Zoology Past Paper PDF
Document Details
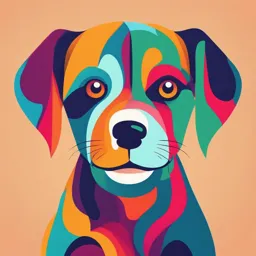
Uploaded by OverjoyedMothman2580
University of Santo Tomas
Tags
Summary
This document discusses Platyhelminthes, commonly known as flatworms. It details their characteristics, including their classification into different classes and their free-living and parasitic forms. The document also describes their life cycle and digestive systems. It seems to be part of a larger zoology textbook or lecture notes.
Full Transcript
Figure 14.5 Hypothetical relationships among members of Platyzoa. Characters are modified subsets of those in Kristensen (2002; see p. 318 for citation) and Brusca and Brusca (2003; see p. 330 for citation). 14.3 PHYLUM PLATYHELMINTHES Members of phylum Platyhelminthes (Gr. platy...
Figure 14.5 Hypothetical relationships among members of Platyzoa. Characters are modified subsets of those in Kristensen (2002; see p. 318 for citation) and Brusca and Brusca (2003; see p. 330 for citation). 14.3 PHYLUM PLATYHELMINTHES Members of phylum Platyhelminthes (Gr. platys, flat, + helmins, worm) are commonly called flatworms. They range in size from a millimeter or less to many meters in length (for some tapeworms), although most are 1 to 3 cm. Their bodies may be slender, broadly leaflike, or long and ribbonlike. The phylum contains free-living forms, such as the common planarian (see Figure 14.6), as well as parasitic flukes and tapeworms. Page 299 Figure 14.6 A, Stained planarian. B, Bipalium, a terrestrial flatworm. Because there is no single unique characteristic (synapomorphy) for the phylum as a whole, some authorities argue that the phylum Platyhelminthes is not a valid monophyletic group. However, Platyhelminthes emerges as a clade in recent molecular phylogenies, and there is a defining feature for a large parasitic clade within Platyhelminthes. The parasites share an external body covering, called a syncytial tegument, or neodermis, which contrasts with the cellular ciliated epidermis of most free-living forms. Some morphological features of the free-living flatworms suggest shared ancestry with the parasitic forms. Pending resolution of the intense debate on the nature of this group of worms, we continue to present it as a phylum. Platyhelminthes is divided into four classes (see Figure 14.7): Turbellaria, Trematoda, Monogenea, and Cestoda. Class Turbellaria contains the free- living flatworms, along with some symbiotic and parasitic forms. Most turbellarians are bottom dwellers in marine or freshwater, living under stones or other hard objects. Freshwater planarians inhabit streams, pools, and even hot springs. Terrestrial flatworms are limited to moist places under stones or logs. Class Turbellaria is depicted as a paraphyletic taxon (see Section 10.4) but a large-scale revision has recently been published. Page 300 Figure 14.7 Hypothetical relationships among parasitic Platyhelminthes. The traditionally accepted class Turbellaria is paraphyletic. Some turbellarians have ectolecithal development and, together with the Trematoda, Monogenea, and Cestoda, form a clade to the exclusion of the endolecithal turbellarians. For the sake of simplicity, the synapomorphies of those turbellarians and of the Aspidogastrea, as well as many others given by Brooks (1989), are omitted. Brooks further defines a clade called Cercomeria that includes all members of Neodermata plus two turbellarian taxa not shown here. Members of Cercomeria possess a posterior adhesive organ. Hooks are present on this organ in monogeneans and cestodes. Source: Modified from D. R. Brooks. The phylogeny of the Cercomeria (Platyhelminthes: Rhabdocoela) and general evolutionary principles. Journal of Parasitology 75: 606–616, 1989. All members of classes Monogenea, Trematoda (flukes), and Cestoda (tapeworms) are parasitic. Most Monogenea are ectoparasites, but all trematodes and cestodes are endoparasitic. Many species have indirect life cycles with more than one host; the first host is often an invertebrate, and the final host is usually a vertebrate. Humans serve as hosts for a number of species. Key Theme 14.1 HUMAN CONNECTIONS Parasitic Infections Many species covered in this chapter and Chapters 11, 17, 18, 19, 20, and 21 are parasites. People have suffered greatly through the centuries from their parasites and those of their domestic animals. Fleas and bacteria conspired to destroy a third of the European population in the seventeenth century, and malaria, schistosomiasis, and African sleeping sickness have sent millions to their graves. Even today, after successful campaigns against yellow fever, malaria, and hookworm infections in many parts of the world, parasitic diseases in association with nutritional deficiencies are the primary killers of people. Civil wars and environmental changes have led to resurgences in malaria, trypanosomiasis, and leishmaniasis, and global prevalences of intestinal roundworms are unchanged in the last 50 years. Forms and Function Epidermis, Muscles Most turbellarians have a cellular, ciliated epidermis resting on a basement membrane. It contains rod-shaped rhabdites, which swell and form a protective mucous sheath around the body when discharged. Single-cell mucous glands open on the surface of the epidermis (see Figure 14.8). Most orders of turbellarians have dual-gland adhesive organs in the epidermis. These organs consist of three cell types: viscid and releasing gland cells and anchor cells (see Figure 14.9). Secretions of the viscid-gland cells apparently fasten microvilli of the anchor cells to the substrate, and secretions of the releasing-gland cells provide a quick, chemical detaching mechanism. Figure 14.8 A, Whole planarian. B, Cross section of planarian through pharyngeal region, showing relationships of body structures. Page 301 Figure 14.9 Reconstruction of dual-gland adhesive organ of the turbellarian Haplopharynx sp. There are two viscid glands and one releasing gland, which lie beneath the body wall. The anchor cell lies within the epidermis, and one of the viscid glands and the releasing gland are in contact with a nerve. In contrast to the ciliated cellular epidermis of most turbellarians, adult members of the three parasitic classes have a nonciliated body covering called a syncytial tegument (see Figure 14.10). The term syncytial means that many nuclei are enclosed within a single cell membrane. It might appear that a completely new body covering appeared in the parasitic classes, but there are some free-living turbellarians with an atypical epidermis that is syncytial, or “insunk.” Figure 14.10 Diagrammatic drawing of the structure of the tegument of a trematode Fasciola hepatica. Some turbellarians have a syncytial epidermis and others have a syncytial “insunk” epidermis where cell bodies (containing nuclei) are located beneath the basement membrane of the epidermis. Cell bodies communicate with the surface cytoplasm (distal cytoplasm) by sending extensions upward. These extensions fuse to form the syncytial covering, much as they do in syncytial tegument formation. The term “insunk” is a misnomer because the surface cytoplasm arises from upward extensions of cell bodies, not from cell bodies sinking beneath the basement membrane. Characteristics of Phylum Platyhelminthes 1. No clear defining feature 2. In marine, freshwater, and moist terrestrial habitats 3. Turbellarian flatworms are mostly free-living; classes Monogenea, Trematoda, and Cestoda entirely parasitic 4. Bilateral symmetry; definite polarity of anterior and posterior ends; body flattened dorsoventrally 5. Adult body three-layered (triploblastic) 6. Body acoelomate 7. Epidermis may be cellular or syncytial (ciliated in some); rhabdites in epidermis of most Turbellaria; epidermis a syncytial tegument in Monogenea, Trematoda, Cestoda, and some Turbellaria 8. Gut incomplete, may be branched, absent in cestodes 9. Muscular system primarily of a sheath form and of mesodermal origin; layers of circular, longitudinal, and sometimes oblique fibers beneath the epidermis 10. Nervous system consisting of a pair of anterior ganglia with longitudinal nerve cords connected by transverse nerves and located in the mesenchyme in most forms 11. Sense organs include statocysts (organs of balance) and ocelli 12. Asexual reproduction by fragmentation and other methods as part of complex parasitic life cycles 13. Most forms monoecious; reproductive system complex, usually with well-developed gonads, ducts, and accessory organs; internal fertilization; development direct in free-swimming forms and those with single hosts; complicated life cycle often involving several hosts in many internal parasites 14. Excretory system of two lateral canals with branches bearing flame cells (protonephridia); lacking in some forms 15. Respiratory, circulatory, and skeletal systems lacking; lymph channels with free cells in some trematodes Adults of all members of Trematoda, Monogenea, and Cestoda possess a syncytial covering that entirely lacks cilia and is designated a tegument (see Figure 14.10). The larval forms of many of these groups are ciliated, but the ciliated covering is shed once a host is contacted. Epidermal shedding has been suggested as a means of avoiding host immune response. Development of tegument occurs as several surface layers of the epidermis are shed; eventually fused cytoplasmic extensions from cell bodies below the basement membrane become the surface covering of the body. The tegument is sometimes called the neodermis, and its shared presence among the parasites is the basis for uniting trematodes, monogeneans, and cestodes in clade Neodermata (see Figure 14.7). The tegument is resistant to the immune system of the host in endoparasites, and it resists digestive juices in tapeworms and others that dwell in a host gut. The syncytial nature of the tegument might render it more resistant because there are no penetrable junctions between cells. The tegument can be both absorptive and secretory. The tegument of one tapeworm has been shown to release enzymes that reduce the effectiveness of the host’s digestive system. Tapeworm tegument absorbs nutrients from the host’s digestive cavity—tapeworms have neither mouth nor gut. In the body wall below the basement membrane of flatworms are layers of muscle fibers that run circularly, longitudinally, and diagonally. A meshwork of parenchyma cells, developed from mesoderm, fills the spaces between muscles and visceral organs. Parenchyma cells in some, perhaps all, flatworms are not a separate cell type but are the noncontractile portions of muscle cells. Nutrition and Digestion In general, platyhelminth digestive systems include a mouth, a pharynx, and an intestine (see Figure 14.11). In turbellarians, such as the planarian Dugesia, the pharynx is enclosed in a pharyngeal sheath (see Figure 14.11) and opens posteriorly just inside the mouth, through which it can extend. The intestine has three many-branched trunks, one anterior and two posterior. The whole forms a gastrovascular cavity lined with columnar epithelium (see Figure 14.11). Page 302 Figure 14.11 Structure of a planarian. A, Reproductive and osmoregulatory systems, shown in part. Inset at left is enlargement of flame cell. B, Digestive tract and ladder-type nervous system. Pharynx is shown in resting position. C, Pharynx extended through ventral mouth. Planarians are mainly carnivorous, feeding largely on small crustaceans, nematodes, rotifers, and insects. They can detect food from some distance by means of chemoreceptors. They entangle prey in mucous secretions from the mucous glands and rhabdites. A planarian grips prey with its anterior end, wraps its body around the prey item, extends its pharynx, and sucks up food in small amounts. Intestinal secretions contain proteolytic enzymes for some extracellular digestion. Bits of food are sucked into the intestine, where phagocytic cells of the gastrodermis complete digestion (intracellular). Undigested food is egested