60-Glom Filtration, Hemodynamics-Notes_2024 PDF
Document Details
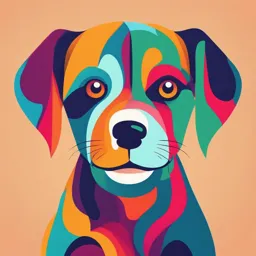
Uploaded by FruitfulIntegral
Wayne State University
Tags
Related
- Renal Physiology Part 3 (Glomerular Filtration) PDF
- BRS Physiology PDF - Glomerular Filtration Rate
- Renal Physiology Lecture 3 - Autoregulation of Glomerular Filtration PDF
- Glomerular Filtration Lecture Slides PDF
- Assessing Renal Function PDF
- Lecture 25: Glomerular Filtration Rate & Renal Clearance - Fall 2024
Summary
These notes cover the regulation of glomerular filtration and renal hemodynamics. They discuss core concepts like the Starling-Landis Principle, glomerular capillary forces, and renal resistance, as well as intrinsic and extrinsic regulation. The detailed analysis of these processes should prove useful for medical physiology students.
Full Transcript
WSUSOM Medical Physiology Rossi-Renal Physiology Page 1 of 12 Regulation of Glomerular Filtration and Renal Hemodynamics Regulation of Glomerular Filtration and Renal Hemodynamics 1. Glomerular filtration rate A. Review the Starling Landis Princi...
WSUSOM Medical Physiology Rossi-Renal Physiology Page 1 of 12 Regulation of Glomerular Filtration and Renal Hemodynamics Regulation of Glomerular Filtration and Renal Hemodynamics 1. Glomerular filtration rate A. Review the Starling Landis Principle as it relates to filtration at the glomerular capillary and the forces that contribute to filtration pressure at this site with a view to full understanding of the impact of alteration in any one parameter on glomerular filtration rate 1) Define and understand the forces exerted by hydrostatic and oncotic pressures 2) Distinguish between osmotic and oncotic pressure 3) Recognize the influence of the ultrafiltration coefficient and the parameters that contribute to its determination B. Distinguish between filtration equilibrium and filtration disequilibrium at the glomerulus and the influence each would have on glomerular filtration rate during different conditions of blood flow C. Be able to calculate glomerular filtration rate and changes in glomerular filtration rate given changes in any of the parameters that alter Starling forces 2. Renal hemodynamics A. Review how flow is related to resistance and pressure within the blood vessel B. Recognize the uniqueness of the anatomical configuration of the renal microvasculature (afferent arteriole, glomerular capillary, efferent arteriole) and comprehend that the arrangement of serial resistance vessels imparts additional control over transcapillary pressures in the glomerulus C. Be able to predict what changes in afferent and efferent arteriolar resistances will exert on transcapillary pressure and, hence, glomerular filtration rate and renal plasma flow D. Distinguish the extrinsic factors, both endogenous (neural and humoral) and exogenous (drugs), that influence arteriolar resistances and their impact on renal plasma flow and glomerular filtration rate E. Identify the intrinsic factors that impact autoregulation of renal plasma (blood) flow and glomerular filtration rate 1) Define and understand the myogenic response, including the structures involved in this response 2) Define and understand tubuloglomerular feedback, including the structures and factors involved in transducing this feedback information WSUSOM Medical Physiology Rossi-Renal Physiology Page 2 of 12 Regulation of Glomerular Filtration and Renal Hemodynamics Starling-Landis Principle Starling Forces in the Glomerular Capillaries Filtration Pressure = [(Pc- Pt) - (pc - pt)] Glom Filtration Rate = Kf [(Pc- Pt) - (pc - pt)] Pc = capillary hydrostatic pressure Pt = tubular hydrostatic pressure pc = capillary oncotic pressure pt = tubular oncotic pressure Kf = ultrafiltration coefficient Colloids are the large molecular weight particles in a solution. In plasma, the major colloids are plasma proteins. The component of the total osmotic pressure due to the colloids (proteins) is known as the colloid osmotic pressure, or oncotic pressure. Oncotic pressure is about 0.5% of the total osmotic pressure but because colloids cannot cross the plasma membrane easily, oncotic pressure is extremely important in transcapillary fluid dynamics. Recall van’t Hoff equation: osmotic pressure = n * cRT/m where n = number of particles into which the substance dissociates in solution c = the concentration in grams/liter m = is the molecular weight R = is the universal gas constant T = the absolute