capacity across the membrane This is advantageous because: 1) Specific sets of enzymes responsible for specific biochemical functions can be kept in close proximity to each other 2) Chloroplast has all enzymes necessary for photosynthesis, mitochondria for aerobic respiration 3) Efficiency of chemical reactions is increases if enzymes, substrates, and products are concentrated in an organelle 4) Incompatible processes like synthesis and degradation can be kept separate and not interfere with each other Unit 4, How do these organelles function in the cell? A chloroplast that is actively engaging in photosynthesis, harnesses light energy and together with atmosphere carbon dioxide it makes important organic sugars and gaseous oxygen. The energy present in sugars can then flow into the remaining ecosystem. 1) Living things need energy from outside sources (non-photosynthetic organisms) because they lack chloroplasts and cannot harness light energy to make organic molecules 2) Much energy is provided through metabolism of organic molecules produced by chloroplasts in the plants that we consume directly, or by feeding animals that feed on plants 3) Cyclists intake sugars to synthesize ATP to power their muscle cells How do chloroplasts make energy? 1) Presence of sunlight, carbon dioxide, water, and the primary energy source being produced as carbohydrates as glucose and oxygen as a byproduct 2) During the photosynthetic cascade of events, light energy is transformed into chemical energy in the form of ATP and NADPH which then enters the Calvin Cycle 3) In the Calvin cycle NADPH and ATP are used to drive the reduction of atmospheric carbon dioxide into carbohydrates This cascade shows the important of light reactions and the calvin cycle to produce sugar and oxygen Carbohydrates are the most abundant sources of ATP that fuel our bodily processes 1) Formed by the polymerization monosaccharides through glycosidic bonds to form complex sugars 2) Monosaccharides include glucose, fructose, and galactose 3) Special proteins called enzymes can catalyze the condensation reaction between specific hydroxyl groups of certain monosaccharides to produce disaccharides such as lactose, sucrose, maltose and others (metabolism of carbohydrates requires proteins called enzymes) 4) The reaction occurs between the OH group of carbon 1 of one molecule and carbon 4 of another monosaccharide to give either a) Alpha 1-4 glycosidic linkage b) Beta 1-4 glycosidic linkage Polysaccharides are the structures that form when many monosaccharides are linked together 1) Simple disaccharides or more, or larger and more common polysaccharides like starch and glycogen 2) Starch is the storage polysaccharide found in all photosynthetic plants made up of a) Alpha 1-4 glycosidic linkages between alpha-glucose monomers Two types of starch include two types of polysaccharides 1) Unbranched amylose 2) Branched amylopectin 3) These differences in structure vary only based on the interactions between neighboring carbon atoms of each glucose monomer a) In amylopectin the branches formed by glycosidic linkages between the carbon 1 of one glucose molecule and carbon 6 of another b) It is often these starches that we consume and use as energy in our own bodies When starches are consumed, we store digested carbohydrates as highly branched glycogen polysaccharide helices. This stored glycogen energy can be broken into glucose through cellular respiration to produce ATP for necessary cellular processes. Our cells are dynamic 1) We require molecular fuel to support our activities 2) The molecular fuel that gives energy for all our cellular processes is derived from ATP a) Cells can generate ATP from the metabolism of simple sugar molecules (cellular respiration) i) 4 steps take place in cellular respiration that result in ATP synthesis ii) A given cell typically only contains enough ATP to fuel 30 seconds - 1 minute of cellular activity, meaning the evolution of fast glucose processing systems has occurred (they are constantly active and efficient) Glycolysis: Occurs in the cytosol of the cell and leads to the production of 2 molecules of ATP and 2 NADH molecules that act as electron donors Step 1 of Glucose Processing 1) Glycolysis results in the production of 2 molecules of a 3-carbon compounds called pyruvate (NADH) for each molecule of glucose 2) Following glycolysis, pyruvate is processed to form acetyl CoA, which is free to enter the mitochondrial matrix of the mitochondria, while also producing carbon dioxide and NADH 3) Once in the mitochondrial matrix, acetyl CoA is further processed in the Krebs cycle producing ATP, carbon dioxide, and additional electron donors in the form of NADH and FADH2. These electron donors will add up during glucose processing to facilitate production of ATP in the mitochondria Not much ATP is produced in the first 3 steps of glucose processing, not a whole lot of ATP is synthesized 4) The bulk of the electron donors are used in the electron transport chain in the inner mitochondrial membranes or cristae to produce another 32 molecules of ATP a) As a result for every glucose molecule that we process in our cells, we produce 36 theoretical ATP, with negligible amounts coming from glycolysis and the Krebs cycle and most ATP forming when electron donors NADH and FADH2 move through the protein complexes of the electron transport chain in the extensive inner mitochondrial membranes b) The transfer of these electrons allows protons to be pumped into the intermembrane spaces of the mitochondria i) Creates an electrochemical concentration gradient across the inner mitochondrial membranes that drives protons through the ATP synthase protein channels that are embedded in membranes ii) This results in the synthesis of 32 additional ATP Until recently it was thought 36 molecules of ATP were synthesized per molecule of glucose, the theoretical maximum, but recent research using more precise measurements suggests its closer to 30 ATP molecules per molecule of glucose A proton gradient drives the synthesis of ATP Carbohydrates are not the only source of energy used to make ATP 1) Proteins and lipids can be converted into components that enter into the cellular respiration pathway and lead to the production of ATP 2) All three of these macromolecules contribute to ATP production, carbohydrates are metabolized first, then fats, and then proteins Why is ATP a source of molecular energy? 1) It fuels cellular processes because it has a very high potential energy. ATP has 3 phosphate groups attached to a ribose sugar and an adenine. The 3 phosphate group shave 4 negative charges in close proximity, so these negatively charged phosphate groups repel each other, giving the electrons of the phosphate groups a very high potential energy 2) The potential energy of ATP can be released and harnessed during hydrolysis, where ATP can react with water and beak the bond between the outermost phosphate group and its neighbor resulting in the formation of ADP and inorganic phosphate 3) The hydrolysis of ATP is highly exergonic (releases energy) The cell’s interior machinery takes advantage of the energy that is released during atp hydrolysis to perform, mechanical transport, and chemical work - Cyclist pedaling uses ATP hydrolysis to fuel the contraction of his or her muscles during strenuous exercise - ATP is also important for the transport of substances across the cell membrane AGAINST a concentration gradient - Many enzymes within a cell require energy to perform their functions and the cell is then able to use ATP-derived energy to help drive endergonic or energy requiring reactions Today’s eukaryotes were likely derived and modified from an ancestral prokaryote that contained genetic material of inheritance. 1) The endosymbiotic theory states that these early eukaryotes became hosts to aerobic and photosynthetic prokaryotes which are now mitochondria and chloroplasts Endomembrane system: a group of organelles in eukaryotic cells that performs most lipid and protein synthesis; endoplasmic reticulum, golgi apparatus, and lysosomes 1) 1) i) - 1) - - 1) 1) 1) i) 1) 1) - - 2) 2) 2)