through the pharynx. Monogeneans and trematodes graze on host cells, feeding on cellular debris and body fluids. The mouth of trematodes and monogeneans usually opens at or near the anterior end of their body into a muscular, nonextensible pharynx (see Figures 14.12 and 14.20). Posteriorly, their esophagus opens into a blindly ending intestine, which is commonly Y- shaped but may be highly branched or unbranched, depending on the species. Figure 14.12 Structure of human liver fluke Clonorchis sinenesis. Because cestodes have no digestive tract, they must depend on host digestion, and absorption is confined to small molecules from the host’s digestive tract. Excretion and Osmoregulation Excretory systems remove wastes from the body, whereas osmoregulatory systems control water balance. Osmoregulatory systems are very common in freshwater animals where concentration gradients between internal fluids and the external environment cause bloating as water enters across the body’s permeable membranes (see Section 30.1). Excess water is typically removed via osmoregulatory systems. Sometimes osmoregulation and excretion are combined when waste products are dissolved in water shed from the body. Flatworms have a system of protonephridia (see Figure 14.11A) that could be used for excretion or osmoregulation (see Section 30.2: Nephridium). Although a small amount of ammonia is excreted via protonephridia, most metabolic wastes are largely removed by diffusion across the body wall. Flatworm protonephridia (excretory or osmoregulatory organs closed at the inner end) have flame cells (see Figure 14.11A). A flame cell is cup-shaped with a tuft of flagella extending from the inner face of the cup. In some turbellarians and in all Neodermata, the protonephridia form a weir (Old English wer, a fence placed in a stream to catch fish); the rim of the cup is elongated into fingerlike projections that extend between similar projections of a tubule cell. The space (lumen) enclosed by the tubule cell continues into collecting ducts that finally open to the outside by pores. Beating flagella (resembling a flickering flame) drive fluid down the collecting ducts and provide a negative pressure to draw fluid through the delicate interlacing projections of the weir. The wall of the duct beyond the flame cell commonly bears folds or microvilli that probably function in resorption of certain ions or molecules. In planarians, collecting ducts join and rejoin into a network along each side of the animal (see Figure 14.11) and may empty through many nephridiopores. This system is mainly osmoregulatory because it is reduced or absent in marine turbellarians, which do not have to expel excess water. Flame-cell protonephridia are present also in the parasitic taxa. Monogeneans usually have two excretory pores opening laterally, near the anterior. Collecting ducts of trematodes empty into an excretory bladder that opens to the exterior by a terminal pore (see Figure 14.12). In cestodes there are two main excretory canals on each side that are continuous through the entire length of the worm (see Figure 14.24). They join in the last segment (proglottid, see Section 14.3: Class Cestoda) to form an excretory bladder that opens by a terminal pore. When the terminal proglottid is shed, the two canals open separately. Nervous System The most primitive flatworm nervous system, found in some turbellarians, is a subepidermal nerve plexus resembling the nerve net of cnidarians. Other flatworms have, in addition to a nerve plexus, one to five pairs of longitudinal nerve cords lying under the muscle layer (see Section 33.3). Freshwater planarians have one ventral pair (see Figure 14.11B). Connecting nerves form a “ladder-type” pattern. Their brain is a bilobed mass of ganglion cells arising anteriorly from the ventral nerve cords. Neurons are organized into sensory, motor, and association types—an important development in evolution of nervous systems. Page 303 Sense Organs Active locomotion in flatworms has favored not only cephalization in the nervous system but also further evolution of sense organs. Ocelli, or light- sensitive eyespots, are common in turbellarians (see Figure 14.11A), monogeneans, and larval trematodes. Tactile cells and chemoreceptive cells are abundant over the body, and in planarians they form distinct organs on the auricles (the earlike lobes on the sides of the head). Some species also have statocysts for equilibrium and rheoreceptors for sensing direction of the water current. Sensory endings are abundant around the oral sucker of trematodes and holdfast organ (scolex; see Section 14.3: Class Cestoda) of cestodes and around genital pores in both groups. Reproduction and Regeneration Many turbellarians reproduce both asexually (by fission) and sexually. Asexually, freshwater planarians merely constrict behind the pharynx and separate into two animals, each of which regenerates the missing parts—a quick means of population increase. Reduced population density may cause an increase in the rate of fissioning. In some fissioning forms individuals may remain temporarily attached, forming chains of zooids (see Figure 14.13). Figure 14.13 Some small freshwater turbellarians. A, Phagocata has numerous pharynges. B and C, Incomplete fission results for a time in a series of attached zooids. The considerable powers of regeneration in planarians have provided an interesting system for experimental studies of development. For example, a piece excised from the middle of a planarian can regenerate both a new head and a new tail. However, the piece retains its original polarity: a head grows at the anterior end and a tail at the posterior end. An extract of heads added to a culture medium containing headless worms will prevent regeneration of new heads, suggesting that substances in one region will suppress regeneration of the same region at another level of the body. Trematodes undergo asexual reproduction in their intermediate hosts, snails. Details of their astonishing life cycles are described in Section 14.3: Class Trematoda. Some juvenile cestodes use asexual reproduction, budding off hundreds, or in some cases, even millions, of offspring (see Section 14.3: Echinococcus granulosus). Almost all flatworms are monoecious (hermaphroditic) but practice cross- fertilization. In some turbellarians the yolk for nutrition of a developing embryo is contained within the egg cell itself (endolecithal), and embryogenesis shows spiral determinate cleavage typical of protostomes (see Section 8.7: Protostome Development). Possession of endolecithal eggs is considered ancestral for flatworms. Trematodes, monogeneans, cestodes, and many of the turbellarian groups share a derived condition in which female gametes contain little or no yolk, and yolk is contributed by cells released from separate organs called vitellaria. Yolk cells are conducted toward a juncture with the oviduct by vitelline ducts (see Figures 14.11 and 14.12). Usually a number of yolk cells surround the zygote within the eggshell; thus development is ectolecithal. Cleavage is affected in such a way that a spiral pattern cannot be distinguished. The entire package consisting of yolk cells and zygote, surrounded by the eggshell, moves into the uterus and finally is released through a common genital pore or a separate uterine pore (see Figure 14.12). Access to the yolk in ectolecithal eggs is problematic for the developing embryo, but the outermost epidermal layers of some embryos grow outward to encompass the yolk. As the outermost epidermal layer is shed during development, successive inner layers enclose and utilize yolk. It has been hypothesized that the shedding of epidermal layers to permit yolk intake in ectolecithal turbellarians formed the evolutionary basis for the shedding of larval epidermal layers as the syncytial tegument forms. Male reproductive organs include one, two, or more testes connected to vasa efferentia that join to become a single vas deferens. The vas deferens commonly leads into a seminal vesicle and hence to a papilla-like penis or an extensible copulatory organ called a cirrus. During breeding season turbellarians develop both male and female organs, which usually open through a common genital pore (see Figure 14.11A). After copulation one or more fertilized eggs and some yolk cells become enclosed in a small cocoon. The cocoons are attached by little stalks to the underside of stones or plants. Embryos emerge as juveniles that resemble mature adults. In some marine forms embryos develop into ciliated free-swimming larvae very similar to the trochophore larvae of other members of Lophotrochozoa. Monogeneans hatch as free-swimming larvae that attach to the next host and develop into juveniles. Larval trematodes emerge from the eggshell as ciliated larvae that penetrate a snail intermediate host, or they may hatch only after being eaten by a snail. Most cestodes hatch only after being consumed by an intermediate host. Many different animals serve as intermediate hosts, and depending on the species, a tapeworm may require one or more specific intermediate hosts to complete the life cycle. Page 304 Class Turbellaria Turbellarians are mostly free-living worms that range in length from 5 mm or less to 50 cm. They live under objects in marine, freshwater, and terrestrial habitats. There are about six species of terrestrial turbellarians in the United States. Their mouth is on the ventral side and leads into a gut cavity, often via a pharynx. Turbellarians are often distinguished on the basis of the form of the gut (present or absent; simple or branched; pattern of branching) and pharynx (simple; folded; bulbous). Except for order Polycladida (Gr. poly, many, + klados, branch), turbellarians with endolecithal eggs have a simple gut and a simple pharynx. In a few turbellarians there is no recognizable pharynx. Polyclads have a folded pharynx and a gut with many branches (see Figure 14.14). Polyclads include many marine forms of moderate to large size (3 to more than 40 mm) (see Figure 14.15), and a highly branched intestine is correlated with larger size in turbellarians. Members of order Tricladida (Gr. treis, three, klados, branch), which are ectolecithal and include freshwater planaria, have a three-branched intestine (see Figure 14.14). Figure 14.14 Intestinal pattern of two orders of turbellarians. A, Tricladida. B, Polycladida. Figure 14.15 Pseudobiceros hancockanus, a marine polyclad turbellarian. Marine polyclads are often large and beautifully colored. This Australian species was photographed on the Great Barrier Reef. Turbellarians typically are creeping forms that combine muscular with ciliary movements to achieve locomotion. Very small planaria swim by means of their cilia. Others move by gliding, head slightly raised, over a slime track secreted by the marginal adhesive glands. Beating of epidermal cilia in the slime track moves the animal forward, while rhythmical muscular waves can be seen passing backward from the head. Large polyclads and terrestrial turbellarians crawl by muscular undulations, much in the manner of a snail. Class Trematoda Trematodes are all parasitic flukes, and as adults they are almost all found as endoparasites of vertebrates. They are chiefly leaflike in form with one or more suckers but lack the opisthaptor present in monogenean flukes (see Section 14.3: Class Monogenea). Other structural adaptations for parasitism are apparent: various penetration glands or glands to produce cyst material, organs for adhesion such as suckers and hooks, and increased reproductive capacity. Otherwise, trematodes share several characteristics with ectolecithal turbellarians, such as a well-developed alimentary canal (but with the mouth at the anterior, or cephalic, end) and similar reproductive, excretory, and nervous systems, as well as a musculature and parenchyma that are only slightly modified from those of turbellarians. Sense organs are poorly developed. Of the