temperature (Kelvin) Total plasma osmotic pressure is about 5,409 mmHg (ie., 7.1 atmospheres). The calculated contribution by plasma proteins (oncotic pressure) is 17.3 mmHg but the measured oncotic pressure is closer to 25 mmHg, due to a composite of other factors (Gibbs-Donnan equilibrium, excluded volume effect, temperature, pH, protein shape). Starling Forces also apply to filtration at the glomerulus but the glomerulus is configured as afferent arteriole - glomerular capillary - efferent arteriole. Note that for simplicity sake we say that albumin and plasma proteins are not filtered, but that is an over simplification. Small proteins like light chains (of immunoglobulins), insulin, vasopressin, etc. are filtered and then reabsorbed by the proximal tubule. Even in normal people, some albumin is filtered but ultimately all reabsorbed by the proximal tubule (via megalin mechanisms) so that no albumin ends up in the urine. For practical purposes (and Step 1, 2 and 3), proteins are not filtered by the normal glomerulus. WSUSOM Medical Physiology Rossi-Renal Physiology Page 3 of 12 Regulation of Glomerular Filtration and Renal Hemodynamics A reminder of the real anatomy of the nephron (see Figure). We will simplify later for ease of conceptualization Pc = hydrostatic pressure within the glomerulus, starts at 60 mmHg and decreases with distance. Pt = hydrostatic pressure within Bowman’s space and tubule (similar to ISF) approximately 10 mmHg pc = plasma colloid oncotic pressure, starts at 25 mmHg and increases with distance along the glomerular capillary (as protein-free fluid is filtered) pt = tubular fluid oncotic pressure, normally nearly 0 mmHg The arteriole-capillary-arteriole configuration permits higher hydrostatic pressures within the glomerular capillaries (compared with the typical capillary bed. Pc = 60 mmHg in glomerulus vs 40 at beginning of capillaries elsewhere). Notice that the plasma leaving the efferent arterioles and going into the peritubular capillaries thus has a lower hydrostatic pressure and a higher oncotic pressure. This will be important to keep in mind for later. Again, we are going to be generalizing quite a bit. Be sure to remember that not all glomeruli and nephrons are identical. The superficial nephrons have lower GFR and shorter loops of Henle than the juxtamedullary nephrons. For our purposes we will talk about all the nephrons as being the same but keep this in mind as these differences influence the susceptibility to pathology (next year; e.g., diabetic nephropathy and focal segmental glomerulosclerosis). WSUSOM Medical Physiology Rossi-Renal Physiology Page 4 of 12 Regulation of Glomerular Filtration and Renal Hemodynamics 1. Glomerular capillaries filter much more that all other capillaries combined. In normal adult humans GFR = 125 ml/min = 180 L/day (vs 20 L/day for all systemic capillaries.) Since pc is the same in plasma entering the capillaries (glom and systemic), logically, glomerular capillaries must have either a higher hydrostatic pressure (higher Pc) or a higher Kf. In the kidney, Pc is higher and likely Kf is too. Kf is the filtration coefficient and varies among different capillary beds (kidney, heart, muscle, etc.). Kf includes factors for surface area and permeability characteristics of the capillaries. Not all capillaries are alike! 2. Changes in Kf, Pc, Pt, pc, and pt will alter GFR in predictable ways. E.g., all other factors being constant, an increase in Pt will decrease GFR (see the formula) If more than one variable changes, the net change in GFR will be harder to predict. 3. The most important variable for controlling the GFR is Pc, the hydrostatic pressure within the glomerular capillaries. (Pc and Pt have not been measured in humans. The above values were obtained in rats, but values are similar in other species that have been tested, so we assume the same in humans.) Kf, Pc, Pt, pc and pt can change. These changes can alter GFR in predictable ways (refer to formula) Pc= most important variable controlling GFR! If all other variables were constant, what would happen to GFR 1. If systemic arterial pressure decreases to very low levels? 2. If the ureters are obstructed? 