subclasses of Trematoda, subclass Aspidogastrea is the least well known. Most parasites in this group have only a single host, usually a mollusc. If there is a second host, it is usually a fish or turtle. Subclass Digenea (Gr. dis, double, + genos, race) is the largest and best known, with many species of medical and economic importance. Subclass Digenea With rare exceptions, digeneans have a complex life cycle, the first (intermediate) host being a mollusc and the definitive host (the host in which sexual reproduction occurs, sometimes called the final host) being a vertebrate. In some species a second, and sometimes even a third, intermediate host intervenes. The group has radiated greatly, and its members parasitize almost all kinds of vertebrate hosts. Digeneans inhabit, according to species, a wide variety of sites in their hosts: all parts of the digestive tract, respiratory tract, circulatory system, urinary tract, and reproductive tract. Among the world’s most amazing biological phenomena are digenean life cycles. Although cycles of different species vary widely in detail, a typical example would include adult, egg (shelled embryo), miracidium, sporocyst, redia, cercaria, and metacercaria stages (see Figure 14.16). The shelled embryo or larva usually passes from the definitive host in excreta and must reach water to develop further. There, it hatches to a free-swimming, ciliated larva, the miracidium. The miracidium penetrates the tissues of a snail, where it transforms into a sporocyst. Sporocysts reproduce asexually to yield either more sporocysts or a number of rediae. Rediae, in turn, reproduce asexually to produce more rediae or to produce cercariae. In this way a single egg can give rise to an enormous number of progeny. Cercariae emerge from the snail and can either penetrate the final host directly (for example, the blood fluke Schistosoma mansoni), penetrate a second intermediate host (for example, the lung fluke Paragonimus westermani), or encyst on aquatic vegetation (for example, the intestinal fluke Fasciolopsis buski). At this point, cercariae develop into metacercariae, which are essentially juvenile flukes. When the metacercariae are eaten by the final host, the juveniles migrate to the site of final infection and grow into adults. Page 305 Figure 14.16 Life cycle of Clonorchis sinensis. Some of the most serious parasites of humans and domestic animals belong to Digenea (see Table 14.1). The first digenean life cycle revealed was that of Fasciola hepatica (L. fasciola, a small bundle, band), which causes “liver rot” in sheep and other ruminants. Adult flukes live in the bile passage of the liver, and eggs are passed in feces. After hatching, a miracidium penetrates a snail to become a sporocyst. There are two generations of rediae, and the cercaria encysts on vegetation. When infested vegetation is eaten by a sheep or other ruminant (or sometimes humans), the metacercariae excyst and grow into young flukes. Page 306 TA B L E 1 4.1 Examples of Flukes Infecting Humans Common and Scientific Means of Infection; Distribution and Prevalence Names in Humans Blood flukes (Schistosoma Cercariae in water penetrate skin; 200 million spp.); three widely people infected with one or more species prevalent species, others reported S. mansoni Africa, South and Central America S. Africa haematobium Eastern Asia S. japonicum Chinese liver flukes Eating metacercariae in raw fish; about 30 million (Clonorchis sinensis) cases in eastern Asia Lung flukes (Paragonimus Eating metacercariae in raw freshwater crabs, spp.), seven species, crayfish; Asia and Oceania, sub-Saharan Africa, most prevalent is P. South and Central America; several million westermani cases in Asia Intestinal fluke Eating metacercariae on aquatic vegetation; 10 (Fasciolopsis buski) million cases in eastern Asia Sheep liver fluke (Fasciola Eating metacercariae on aquatic vegetation; hepatica) widely prevalent in sheep and cattle, occasional in humans Clonorchis sinensis: Liver Fluke in Humans Clonorchis (Gr. clon, branch, + orchis, testis) is the most important liver fluke of humans and is common in many regions of eastern Asia, especially in China, Southeast Asia, and Japan. Cats, dogs, and pigs are also often infected. Structure The worms vary from 10 to 20 mm in length (see Figure 14.12). Their structure is typical of many trematodes in most respects. They have an oral sucker and a ventral sucker. The digestive system consists of a pharynx, a muscular esophagus, and two long, unbranched intestinal ceca. The excretory system consists of two protonephridial tubules, with branches lined with flame cells. The two tubules unite to form a single median bladder that opens to the outside. The nervous system, like that of other flatworms, consists of two cerebral ganglia connected to longitudinal cords that have transverse connectives. As is common in flukes, about 80% of the body is devoted to reproduction. The reproductive system is hermaphroditic and complex. They have two branched testes that unite to form a single vas deferens, which widens into a seminal vesicle. The seminal vesicle leads into an ejaculatory duct, which terminates at the genital opening. The female system contains a branched ovary with a short oviduct, which is joined by ducts from the seminal receptacle and vitellaria at an ootype. The ootype is surrounded by a glandular mass, Mehlis’s gland, of uncertain function. From Mehlis’s gland the much-convoluted uterus runs to the genital pore. Cross- fertilization between individuals is usual, and sperm are stored in the seminal receptacle. When an oocyte is released from the ovary, it is joined by a sperm and a group of vitelline cells and is fertilized. The vitelline cells release a proteinaceous shell material, which is stabilized by a chemical reaction; the Mehlis’s gland secretions are added, and the egg passes into the uterus. Life Cycle The normal habitat of the adults is in the bile passageways of humans and other fish-eating mammals (see Figure 14.16). Eggs, each containing a complete miracidium, are shed into water with the feces but do not hatch until they are ingested by the snail Parafossarulus or related genera. Eggs, however, may live for some weeks in water. In a snail a miracidium enters the tissues and transforms into a sporocyst, which produces one generation of rediae. A redia is elongated, with an alimentary canal, a nervous system, an excretory system, and many germ cells in the process of development. Rediae pass into the liver of the snail, where the germ cells continue embryonation and give rise to tadpolelike cercariae. These two asexual stages in the intermediate host allow a single miracidium to produce as many as 250,000 infective cercariae. Cercariae escape into the water and swim until they encounter a fish of family Cyprinidae. They then bore under scales into the fish’s muscles. Here cercariae lose their tails and encyst as metacercariae. If a mammal eats raw or undercooked infected fish, the metacercarial cyst dissolves in the intestine, and young flukes apparently migrate up the bile duct, where they become adults. There the flukes may live for 15 to 30 years. The effect of these flukes on a person depends mainly on the extent of the infection but includes abdominal pain and other abdominal symptoms. A heavy infection can cause a pronounced cirrhosis of the liver and death. Cases are diagnosed through fecal examinations. Destruction of snails that carry larval stages has been used as a method of control. However, the simplest method to avoid infection is to make sure that all fish used as food is thoroughly cooked. Schistosoma: Blood Flukes Schistosomiasis, infection with blood flukes of genus Schistosoma (Gr. schistos, divided, + soma, body), ranks among the major infectious diseases in the world, with 200 million people infected. The disease is widely prevalent over much of Africa and parts of South America, the West Indies, the Middle East, and the Far East. The old generic name for the worms was Bilharzia (from Theodor Bilharz, German parasitologist who discovered Schistosoma haematobium), and the infection was called bilharziasis, a name still used in many areas. Blood flukes differ from most other flukes in being dioecious and having the two branches of the digestive tube united into a single tube in the posterior part of the body. Males are broader and heavier and have a large, ventral groove, the gynecophoric canal, posterior to the ventral sucker. The gynecophoric canal embraces the long, slender female (see Figure 14.17). Page 307 Figure 14.17 A, Adult male and female Schistosoma japonica in copulation. The male has a long gynecophoric canal that holds the female. Humans are usually hosts of adult parasites, found mainly in Africa but also in South America and elsewhere. Humans become infected by wading or bathing in cercariainfested waters. B, Life cycle of Schistosoma mansoni. Three species account for most schistosomiasis in humans: S. mansoni, which lives primarily in veins draining the large intestine; S. japonicum, which occurs mostly in veins of the small intestine; and S. haematobium, which lives in veins of the urinary bladder. Schistosoma mansoni is common in parts of Africa, Brazil, northern South America, and the West Indies; species of Biomphalaria are the principal snail intermediate hosts. Schistosoma haematobium is widely prevalent in Africa, using snails of the genera Bulinus and Physopsis as the main intermediate hosts. Schistosoma japonicum is confined to the Far East, and its hosts are several species of Oncomelania (see Section 35.3 for a discussion of immunity in snails). The life cycle of blood flukes is similar in all species. Eggs are discharged in human feces or urine; if they get into water, they hatch as ciliated miracidia, which must contact the required kind of snail within a few hours to survive. In the snail, they transform into sporocysts, which produce another generation of sporocysts. Daughter sporocysts give rise to cercariae directly, without formation of rediae. Cercariae escape from the snail and swim until they contact bare human skin. They penetrate the skin, shedding their tails in the process, and reach a blood vessel where they enter the circulatory system. There is no metacercarial stage. The young schistosomes make their way to the hepatic portal system of blood vessels and undergo a period of development in the liver before migrating to their characteristic sites. As eggs are released by adult females, they are somehow extruded through the wall of veins and through the gut or bladder lining, to be voided with feces or urine, according to species. Many eggs do not make this difficult transit and are swept by blood flow back to the liver or other areas, where they become centers of inflammation and tissue reaction. The parasite’s eggs produce the main ill effects of schistosomiasis. With S. mansoni and S. japonicum, eggs in the intestinal wall cause ulceration, abscesses, and bloody diarrhea with abdominal pain. Similarly, S. haematobium causes ulceration of the bladder wall with bloody urine and pain on urination. Eggs swept to the liver or other sites cause symptoms associated with the organs where they lodge. When they are caught in the capillary bed of the liver, they impede circulation and cause cirrhosis, a fibrotic reaction that interferes with liver function (see Figure 14.18). Of the three species, S. haematobium is considered least serious and S. japonicum most severe. The prognosis is poor in heavy infections of S. japonicum without early treatment. Page 308 Figure 14.18 This cut surface of a liver shows schistosomal hepatic fibrosis associated with the deposition of many schistosome eggs along the portal vein. Fibrosis leads to vascular obstruction, but it develops in only 5% to 10% of infected people. Researchers suspect a genetic component to the severity of response to the presence of schistosome eggs. Courtesy A. W. Cheever/From H. Zaiman, A