3. If the disease causes an increase in vascular permeability? WSUSOM Medical Physiology Rossi-Renal Physiology Page 5 of 12 Regulation of Glomerular Filtration and Renal Hemodynamics GFR Glomerular capillaries filter more than other capillaries GFR = 100-125 ml/min OR 144 - 180 L/day all other capillaries combined » 20 L/day Question Does filtration occur throughout the glomerular capillary from the afferent arteriole all the way to the efferent arteriole? To put it another way, do we use ALL the surface area of our glomerular capillaries to filter all the time? Answer: that depends…let’s see GFR = Kf [(Pc- Pt) - (pc - pt)] DP Dp Filtration equilibrium exists when DP = Dp; at that point no more net filtration occurs. If filtration equilibrium exists, then RBF ® GFR Filtration Equilibrium or NOT? The figure above shows two scenarios regarding glomerular filtration. The graph on the left depicts filtration equilibrium. The graph on the right shows filtration disequilibrium. Filtration equilibrium occurs when the pressure favoring filtration ∆P (primarily Pc) is perfectly balanced with the pressure opposing filtration, Dp , such that [(Pc - Pt) - (pc - pt)] = 0 before the end of the glomerular capillaries (arrow on left graph). At that point net filtration STOPS (left panel above). (Unlike other vascular beds where the venular side begins to reabsorb fluid from the interstitium this does NOT occur in the glomerulus. Once fluid is filtered it flows into the tubules. Reabsorption is the responsibility of the tubules and their capillaries. If Dp never equals ∆P (right panel), filtration will occur throughout the whole glomerular capillary from one end to the other. Consider: What will happen to GFR if the filtration equilibrium on the left graph is achieved farther along the glomerular capillaries. WSUSOM Medical Physiology Rossi-Renal Physiology Page 6 of 12 Regulation of Glomerular Filtration and Renal Hemodynamics If filtration equilibrium DOES exist, then an increase in RBF will lead to an increase in GFR (and vice versa). As RBF increases, the point at which Dp rises to equal ∆P will move farther toward the efferent arteriole (to the right). In other words, Dp rises slower and more of the glomerular capillaries are used for filtration. Thus, the surface area used for filtration will increase (Kf increases) and the area between the curves (= GFR) will be larger. This occurs during physiological conditions such as after a large protein meal or normal pregnancy. This is known as “renal reserve” and may be lost in patients with chronic kidney disease as they may not have glomerular capillaries that are not being used! If filtration disequilibrium exists (right graph) the impact of increased RBF on GFR is less dramatic. (Think about the reason why this might happen, but do NOT rack your brains over it.) Trivia: Rats and humans are in filtration equilibrium. Dogs are in filtration disequilibrium. Here is a typical depiction of a glomerulus with filtration disequilibrium and typical variables at the afferent and efferent end of the glomerular capillary bed (do not memorize the numbers). PF is the net ultrafiltration pressure: note it is higher at the afferent than at the efferent end. The afferent and efferent arterioles are the major sites of resistance to blood flow. Changes in contractility affect the radius of the blood vessels, which affect the resistance. Changes in resistance affect the renal blood flow (RBF) and the glomerular capillary hydrostatic pressure, Pc. Thus, changes in resistance affect glomerular filtration rate, GFR. Left: normal glomerulus Right: diabetic nephropathy Note loss of open capillaries on the left (loss of surface area for filtration = decrease in Kf) The glomerulus on the left is normal. The one on the right has diabetic changes (more next year). BUT you should appreciate that the surface area of OPEN capillaries available for filtration to occur is markedly decreased in the diseased glomerulus! WSUSOM Medical Physiology Rossi-Renal Physiology Page 7 of 12 Regulation of Glomerular Filtration and Renal Hemodynamics Control of Renal Hemodynamics Q=P R Q = flow; P = pressure; R = resistance Sites of resistance = afferent & efferent arterioles change in contractility ® change in vessel radius ® change in resistance ® change in RBF and Pc ® change in GFR RBF can be related to resistance and pressure by analogy to Ohm’s Law: Ohm’s Law: current = voltage resistance RBF = pressure Q=P resistance R Thus, if systemic arterial pressure is constant, changes in resistance will result in predictable changes in Pc and therefore RBF and GFR. See next slide. Two ways of illustrating what happens to glomerular capillary pressure (Pc or Pgc) on this and the next page/slide when you constrict the afferent vs