Pictorial Presentation of Parasites. Control is best achieved by educating people to dispose of body wastes hygienically and to avoid exposure to contaminated water. These are difficult problems for poverty-stricken people living under unsanitary, crowded conditions. Key Theme 14.2 HUMAN CONNECTIONS Controlling Schistosomiasis Humans infected with schistosomiasis can be effectively treated with Praziquantel. Development of a vaccine is the subject of much research, but an effective vaccine is not yet available. Proper disposal of human wastes, snail eradication, and vector control have some promise. Biological controls include introduction of species of snails, crayfish, and fish that prey on the snail vectors. However, biological control attempts for other species have often been fraught with unexpected ecological impacts. In some cases, the biological control has been more of a problem in the long run than the pest species it was supposed to control. Many biologists consider such introductions an extreme risk that should be avoided. Schistosome Dermatitis (Swimmer’s Itch) Various species of schistosomes in several genera cause a rash or dermatitis when their cercariae penetrate hosts that are unsuitable for further development. Cercariae of several genera whose normal hosts are North American birds cause dermatitis in bathers in northern lakes. Severity of the rash increases with an increasing number of contacts with the organisms, or sensitization. After penetration, cercariae are attacked and killed by the host’s immune mechanisms, and they release allergenic substances, causing itching. The condition is more an annoyance than a serious threat to health, but there may be economic losses to vacation trade around infested lakes. Paragonimus: Lung Flukes Several species of Paragonimus (Gr. para, beside, + gonimos, generative), a fluke that lives in the lungs of its host, are known from a variety of mammals. Paragonimus westermani (see Figure 14.19) from East Asia and the southwest Pacific parasitizes a number of wild carnivores, humans, pigs, and rodents. Its eggs are coughed up in the sputum, swallowed, then eliminated with feces. Zygotes develop in the water, and the miracidium penetrates a snail host. Within the snail, miracidia give rise to sporocysts, which in turn develop into rediae. Cercariae form in rediae and are then shed into the water or ingested directly by freshwater crabs that prey on infected snails. Metacercariae develop in the crabs, and the infection is acquired by eating raw or undercooked crab meat. The infection causes respiratory symptoms, with breathing difficulties and chronic cough. Fatal cases are common. A closely related species, P. kellicotti, occurs in mink and similar animals in North America, but only one human case has been recorded. Its metacercariae live in crayfish. Figure 14.19 Lung fluke Paragonimus westermani. Adults are up to 2 cm long. Eggs discharged in sputum or feces hatch into freeswimming miracidia that enter snails. Cercariae from snails enter freshwater crabs and encyst in soft tissues. Humans are infected by eating poorly cooked crabs or by drinking water containing larvae freed from dead crabs. Some Other Trematodes Fasciolopsis buski (L. fasciola, small bundle, + Gr. opsis, appearance) parasitizes the intestine of humans and pigs in India and China. Larval stages occur in several species of planorbid snails, and cercariae encyst on water chestnuts, an aquatic plant eaten raw by both humans and pigs. Leucochloridium is noted for its remarkable sporocysts. Snails (Succinea) eat vegetation infected with eggs from bird droppings. Sporocysts become much enlarged and branched, and cercariae encyst within the sporocyst. Sporocysts enter the snail’s head and tentacles, become brightly striped with orange and green bands, and pulsate at frequent intervals. Birds are attracted by the enlarged and pulsating tentacles, eat the snails, and so complete the life cycle. Class Monogenea Monogenetic flukes were traditionally placed as an order of Trematoda, but morphological and molecular data support their placement in a different class. Cladistic analysis places them closer to the Cestoda, and some authorities now argue that cestodes and monogenean flukes are sister taxa, both having a posterior attachment organ with hooks. Monogeneans are all parasites, primarily of gills and external surfaces of fishes. A few inhabit the urinary bladder of frogs and turtles, and one parasitizes the eye of a hippopotamus. Although widespread and common, monogeneans seem to cause little damage to their hosts under natural conditions. However, like numerous other fish pathogens, they become a serious threat when their hosts are crowded together, as, for example, in fish farming. Life cycles of monogeneans are direct, with a single host. The egg hatches to produce a ciliated larva, called an oncomiracidium, that attaches to its host. The oncomiracidium bears hooks on its posterior, which in many species become the hooks on the large posterior attachment organ (opisthaptor) of the adult (see Figure 14.20). Because monogeneans must cling to the host and withstand the force of water flow over the gills or skin, adaptive diversification has produced a wide array of opisthaptors in different species. Opisthaptors may bear large and small hooks, suckers, and clamps, often in combination with each other. Page 309 Figure 14.20 A monogenetic fluke Gyrodactylus cylindriformis, ventral view. Class Cestoda Cestodes, or tapeworms, differ in many respects from the preceding classes. They usually have long, flat bodies composed of a scolex, for attachment to the host, followed by a linear series of reproductive units or proglottids (see Figure 14.21). The scolex, or holdfast, is usually provided with suckers or suckerlike organs and often with hooks or spiny tentacles as well (see Figure 14.21). Figure 14.21 A tapeworm, showing strobila and scolex. The scolex is the organ of attachment. Tapeworms entirely lack a digestive system but do have welldeveloped muscles, and their excretory system and nervous system are somewhat similar to those of other flatworms. They have no special sense organs but do have sensory endings in the tegument that are modified cilia (see Figure 14.22). Figure 14.22 Schematic drawing of a longitudinal section through a sensory ending in the tegument of Echinococcus granulosus. As in Monogenea and Trematoda, no external, motile cilia occur in adults, and the tegument is of a distal cytoplasm with sunken cell bodies beneath the superficial muscle layer (see Figure 14.22). In contrast to monogeneans and trematodes, however, the entire surface of cestodes is covered with minute projections similar to microvilli of the vertebrate small intestine (see Section 3.2: Surfaces of Cells and Their Specializations). These microtriches (sing. microthrix) greatly enlarge the surface area of the tegument, which is a vital adaptation for a tapeworm since it must absorb all its nutrients across its tegument. Subclass Eucestoda contains the great majority of species of Cestoda. The main body of tapeworms, the chain of proglottids, is called a strobila (see Figure 14.21). Typically, there is a germinative zone just behind the scolex where new proglottids are formed. As younger proglottids are differentiated in front of it, each individual proglottid moves outward in the strobila, and its gonads mature. Page 310 Unlike most other flatworms, many eucestodes are known to self-fertilize, although mutual cross-fertilization remains the norm when mates are available. Each proglottid contains a complete male and female reproductive system, and during mutual cross-fertilization, sperm from each strobila is transferred to the other. However, many tapeworms are known to double back upon themselves so that two proglottids from the same individual may fertilize one another. The shelled embryos form in the uterus of the proglottid, and they are expelled through a uterine pore, or the entire proglottid is shed from the worm as it breaks free at zones of muscle weakness between each proglottid. The tapeworm body is unusual because of the absence of many typical landmarks. There is no head. The scolex, used for attachment, is a remnant of the posterior part of the ancestral body. Cestodes and monogeneans thus share a posterior attachment organ with hooks. Some zoologists regard proglottid formation in cestodes as “true” segmentation (metamerism), but we do not support this view. Segmentation of tapeworms is best considered a replication of sex organs to increase reproductive capacity (pseudometamerism) and is not homologous to the metamerism found in Annelida, Arthropoda, and Chordata (see Section 9.2: A Complete Gut and Segmentation, and Section 17.3). More than 1000 species of tapeworms are known to parasitologists. With rare exceptions, cestodes require at least two hosts, and adults are parasites in the digestive tract of vertebrates. Often their intermediate host is an invertebrate. Collectively these animals are capable of infecting almost all vertebrate species. Normally, adult tapeworms do little harm to their hosts. The most common tapeworms found in humans are listed in Table 14.2. TA B L E 1 4. 2 Common Cestodes of Humans Common and Scientific Name Means of Infection; Prevalence in Humans Common and Scientific Name Means of Infection; Prevalence in Humans Beef tapeworm Eating rare beef; most common of all tapeworms in (Taenia saginata) humans Pork tapeworm Eating rare pork; less common than T. saginata (Taenia solium) Fish tapeworm Eating rare or poorly cooked fish; fairly common in Great (Diphyllobothrium Lakes region of United States, and other areas of latum) world where raw fish is eaten Dog tapeworm Unhygienic habits of children ( juveniles in flea and (Dipylidium louse); moderate frequency caninum) Dwarf tapeworm Juveniles in flour beetles; common (Hymenolepis nana) Unilocular hydatid Cysts of juveniles in humans; infection by contact with (Echinococcus dogs; common wherever humans are in close granulosus) relationship with dogs and ruminants Multilocular hydatid Cysts of juveniles in humans; infection by contact with (Echinococcus foxes; less common than unilocular hydatid multilocularis) Key Theme 14.3 HUMAN CONNECTIONS Gutless Wonder Though lacking skeletal strengths Which we associate with most Large forms, tapeworms go to great Lengths to take the measure of a host. Monotonous body sections In a limp mass-production line Have nervous and excretory connections And the means to sexually combine And to coddle countless progeny But no longer have the guts To digest for themselves or live free Or know a meal from soup to nuts. Copyright 1975 by John M. Burns. Reprinted by permission of the author from BioGraffiti: A Natural Selection by John M. Burns. Published hardbound by Quadrangle/The New York Times Book Co., 1975. Republished as a paperback by W. W. Norton & Company, Inc., 1981. Taenia saginata: Beef Tapeworm Structure Taenia saginata (Gr. tainia, band, ribbon) is called the beef tapeworm, but it lives as an adult in the human intestine. Juvenile forms occur primarily in intermuscular tissue of cattle. A mature adult may reach a length of 10 m or more. Its scolex has four suckers for attachment to the intestinal wall, but no hooks. A short neck connects the scolex to the strobila, which may have as many as 2000 proglottids. Gravid proglottids bear shelled, infective larvae (see Figure 14.23), which become detached and pass in feces. Page 311 Figure 14.23 Life cycle of beef tapeworm, Taenia saginata. Ripe proglottids detach in the human intestine, leave the body in feces, crawl onto grass, and are ingested by cattle. Eggs hatch in the cow’s intestine, freeing oncospheres, which penetrate into muscles and encyst, developing into “bladder worms.” A human eats infected rare beef, and cysticercus is freed in the intestine where it attaches to the intestinal wall, forms a strobila, and matures. Although tapeworms lack true metamerism, there is repetition of the reproductive and excretory systems in each proglottid. Excretory canals in the scolex are continued the length of the body by a pair of dorsolateral, and a pair of ventrolateral, excretory canals. These paired canals are connected by a transverse canal near the distal end of each proglottid. Two longitudinal nerve cords from a nerve ring in the scolex also run back through each proglottid (see Figure 14.24). Attached to the excretory ducts are flame cells. Each mature proglottid also contains muscles and parenchyma as well as a complete set of male and female organs similar to those of a trematode. Page 312 Figure 14.24 A, Two mature proglottids of Taenia pisiformis, a dog tapeworm. B, More anatomical details are marked in a drawing of two complete proglottids, two other partial proglottids are shown. In this group of tapeworms, vitellaria are typically a single, compact vitelline gland located just posterior to the ovaries. When gravid proglottids break off and pass out with the feces, they usually crawl out of the fecal mass and onto vegetation nearby. There they may be eaten by grazing cattle. A proglottid ruptures as it dries, further scattering the embryos on soil and grass. Embryos may remain viable on grass for as long as 5 months. Life Cycle Shelled larvae (oncospheres) swallowed by cattle hatch and use their hooks to burrow through the intestinal wall into blood or lymph vessels and finally reach voluntary muscle, where they encyst to become bladder worms (juveniles called cysticerci). There the juveniles develop an invaginated scolex but remain quiescent. When raw or undercooked “measly” meat is eaten by a suitable host, the cyst wall dissolves, the scolex evaginates and attaches to the intestinal mucosa, and new proglottids begin to develop. It takes 2 to 3 weeks for a mature worm to form. When a person is infected with one of these tapeworms, numerous gravid proglottids are expelled daily, sometimes even crawling out the anus. Humans become infected by eating rare beef, steaks, and barbecues. Considering that about 1% of American cattle are infected, that 20% of all cattle slaughtered are not federally inspected, and that even in inspected meat one-fourth of infections are missed, it is not surprising that tapeworm infection is fairly common. Infection is easily avoided when meat is thoroughly cooked. Some Other Tapeworms Taenia solium: Pork Tapeworm The tapeworm Taenia solium (Gr. tainia, band, ribbon) uses humans as the definitive host and pigs as the intermediate host. Adult tapeworms live in the human gut, and infected humans shed fertilized tapeworm eggs with their feces. Pigs that consume infected human fecal material are colonized by the worms. Inside a pig, tapeworm larvae form cysts in muscle tissue. When humans consume infected undercooked pork, the cysts hatch and develop into adult tapeworms; this is the most common mode of infection. However, there is another way that humans become infected: people who live with, or contact, other people infected with tapeworms may develop cysticercosis, a serious disease caused by ingesting the fertilized eggs directly, without any intermediate host. When fertilized eggs are ingested by a human, instead of by a pig, the resulting larvae migrate to tissues such as the brain, spinal cord, liver, muscles, or eyes. Infection of the brain or spinal cord is very serious and may cause death (see Figure 14.25). Infection of other organs may be treatable. Figure 14.25 Section through the brain of a person who died of cerebral cysticercosis, an infection with cysticerci of Taenia solium. Diphyllobothrium latum: Fish Tapeworm Adult Diphyllobothrium (Gr. dis, double, + phyllon, leaf, + bothrion, hole, trench) live in the intestines of humans, dogs, cats, and other mammals; immature stages develop in crustaceans and fish. With a length up to 20 m, it is the largest cestode that infects humans. Fish tapeworm infections can occur anywhere in the world where people commonly eat raw fish; in the United States infections are most common in the Great Lakes region. In Finland the worm can cause a serious anemia not apparent in other areas. Echinococcus granulosus: Unilocular Hydatid Echinococcus granulosus (Gr. echinos, hedgehog, + kokkos, kernel) (see Figure 14.26B), a dog tapeworm, causes hydatidosis, a very serious human disease in many parts of the world. Adult worms develop in canines, and juveniles grow in more than 40 species of mammals, including humans, monkeys, sheep, reindeer, and cattle. Thus humans may serve as a dead-end host for this tapeworm. The juvenile stage is a special kind of cysticercus called a hydatid cyst (Gr. hydatis, watery vesicle). It grows slowly but for a long time—up to 20 years—reaching the size of a basketball in an unrestricted site such as the liver. If a hydatid grows in a critical location, such as the heart or central nervous system, serious symptoms may appear sooner. The main cyst maintains a single (or unilocular) chamber, but daughter cysts bud off, and each contains thousands of scolices. Each scolex produces a worm only when eaten by a canine. Treatment options include chemotherapies and surgical removal of the hydatid. Page 313 Page 338 16 Molluscs Fluted giant clam, Tridacna maxima. ©Larry Roberts/McGraw-Hill Education LEARNING OBJECTIVES Readers will be able to: 16.1 Explain the biological and economic impact of ocean acidification on mollusc growth and harvest. 16.2 Describe the key features of the molluscan body plan. 16.3 Compare and contrast the molluscan body plan across eight classes. 16.4 Discuss the evidence for a non-segmented molluscan ancestor and for the independent evolution of segmentation in animals. A Shell-Collector’s Delight Molluscs are astonishingly diverse. The group includes wormlike animals and giant squids, as well as animals with a single shell, two shells, eight shell plates, and no shell at all. Some have wondered whether the molluscs really form a clade, but there are several features present in almost all molluscs that suggest shared ancestry. Most molluscs have an unusual ribbon of teeth called a radula that they use to feed, often scraping algae from hard surfaces. Most molluscs have a large muscular foot used in locomotion and a unique tissue layer called a mantle. The mantle secretes the shell and makes the respiratory and sensory organs, among other things. These common molluscan features are employed in very different ways across the eight classes of molluscs: for example, the radular teeth are used to inject a paralyzing venom in some species, whereas another group has lost the radula, along with its head. Snails crawl on the foot, whereas octopi divide it into muscular, prehensile arms. Humans have exploited this diversity in many ways. Shells were used as money on almost every continent—the money cowry is a snail whose shell was widely used as currency. There is debate as to whether the first use of shells was as currency or adornment, but shells are still used in jewelry, buttons, and decoration throughout the world. Pearls are another important product of bivalves. By far the most common use of molluscs is as food. We eat clams, oysters, scallops, mussels, snails, abalone, squids, and octopi, to name just a few of the molluscs exploited commercially. Sometimes we eat the mantle, sometimes the foot, and other times the entire body. Our dependence on these animals behooves us to be good stewards of the environment for molluscs by maintaining unpolluted riverine, coastal, and oceanic habitats and by developing sustainable harvest practices. PHYLUM MOLLUSCA Page 339 16.1 MOLLUSCS Mollusca (mol-lus′ka) (L. molluscus, soft) is one of the largest animal phyla after Arthropoda. There are over 90,000 living species and some 70,000 fossil species. Molluscs are coelomate lophotrochozoan protostomes, and as such they develop via spiral mosaic cleavage and make a coelom by schizocoely. The ancestral larval stage is a trochophore, but development is variously modified within the classes. The name Mollusca indicates one of their distinctive characteristics, a soft body. This very diverse group (see Figure 16.1) includes chitons, tusk shells, snails, slugs, nudibranchs, sea butterflies, clams, mussels, oysters, squids, octopuses, and nautiluses. The group ranges from fairly simple organisms to some of the most complex of invertebrates; sizes range from almost microscopic to the giant squid Architeuthis. These huge molluscs may grow to nearly 20 m long, including their tentacles. They may weigh up to 900 kg (1980 pounds). The shells of some giant clams, Tridacna gigas, which inhabit Indo-Pacific coral reefs, reach 1.5 m in length and weigh more than 250 kg. These are extremes, however, for probably 80% of all molluscs are less than 10 cm in maximum shell size. The phylum includes some of the most sluggish and some of the swiftest and most active invertebrates. It includes herbivorous grazers, predaceous carnivores, filter feeders, detritus feeders, and parasites. Figure 16.1 Molluscs: a diversity of lifeforms. The basic body plan of this ancient group has become variously adapted for different habitats. A, A chiton (Tonicella lineata), class Polyplacophora. B, A marine snail (Calliostoma), class Gastropoda. C, A nudibranch (Chromodoris sp.), class Gastropoda. D, Geoduck clams from Puget Sound, Washington, extend their large siphons, class Bivalvia. E, An octopus (Octopus briareus), class Cephalopoda, forages at night on a Caribbean coral reef. Molluscs occupy a great range of habitats, from the tropics to polar seas, at altitudes exceeding 7000 m, in ponds, lakes, and streams, on mud flats, in pounding surf, and in open ocean from the surface to abyssal depths. They represent a variety of lifestyles, including bottom feeders, burrowers, borers, and pelagic forms. According to fossil evidence, molluscs originated in the sea, and most of them have remained there. Much of their evolution occurred along the shores, where food was abundant and habitats were varied. Only bivalves and gastropods moved into brackish and freshwater habitats. As filter feeders, bivalves were unable to leave aquatic surroundings. Only slugs and snails (gastropods) actually invaded the land. Terrestrial snails are limited in their range by their need for humidity, shelter, and presence of calcium in the soil. Molluscs are an extremely important food source for people around the world; in 2006, 450,687,000 tonnes of molluscs were harvested commercially on the eastern seaboard of the US, with the western seaboard and the Gulf of Mexico adding 3,870,000 and 44,537,000 tonnes, respectively. The value of the eastern seaboard catch alone was nearly $6.28 billion dollars1, so the economic importance of molluscs cannot be overstated. A healthy mollusc “fishery” depends on healthy oceans, but recent threats to mollusc populations come from an unexpected direction: ocean acidification. Increased amounts of CO2 in the atmosphere initiate a set of chemical reactions in the oceans that lower pH. As the ocean becomes more acidic, levels of biologically available calcium decline, making it more difficult for marine organisms to form calcium skeletons. Calcium is essential for the foundation beneath living coral tissue (see Section 13.1: Coral Reefs), and is critical to healthy mollusc shells. Clams, oysters, mussels, and scallops produce thinner and weaker shells when reared at low pH. Similarly, larval abalone fail to develop normally, and many cannot create normal shells. Larval survivorship for oysters is reduced when ocean acidity increases, so the oyster industry in the Pacific Northwest is concerned about its $278 million dollar revenue (2009 data). One Oregon oyster farm adds calcium chloride and sodium carbonate to seawater to increase the amount of biologically available calcium for young animals. The one bright spot in the future of mollusc harvesting lies with cephalopods such as squid and octopus. They appear to be