the efferent arteriole. Notice that Pc changes in opposite directions depending on whether vasoconstriction occurs PRE glomerulus or POST glomerulus. Note below where the big drops in pressure are. Those are the sites of maximum resistance (afferent and efferent arterioles). WSUSOM Medical Physiology Rossi-Renal Physiology Page 8 of 12 Regulation of Glomerular Filtration and Renal Hemodynamics Thus, GFR will change differently depending on PRE vs POST glomerulus resistances…though RBF will change similarly …see next few slides. Too bad we can’t do the “glomerular ballet” to illustrate that we used to do in class! Renal hemodynamics: Q = P/R Example: At a given systemic arterial blood pressure, if the afferent arteriole constricts, resistance prior to the glomerular capillaries will increase, Pc in the capillaries will decrease, RBF will decrease and GFR will decrease. However, at the same systemic pressure, if the efferent arteriole constricts, resistance after the glomerular capillaries will increase. In this circumstance, the hydrostatic pressure within the glomerular capillaries, Pc, will increase and GFR will increase, even though RBF decreases. Combinations of changes in resistance to both afferent and efferent arterioles can occur. Under such circumstances the directional change in RBF and/or GFR can be more complex and difficult to predict. The major role of these arterioles is to keep Pc constant so that GFR does not vary widely! Filtration Fraction Filtration fraction = the fraction of the plasma flowing through the glomerulus that is filtered. FF = GFR = Cin eRPF CPAH What will happen to FF if you constrict the efferent arteriole? The afferent arteriole? If you constrict the efferent arteriole, eRPF will go down and GFR will go up, so filtration fraction will go UP. When a person is hypotensive (below the autoregulatory range (next few slides), substances are released (angiotensin II) that work on the efferent arteriole to constrict it and maintain GFR even in the face of severe hypotension. In these circumstances the FF will be higher. WSUSOM Medical Physiology Rossi-Renal Physiology Page 9 of 12 Regulation of Glomerular Filtration and Renal Hemodynamics If the person is on a drug that blocks angiotensin formation or action (commonly used for hypertension and heart failure) and then gets hypotensive (hemorrhage, septic shock, etc.), this protective response may be hindered and GFR may drop dramatically. Regulation of GFR and RPF 1. Intrinsic control (within the kidney itself) 2. Extrinsic control (outside the kidney) Intrinsic Control Afferent arteriole resistance µ systemic arterial BP Arterial Flow = Pressure_ Resistance Autoregulation: RBF and GFR constant over a wide range of systemic pressures » 80-180 mmHg (mean arterial pressure). Autoregulation is due to intrinsic control. Renal nerves and circulating hormones are NOT involved in AUTOregulation! They are OUTSIDE the kidney. Local AUTOcrine substances released by the cells within the kidney are involved in AUTOregulation: e.g., nitric oxide, adenosine, prostaglandins, etc.). Observation: RBF and GFR are constant over a wide range of arterial pressures, from » 80 mmHg to » 180 mmHg. RBF = Pressure Resistance Therefore, for RBF (or GFR) to remain constant arteriolar resistance must change proportionally to arterial pressure. That is, if blood pressure increases (or decreases) there must be a proportional increase (or decrease) in afferent arteriolar resistance. This mechanism is known as autoregulation. Autoregulation occurs by an intrinsic mechanism that does NOT involve renal nerves or circulating humoral substances. Non –Autoregulation Autoregulation Systemic pressure ® RBF ; GFR Systemic pressure ® aff art resistance resistance is constant RBF and GFR remain constant WSUSOM Medical Physiology Rossi-Renal Physiology Page 10 of 12 Regulation of Glomerular Filtration and Renal Hemodynamics If pressure ® efferent art resistance, then RBF would be autoregulated but NOT GFR. Therefore, autoregulation must regulate Pc via the afferent arteriole. Two major theories for intrinsic mechanism of autoregulation of RBF (or GFR): 1. Myogenic response 2. Tubuloglomerular feedback Intrinsic Mechanisms of Autoregulation To understand the intrinsic mechanisms of autoregulation, recall the organization of the nephron unit: The tubules are organized so that the end of the thick ascending limb of Henle as it emerges to become the distal convoluted tubule passes between the afferent and efferent arteriole of the glomerulus associated with that same tubule. This area is known as the juxtaglomerular apparatus (JGA) and is involved in tubuloglomerular feedback (and release of renin more about this later). This is a specially organized structure comprised of - macula densa cells (specialized cells of the thick ascending limb) - extraglomerular mesangial cells - granular cells (renin producing cells of the afferent arteriole) 1. Myogenic response pressure within afferent arteriole ® stretch of vascular smooth muscle cells (VSMC) ® force of contraction ® resistance Decreased pressure within the afferent arteriole leads to opposite effects. 