much less affected by ocean acidification than their kin, presumably because they do not have shells. Page 340 Research has shown direct adverse effects of ocean acidification on coral settlement and growth, and on the neurological development of larval tropical reef fishes, but indirect damage to the coral reef ecosystem is equally worrying. The complex food webs (see Section 13.1: Coral Reefs) present on the reef will change with the distribution and abundance of corals and coralline algae. If acidification leads to increased abundance of algal turfs and sea grass beds, community changes may act on those molluscs and other taxa not directly impacted by the acidification of our oceans. In this chapter we explore the various major groups of molluscs (see Figure 16.2), including those groups having limited diversity (classes Caudofoveata, Solenogastres, Monoplacophora, and Scaphopoda). Members of class Polyplacophora (chitons) are common to abundant marine animals, especially in the intertidal zone. Bivalves (class Bivalvia) have evolved many species, both marine and freshwater. Class Cephalopoda (squids, cuttlefish, octopuses, and their kin) contains the largest and most intelligent of all invertebrates. Most abundant and widespread of molluscs, however, are snails and their relatives (class Gastropoda). Although enormously diverse, molluscs have in common a basic body plan (see Section 16.2). The coelom in molluscs is limited to a space around the heart, and perhaps around the gonads and part of the kidneys. Although it develops embryonically in a manner similar to the coelom of annelids (see Chapter 17), the functional consequences of this space are quite different because it is not used in locomotion. Figure 16.2 Cladogram showing hypothetical relationships among classes of Mollusca. Synapomorphies that identify the various clades are shown, although a number of these have been modified or lost in some descendants. For example, the univalve shell (as well as shell coiling) has been reduced or lost in many gastropods and cephalopods, and many gastropods have undergone detorsion. The bivalve shell of the Bivalvia was derived from an ancestral univalve shell. The byssus is not present in most adult bivalves but functions in larval attachment in many; therefore the byssus is considered a synapomorphy of Bivalvia. Page 341 16.2 FORM AND FUNCTION The enormous variety, great beauty, and easy availability of shells of molluscs have made shell collecting a popular pastime. However, many amateur shell collectors, even though able to name hundreds of the shells that grace our beaches, know very little about the living animals that created those shells and once occupied them. Reduced to its simplest dimensions, the mollusc body plan has a head-foot portion and a visceral mass portion (see Figure 16.3). The head-foot is the more active area, containing the feeding, cephalic sensory, and locomotor organs. It depends primarily on muscular action for its function. The visceral mass is the portion containing digestive, circulatory, respiratory, and reproductive organs, and it depends primarily on ciliary tracts for its functioning. Two folds of skin, outgrowths of the dorsal body wall, form a protective mantle, which encloses a space between the mantle and body wall called the mantle cavity. The mantle cavity houses gills (ctenidia) or a lung, and in some molluscs the mantle secretes a protective shell over the visceral mass. Modifications of the structures that form the head-foot and the visceral mass produce the great diversity of patterns observed in Mollusca. Greater emphasis on either the headfoot portion or the visceral mass portion can be observed in various classes of molluscs. Figure 16.3 Generalized mollusc. Although this construct is often presented as a “hypothetical ancestral mollusc (HAM),” most experts now reject this interpretation. Such a diagram is useful, however, to facilitate description of the general body plan of molluscs. Head-Foot Most molluscs have well-developed heads, which bear their mouth and some specialized structures such as sensory tentacles and photoreceptors. Photoreceptors may be simple, but some, such as the eyes of cephalopods, are quite complex. Within the mouth is a structure unique to molluscs, the radula, and usually posterior to the mouth is the chief locomotor organ, or foot. Page 342 Radula The radula is a rasping, protrusible, tonguelike organ found in all molluscs except bivalves and most solenogasters. It is a ribbonlike membrane bearing a surface of tiny, backward-pointing teeth used for feeding (see Figure 16.4). Complex muscles move the radula and its supporting cartilages (odontophore) in and out of the mouth while the membrane is partly rotated over the tips of the cartilages. There may be a few or as many as 250,000 teeth, which, when protruded, can scrape, pierce, tear, or cut. The usual function of the radula is twofold: to rasp fine particles of food material from hard surfaces and to serve as a conveyor belt for carrying particles in a continuous stream toward the digestive tract. As the radula wears away anteriorly, new rows of teeth are continuously replaced by secretion at its posterior end. The pattern and number of teeth in a row are specific for each species and are used in the classification of molluscs. Very interesting radular specializations, such as for boring through hard materials or for harpooning prey, occur in some forms. Figure 16.4 A, Diagrammatic longitudinal section of a gastropod head showing a radula and radula sac. The radula moves back and forth over the odontophore cartilage. As the animal grazes, the mouth opens, the odontophore is thrust forward, the radula gives a strong scrape backward bringing food into the pharynx, and the mouth closes. The sequence is repeated rhythmically. As the radula ribbon wears out anteriorly, it is continually replaced posteriorly. B, Radula of a snail prepared for microscopic examination. Characteristics of Phylum Mollusca 1. Dorsal body wall forms pair of folds called the mantle, which encloses the mantle cavity, is modified into gills or lungs, and secretes the shell (shell absent in some); ventral body wall specialized as a muscular foot, variously modified but used chiefly for locomotion; radula in mouth 2. Live in marine, freshwater, and terrestrial habitats 3. Free-living or occasionally parasitic 4. Body bilaterally symmetrical (bilateral asymmetry in some); unsegmented; often with definite head 5. Triploblastic body 6. Coelom limited mainly to area around heart, and perhaps lumen of gonads, part of kidneys, and occasionally part of the intestine 7. Surface epithelium usually ciliated and bearing mucous glands and sensory nerve endings 8. Complex digestive system; rasping organ (radula) usually present; anus usually emptying into mantle cavity; internal and external ciliary tracts often of great functional importance 9. Circular, diagonal, and longitudinal muscles in the body wall; mantle and foot highly muscular in some classes (for example, cephalopods and gastropods) 10. Nervous system of paired cerebral, pleural, pedal, and visceral ganglia, with nerve cords and subepidermal plexus; ganglia centralized in nerve ring in gastropods and cephalopods 11. Sensory organs of touch, smell, taste, equilibrium, and vision (in some); the highly developed direct eye (photosensitive cells in retina face light source) of cephalopods is similar to the indirect eye (photosensitive cells face away from light source) of vertebrates but arises as a skin derivative in contrast to the brain eye of vertebrates 12. No asexual reproduction 13. Both monoecious and dioecious forms; spiral cleavage; ancestral larva a trochophore, many with a veliger larva, some with direct development 14. One or two kidneys (metanephridia) opening into the pericardial cavity and usually emptying into the mantle cavity 15. Gaseous exchange by gills, lungs, mantle, or body surface 16. Open circulatory system (secondarily closed in cephalopods) of heart (usually three chambered), blood vessels, and sinuses; respiratory pigments in blood Foot The molluscan foot (see Figure 16.3) may be variously adapted for locomotion, for attachment to a substratum, or for a combination of functions. It is usually a ventral, sole-like structure in which waves of muscular contraction effect a creeping locomotion. However, there are many modifications, such as the attachment disc of limpets, the laterally compressed “hatchet foot” of bivalves, or the siphon for jet propulsion in squids and octopuses. Secreted mucus is often used as an aid to adhesion or as a slime tract by small molluscs that glide on cilia. In snails and bivalves the foot extends from the body hydraulically, by engorgement with blood. Burrowing forms can extend the foot into the mud or sand, enlarge it with blood pressure, then use the engorged foot as an anchor to draw the body forward. In pelagic (free-swimming) forms the foot may be modified into winglike parapodia, or thin, mobile fins for swimming. Visceral Mass Mantle and Mantle Cavity The mantle is a sheath of skin, extending from the visceral mass, that hangs down on each side of the body, protecting the soft parts and creating between itself and the visceral mass a space called the mantle cavity. The outer surface of the mantle secretes the shell. The mantle cavity (see Figure 16.3) plays an enormous role in the life of a mollusc. It usually houses respiratory organs (gills or lung), which develop from the mantle, and the mantle’s own exposed surface serves also for gaseous exchange. Products from the digestive, excretory, and reproductive systems are emptied into the mantle cavity. In aquatic molluscs a continuous current of water, kept moving by surface cilia or by muscular pumping, brings in oxygen and, in some forms, food. This same water current also flushes out wastes and carries reproductive products out to the environment. In aquatic forms the mantle is usually equipped with sensory receptors for sampling environmental water. In cephalopods (squids and octopuses) the muscular mantle and its cavity create jet propulsion used in locomotion. Many molluscs can withdraw their head or foot into the mantle cavity, which is surrounded by the shell, for protection. In the simplest form, a mollusc ctenidium (gill) consists of a long, flattened axis extending from the wall of the mantle cavity (see Figure 16.5). Many leaflike gill filaments project from the central axis. Water is propelled by cilia between gill filaments, and blood diffuses from an afferent vessel in the central axis through the filament to an efferent vessel. Direction of blood movement is opposite to the direction of water movement, thus establishing a countercurrent exchange mechanism (see Section 31.4: Efficient Exchange in Water: Gills.). The two ctenidia are located on opposite sides of the mantle cavity and are arranged so that the cavity is functionally divided into an incurrent chamber and an excurrent chamber. The basic arrangement of gills is variously modified in many molluscs. Figure 16.5 Primitive condition of mollusc gill (ctenidium). Circulation of water between gill filaments is by cilia, and blood diffuses through the filament from the afferent vessel to the efferent vessel. Black arrows are ciliary cleansing currents. Red arrows indicate blood flow. Page 343 Shell The shell of a mollusc, when present, is secreted by the mantle and is lined by it. Typically there are three layers (see Figure 16.6A). The periostracum is the outer organic layer, composed of an organic substance called conchiolin, which consists of quinone-tanned protein. It helps to protect underlying calcareous layers from erosion by boring organisms. It is secreted by a fold of the mantle edge, and growth occurs only at the margin of the shell. On the older parts of the shell, periostracum often becomes worn away. The middle prismatic layer is composed of densely packed prisms of calcium carbonate (either aragonite or calcite) laid down in a protein matrix. It is secreted by the glandular margin of the mantle, and increase in shell size occurs at the shell margin as the animal grows. The inner nacreous layer of the shell lies next to the mantle and is secreted continuously by the mantle surface, so that it increases in thickness during the life of the animal. The calcareous nacre is laid down in thin layers. Very thin and wavy layers produce the iridescent mother-of-pearl found in abalones (Haliotis), chambered nautiluses (Nautilus), and many bivalves. Such shells may have 450 to 5000 fine parallel layers of crystalline calcium carbonate for each centimeter of thickness. Figure 16.6 A, Diagrammatic vertical section of shell and mantle of a bivalve. The outer mantle epithelium secretes the shell; the inner epithelium is usually ciliated. B, Formation of pearl between mantle and shell as a parasite or bit of sand under the mantle becomes covered with nacre. There is great variation in shell structure among molluscs. Freshwater molluscs usually have a thick periostracum that gives some protection against acids produced in the water by decay of leaf litter. In many marine molluscs the periostracum is relatively thin, and in some it is absent. Calcium for the shell comes from environmental water or soil or from food. The first shell appears during the larval period and grows continuously throughout life. Internal Structure and Function Gas exchange occurs in specialized respiratory organs such as ctenidia, secondary gills and lungs, as well as the body surface, particularly the mantle. There is an open circulatory system with a pumping heart, blood vessels, and blood sinuses. In an open circulatory system blood is not entirely contained within blood vessels; rather, it flows through vessels in some parts of the body and enters open sinuses in other parts (see Section 31.3). An open circulatory system is less efficient at supplying oxygen to all tissues in the body, so it is common in slow-moving animals. Insects are a notable exception, but in these animals oxygen is distributed by the tracheal system, not by the circulatory system. In a closed circulatory system, blood moves to and from tissues within blood vessels. Most cephalopods have a closed circulatory system with vessels and capillaries. The digestive tract is complex and highly specialized, according to feeding habits of the various molluscs, and is usually equipped with extensive ciliary tracts. Most molluscs have a pair of kidneys (metanephridia, a type of nephridium in which the inner end opens into the coelom by a nephrostome; see Section 30.2). Ducts of the kidneys in many forms also serve for discharge of eggs and sperm. The nervous system consists of several pairs of ganglia with connecting nerve cords, and it is generally simpler than that of annelids and arthropods. The nervous system contains neurosecretory cells that, at least in certain air- breathing snails, produce a growth hormone and function in osmoregulation. There are various types of highly specialized sense organs. Reproduction and Life History Most molluscs are dioecious, although some are hermaphroditic. The free- swimming trochophore larva that emerges from the egg in many molluscs (see Figure 16.7) is remarkably similar to that seen in annelids. Direct metamorphosis of a trochophore into a small juvenile, as in chitons, is considered ancestral for molluscs. However, in many molluscan groups (especially gastropods and bivalves) the trochophore stage gives rise to a uniquely molluscan larval stage called a veliger. The free-swimming veliger (see Figure 16.8) has the beginnings of a foot, shell, and mantle. In many molluscs the trochophore stage occurs in the egg, and a veliger hatches to become the only free-swimming stage. Cephalopods, some freshwater bivalves, and freshwater and some marine snails have no free-swimming larvae; instead, juveniles hatch directly from eggs. Page 344 Figure 16.7 A, Generalized trochophore larva. Molluscs and annelids with an ancestral pattern of embryonic development have trochophore larvae, as do several other phyla. B, Trochophore of a Christmas tree worm, Spirobranches spinosus (Annelida). Figure 16.8 Veliger of a snail, Pedicularia, swimming. The adults are parasitic on corals. The ciliated process (velum) develops from the prototroch of the trochophore (see Figure 16.7). Key Theme 16.1 GENETICS AND DEVELOPMENT Trochophore Larvae Trochophore larvae (see Figure 16.7) are minute, translucent, and more or less top-shaped, and have a prominent circlet of cilia (prototroch) and sometimes one or two accessory circlets. They characterize molluscs and annelids exhibiting the ancestral embryonic development pattern and are usually considered homologous between the two phyla. Some form of trochophorelike larva also characterizes marine turbellarians, nemertines, brachiopods, phoronids, sipunculids, and echiurids, and together with molecular evidence, it supports a taxonomic group called Trochozoa within superphylum Lophotrochozoa. 16.3 CLASSES OF MOLLUSCS For more than 50 years taxonomists recognized five classes of living molluscs: Amphineura, Gastropoda, Scaphopoda, Bivalvia (also called Pelecypoda), and Cephalopoda. Discovery of Neopilina in the 1950s added another class (Monoplacophora), and Hyman2 contended that solenogasters and chitons were separate classes (Aplacophora and Polyplacophora), lapsing the name Amphineura. Subsequently, Aplacophora was divided into the sister groups Caudofoveata and Solenogastres.3 Members of both groups are wormlike and shell-less with calcareous sclerites or spicules in their integument. They have reduced heads and lack nephridia. In spite of these similarities, there are important differences between groups. Class Caudofoveata Members of class Caudofoveata comprise about 120 species of wormlike, marine organisms ranging from 2 to 140 mm in length (see Figure 16.9). They are mostly burrowers and orient themselves vertically, with the terminal mantle cavity and gills at the entrance of the burrow. They feed primarily on microorganisms and detritus. They possess an oral shield, an organ apparently associated with food selection and intake, as well as a radula. They have one pair of gills. They are dioecious. The body plan of caudofoveates may have more features in common with the ancestor of molluscs than any other living group. This class is sometimes called Chaetodermomorpha. Page 345 Figure 16.9 Spicules are clearly visible on the skin of solenogasters and caudofoveates. A, Neomeniomorpha, a solenogaster. B, Chaetoderma elegans, a caudofoveate. Class Solenogastres Solenogasters (see Figure 16.9) are a small group of about 250 species of marine animals similar to caudofoveates. Solenogasters, however, usually have no radula and no gills (although secondary respiratory structures may be present). Their foot is represented by a midventral, narrow furrow, the pedal groove. They are hermaphroditic. Solenogasters are bottom-dwellers, and often live and feed on cnidarians. This class is sometimes called Neomeniomorpha. Class Polyplacophora: Chitons Chitons (Gr. coat of mail, tunic) (see Figure 16.10) represent a somewhat more diverse molluscan group with about 1000 currently described species. They are rather flattened dorsoventrally and have a convex dorsal surface that bears seven or eight articulating limy plates, or valves; hence their name Polyplacophora (“many plate bearers”). The plates overlap posteriorly and are usually dull colored to match the rocks to which chitons cling. Their head and cephalic sensory organs are reduced, but photosensitive structures (esthetes), which have the form of eyes in some chitons, pierce the plates. Figure 16.10 Lined chiton, Tonicella lineata. The upper surface of the mantle, or “girdle,” is smooth, but in other genera the girdle is covered with hairs and bristles, an adaptation for defense. Most chitons are small (2 to 5 cm); the largest, Cryptochiton (Gr. crypto, hidden, + chiton, coat of mail), rarely exceeds 30 cm. They prefer rocky surfaces in intertidal regions, although some live at great depths. Most chitons are stay-at-home organisms, straying only very short distances for feeding. Most feed by projecting the radula outward from the mouth to scrape algae from rocks. Scraping is aided by radular teeth reinforced with the iron- containing mineral, magnetite. However, the chiton Placiphorella velata is an unusual predatory species that uses a specialized head flap to capture small invertebrate prey. A chiton clings tenaciously to its rock with its broad, flat foot. If detached, it can roll up like an armadillo for protection. The mantle forms a girdle around the margin of the plates, and in some species mantle folds cover part or all of the plates. Compared with other molluscan classes, the mantle cavity of polyplacophorans is extended along the side of the foot, and the gills are more numerous. The gills are suspended from the roof of the mantle cavity along each side of the broad ventral foot (see Figure 16.11). With the foot and the mantle margin adhering tightly to the substrate, these grooves become closed chambers, open only at the ends. Water enters the grooves anteriorly, flows across the gills bringing a continuous supply of oxygen, and leaves posteriorly. At low tide the margins of the mantle can be tightly pressed to the substratum to diminish water loss, but in some circumstances, the mantle margins can be held open for limited air breathing. A pair of osphradia (chemoreceptive sense organs for sampling water) occupy the mantle grooves near the anus of many chitons. Page 346 Figure 16.11 Anatomy of a chiton (class Polyplacophora). A, Longitudinal section. B, Transverse section. C, External ventral view. Blood pumped by the three-chambered heart reaches the gills by way of an aorta and sinuses. A pair of kidneys (metanephridia) carries waste from the pericardial cavity to the exterior. Two pairs of longitudinal nerve cords connect in the buccal region. Sexes are separate in most chitons, and trochophore larvae metamorphose directly into juveniles, without an intervening veliger stage. Class Monoplacophora Monoplacophora were long thought to be extinct; they were known only from Paleozoic shells. However, in 1952 living specimens of Neopilina (Gr. neo, new, + pilos, felt cap) were dredged up from the seafloor near the west coast of Costa Rica. About 25 species of monoplacophorans are now known. These molluscs are small and have a low, rounded shell and a creeping foot (see Figure 16.12). The mouth bears a characteristic radula. They superficially resemble limpets, but unlike most other molluscs, have some serially repeated organs. These animals have three to six pairs of gills, two pairs of heart atria, three to seven pairs of metanephridia, one or two pairs of gonads, and a ladderlike nervous system with 10 pairs of pedal nerves. Such serial repetition occurs to a more limited extent in chitons. Why should there be repeated sets of body structures in these animals? Body structures repeat in each segment of an annelid worm (see Chapter 17, opening essay). Are the repeated structures indications that molluscs had a segmented (metameric) ancestor? Most current research indicates that Neopilina shows pseudometamerism, and that molluscs did not have a metameric ancestor. The Serialia hypothesis (see Section 16.4) proposes monoplacophorans and polyplacophorans as sister taxa, where serially repeated structures evolved in the ancestor of these two groups. Figure 16.12 Neopilina, class Monoplacophora. Living specimens range from 3 mm to about 3 cm in length. A, Ventral view. B, Dorsal view. Class Gastropoda Among molluscs, class Gastropoda is by far the largest and most diverse, containing over 70,000 living and more than 15,000 fossil species. It contains so much diversity that there is no single general term in our language that can apply to it. It contains snails, limpets, slugs, whelks, conchs, periwinkles, sea