2. Tubuloglomerular feedback: The flow rate and ion content of the fluid in the distal tubule are sensed at the juxtaglomerular apparatus (JGA) and the information is transmitted by paracrine (“local”) factors (nitric oxide, adenosine, prostaglandins) to the vascular smooth muscle cells of the afferent arteriole. Resistance of the afferent arteriole changes accordingly. WSUSOM Medical Physiology Rossi-Renal Physiology Page 11 of 12 Regulation of Glomerular Filtration and Renal Hemodynamics Example: If GFR were to increase, then distal tubular fluid flow rate and/or delivery of NaCl content to the JGA would increase. That is the LOAD (or amount) of NaCl to the JGA increases. The macula densa cells sense this increase in ion content and send back a message to the afferent arteriole that RBF needs to decrease. Thus, the arteriole constricts, resistance increases, and RBF (and GFR) decreases. Conversely, decreases in distal tubule flow rate and/or NaCl content lead to decreased resistance, dilation of the arteriole and increased RBF and GFR. The myogenic response and tubuloglomerular feedback are not mutually exclusive. Both mechanisms may be engaged simultaneously in controlling resistance. Recent data indicate that the myogenic response accounts for about 60% of autoregulation and tubuloglomerular feedback for about 40%. What is/are the ion signal in the macula densa? It is now thought that a rise in [NaCl] at the tubular lumen side of the cell together with a Cl- channel on the basolateral membrane leads to depolarization, which activates a calcium channel. The calcium channel lets Ca2+ enter the macula densa cell. The resulting increase in [Ca2+]i causes the macula densa cell to release paracrine agents (nitric oxide, adenosine, prostaglandins, etc.) that trigger contraction or dilation (as appropriate) of the vascular smooth muscle cells of the arterioles. Clinical tidbit: Nonsteroidal anti-inflammatory medications (e.g., ibuprofen, naproxyn, etc.) inhibit formation of protective prostaglandins. These drugs are used to treat arthritis but act on the macula densa area to impair tubuloglomerular feedback and may cause impaired kidney function in some circumstances via this mechanism. Extrinsic Control (NOT Autoregulation) – when things get really bad! Arteriolar resistance is also controlled by extrinsic factors. Two major categories: 1. Neural – renal sympathetic nerves renal sympathetic nerve activity local release norepinephrine activation of a adrenergic receptors vasoconstriction (afferent and efferent) 2. Humoral - hormones, metabolites, drugs a adrenergic - constriction b adrenergic - dilation angiotensin II - constriction (primarily efferent) vasopressin - constriction adenosine A1, constriction; A2 dilation Endothelin, prostaglandins, nitric oxide, cytokines, the list goes on... WSUSOM Medical Physiology Rossi-Renal Physiology Page 12 of 12 Regulation of Glomerular Filtration and Renal Hemodynamics Renal nerves are NOT essential for normal renal function. The nerves to the kidney itself are sympathetic nerves. Note that transplanted kidneys are denervated and can still regulate RBF and GFR normally. (The kidney is NOT innervated by parasympathetic nerves [new data emerging but not yet replicated], although the bladder is.) Extrinsic control becomes very important in times of stress such as hemorrhagic shock. The humoral factors listed above are among numerous vasoactive substances that can impinge on receptors on the vascular smooth muscle cells of the afferent and efferent arterioles. The mesangial cells of the glomerulus also carry receptors and the mesangial cells can also respond to these substances. Filtration Pressure = [(Pc- Pt) - (pc - pt)] GFR = Kf [(Pc- Pt) - (pc - pt)] Review the forces involved in and influencing RBF and GFR for yourself.