slugs, sea hares, and sea butterflies. These forms range from marine molluscs to terrestrial, air-breathing snails and slugs. Gastropods are usually sluggish, sedentary animals because most of them have heavy shells and slow locomotion. Some are specialized for climbing, swimming, or burrowing. Shells are their chief defense. The shell, when present, is always of one piece (univalve) and may be coiled or uncoiled. Starting at the apex, which contains the oldest and smallest whorl, the whorls become successively larger and spiral around the central axis, or columella (see Figure 16.13). The shell may be right handed (dextral) or left handed (sinistral), depending on the direction of coiling. Direction of coiling is genetically controlled and dextral shells are far more common. Many snails have an operculum, a plate made of tanned protein that covers the shell aperture when the body is withdrawn into the shell. Page 347 Figure 16.13 Shell of the whelk Busycon. A and B, Busycon carica, a dextral, or right- handed, shell. A dextral shell has the aperture on the right side when the shell is held with the apex up and the aperture facing the observer. C, B. contrarium, a sinistral, or left-handed, shell. Gastropods range from microscopic forms to giant marine forms such as Pleuroploca gigantea, a snail with a shell up to 60 cm long, and sea hares, Aplysia (see Figure 16.22), some species of which reach 1 m in length. Most gastropods, however, are between 1 and 8 cm in length. Some fossil species are as much as 2 m long. The range of gastropod habitats is large. Marine gastropods are common both in littoral zones and at great depths, and some are even pelagic. Some are adapted to brackish water and others to fresh water. On land they are restricted by such factors as mineral content of the soil and extremes of temperature, dryness, and acidity. Even so, they are widespread; some occur at great altitudes and some even in polar regions. Snails occupy all kinds of habitats: in small pools or large bodies of water, in woodlands, in pastures, under rocks, in mosses, on cliffs, in trees, underground, and on the bodies of other animals. They have successfully undertaken every mode of life except aerial locomotion. Gastropods may be protected by shells, by distasteful or toxic secretions, and/or by secretive habits. Some species are even capable of deploying the stinging cells of their cnidarian prey for their own defense. A few, such as Strombus, can deal an active blow with their foot, which bears a sharp operculum. Nevertheless, they are eaten by birds, beetles, small mammals, fish, and other predators. Serving as intermediate hosts for many kinds of parasites, especially trematodes (see Section 14.3: Class Trematoda), snails are often harmed by larval stages of parasites. There are three gastropod subclasses: Prosobranchia, Opisthobranchia, and Pulmonata. They are described in Section 16.3: Major Groups of Gastropods, but subclass names are commonly used to discuss particular animals. Familiar prosobranchs include periwinkles, limpets, whelks, conchs, abalones, and cowries. Marine sea slugs, sea hares, and nudibranchs are often called opisthobranchs. Pulmonates include most land and freshwater snails and slugs. Form and Function Torsion Gastropod development varies with the particular group under discussion, but in general there is a trochophore larval stage followed by a veliger larval stage where the shell first forms. The veliger has two ciliated velar lobes, used in swimming, and the developing foot is visible (see Figure 16.14). The mouth is anterior and the anus is posterior initially, but the relative positions of the shell, the digestive tract and anus, the nerves that lie along both sides of the digestive tract, and the mantle cavity containing the gills all change in a process called torsion. Figure 16.14 Ontogenetic torsion in a gastropod veliger larva. Torsion is usually described as a two-step process. In the first step, an asymmetrical foot retractor muscle running along the side of the gut and body organs contracts and pulls the shell and enclosed viscera (containing organs of the body) 90 degrees counterclockwise, relative to the head. This movement brings the anus from the posterior to the right side of the body (see Figure 16.14). Typical descriptions state that movement of the shell accompanies visceral movement, but detailed studies show that movement of the shell is independent of visceral movements. The first movements of the shell rotate it between 90 and 180 degrees into a position that will persist into adulthood. It was previously assumed that the mantle cavity, which houses both the gills and the anus in adult animals, moved with the anus in the first 90 degrees of torsion. However, studies show that the mantle cavity develops on the right side of the body near the anus, but is initially separate from it. The anus and mantle cavity usually move farther to the right and the mantle cavity is remodeled to encompass the anus. In a slower and more variable series of changes, the digestive tract moves both laterally and dorsally so that the anus lies above the head within the mantle cavity (see Figure 16.14). Page 348 After torsion, the anus and mantle cavity open above the mouth and head. The left gill, kidney, and heart atrium are now on the right side, whereas the original right gill, kidney, and heart atrium are now on the left, and the nerve cords form a figure eight. Because of the space available in the mantle cavity, the animal’s sensitive head end can now be withdrawn into the protection of the shell, with the tougher foot, and when present the operculum, forming a barrier to the outside. The developmental sequence just described is called ontogenetic torsion. Evolutionary torsion is the series of changes that produced the modern torted gastropod body from the ancestral untorted form. The hypothetical ancestral gastropod was assumed to have a posterior mantle cavity like the hypothetical ancestral mollusc (see Figure 16.3). It has long been assumed that morphological changes in ontogenetic torsion represent the sequence of evolutionary changes. However, new studies of development in several kinds of gastropods suggest a different scenario; researchers hypothesize that the ancestral gastropod had two lateral mantle cavities, much like those in Neopilina (see Figure 16.12) and in chitons (see Figure 16.11). A single mantle cavity over the head may have arisen when the left lateral mantle cavity was lost and the right cavity expanded toward the middle of the body after the first 90 degrees of torsion. Careful study of ontogenetic torsion shows that asynchronous displacements of the shell, the visceral mass and anus, and the mantle cavity are possible, although some features move together in some taxa. Torsion has been reinterpreted as a conserved anatomical stage, where the shell has moved to the adult position and the anus and mantle cavity are on the right side of the body, rather than a conserved process of change.4 Varying degrees of detorsion occur in opisthobranchs and pulmonates, and the anus opens to the right side or even to the posterior (see Figure 16.22). However, both of these groups were derived from torted ancestors. The curious arrangement that results from torsion, where the anus opens over the head and gills, creates the possibility of wastes being washed back over the gills (fouling) and causes us to wonder what strong evolutionary pressures selected for such a strange realignment of body structures. Several explanations have been proposed, none entirely satisfying. For example, sense organs of the mantle cavity (osphradia) would better sample water when turned in the direction of travel. Certainly the consequences of torsion and the resulting need to avoid fouling have been very important in the subsequent evolution of gastropods. These consequences cannot be explored, however, until we describe another unusual feature of gastropods—coiling. Coiling Coiling, or spiral winding, of the shell and visceral mass is not the same as torsion. Coiling may occur in the larval stage at the same time as torsion, but the fossil record shows that coiling was a separate evolutionary event and originated in gastropods earlier than did torsion. Nevertheless, all living gastropods have descended from coiled, torted ancestors, although some have lost these characteristics. Early gastropods had a bilaterally symmetrical planospiral shell, in which all whorls lay in a single plane (see Figure 16.15A). Such a shell was not very compact, since each whorl had to lie completely outside the preceding one. The compactness problem of a planospiral shell was solved by the conispiral shape, in which each succeeding whorl is at the side of the preceding one (see Figure 16.15B). However, this shape was clearly unbalanced. Better weight distribution was achieved by shifting the shell upward and posteriorly, with the shell axis oblique to the longitudinal axis of the foot (see Figure 16.15C). The weight and bulk of the main body whorl, the largest whorl of the shell, pressed on the right side of the mantle cavity, however, and apparently interfered with the organs on that side. Accordingly, the gill, atrium, and kidney of the right side have been lost in most living gastropods, leading to a condition of bilateral asymmetry. Surprisingly, a few modern species have secondarily returned to a planospiral shell form. Figure 16.15 Evolution of shell in gastropods. A, Earliest coiled shells were planospiral, each whorl lying completely outside the preceding whorl. B, Better compactness was achieved by snails in which each whorl lay partially to the side of the preceding whorl. C and D, Better weight distribution resulted when shell was moved upward and posteriorly. However, some modern forms have reevolved the planospiral form. Although loss of the right gill was probably an adaptation to the mechanics of carrying a coiled shell, that condition, displayed in most modern prosobranchs, made possible a way to avoid the fouling problem caused by torsion. Water is brought into the left side of the mantle cavity and out the right side, carrying with it wastes from the anus and nephridiopore, which lie near the right side. Ways in which fouling is avoided in other gastropods are mentioned as each subclass is discussed. Page 349 Feeding Habits Feeding habits of gastropods are as varied as their shapes and habitats, but all include use of some adaptation of the radula. Most gastropods are herbivorous, rasping particles of algae from hard surfaces. Some herbivores are grazers, some are browsers, and some are planktonic feeders. Haliotis, the abalone (see Figure 16.16A), holds seaweed with its foot and breaks off pieces with its radula. Land snails forage at night for green vegetation. Figure 16.16 A, Red abalone, Haliotis rufescens. These large limpetlike snails are prized as food and extensively marketed. Abalones are strict vegetarians, feeding especially on sea lettuce and kelp. B, Moon snail, Naticarius orientalis, from Sulawesi, Indonesia, is similar to the North American moon snail, a predator of clams and mussels. It uses its radula to drill neat holes through its victim’s shell, through which the proboscis is then extended to eat the bivalve’s fleshy body. Some snails, such as Bullia and Buccinum, are scavengers living on dead and decaying flesh; others are carnivores that tear their prey with radular teeth. Melongena feeds on clams, especially Tagelus, the razor clam, thrusting its proboscis between the gaping shell valves. Fasciolaria and Polinices (see Figure 16.16B) feed on a variety of molluscs, preferably bivalves. Urosalpinx cinerea, oyster borers, drill holes through the shells of oysters. The radula, bearing three longitudinal rows of teeth, begins the drilling action; then the snail glides forward, everts an accessory boring organ through a pore in the anterior sole of the foot, and holds it against the oyster’