Lesson No. 2 Chemical Thermodynamics PDF
Document Details
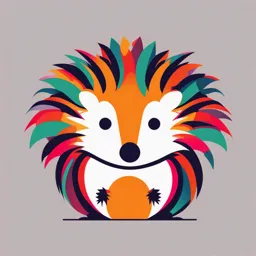
Uploaded by EnoughOceanWave5313
KBSU
Tags
Summary
This document provides a lesson about chemical thermodynamics. It covers topics such as thermodynamic systems, parameters, and Hess's law. The lesson is designed for an undergraduate-level audience.
Full Transcript
## Lesson No. 2 Basics chemical thermodynamics ### Subject and object of study thermodynamics | Subject of study | Object of study | |---|---| | thermodynamics -energy, forms of its existence and methods of transmission from one object to another. | thermodynamics- thermodynamic system. | | **Chem...
## Lesson No. 2 Basics chemical thermodynamics ### Subject and object of study thermodynamics | Subject of study | Object of study | |---|---| | thermodynamics -energy, forms of its existence and methods of transmission from one object to another. | thermodynamics- thermodynamic system. | | **Chemical thermodynamics** <br> - is a section of physical chemistry that studies conversion of various types of energy into chemical processes | | ### Thermodynamic system - a portion of space separated from the surrounding environment by a real or imaginary shell, containing a statistically significant amount objects. ### Thermodynamic systems | | closed | isolated | open | |----------------------------------------------------|---------------------------------------------------------------------------------------------------------|---------------------------------------------------------------------------------------------------------|----------------------------------------------------------------------------------------------------------| | **Exchange with environment energy and mass** | exchange with environment energy, but do not exchange mass | do not exchange with environment energy and mass | exchange with environment energy and mass | | **Example** | planet | thermostat | organism | ### Thermodynamic system - Homogeneous part of the system with the same chemical and thermodynamic properties, separated from other parts by a visible surface section, called **phase**. - Systems consisting of only one phase, are called **homogenetic** (homogeneous). - Systems containing two or more phases are called **heterogenetic** (heterogeneous). ### Thermodynamic parameters | main | general | |------------------------------------------------------------------------------|----------------------------------------------------------------------------| | **measurable** | **state functions** | | * pressure (P) <br> * temperature (T) <br> * volume (V) <br> * weight (m) | * internal energy (E) <br> * enthalpy (H) <br> * entropy (S) <br> * Gibbs energy (G) | Are measured **absolute values** Changes are measured **ΔΗ, ΔΕ, ΔΕ, AS** ### The heat supplied to the system is used to increase the internal energy of the system and doing external work $Q = ΔE + A$ where:<br> * ΔE - change in internal energy, <br> * A - work against external forces: A=p∆V In **isolated system** energy is the sum of all types energy: is a constant quantity $ΔE = 0$ ### Thermal effect at different constant parameters - In isobaric process <br> $p$ - const <br> $Q_p = ΔE + A= ΔE + pΔV$ <br> $ΔH = ΔE + pΔV$ <br> $Q_p= ΔH$ - In isohoric process <br> $V$ – const, $ΔV=0$ <br> $Q_v =ΔE$ ### The fundamental law of thermochemistry - Hess's law. The thermal effect of a reaction does not depend on the reaction path, but is determined only by the initial and final state systems ### An example of the implementation of Hess's law - $CO$ <br> $ΔH°_2$ ⬆ $ΔH°_3$⬇ | $ΔH°_1$ ⬅ $CO_2$ | $C$ - $ΔH°_1= ΔH°_2+ ΔH°_3$ <br> - $C + O_2→CO_2$ $ΔH°_1= – 393.5 kJ$ <br> - $C + 1/2O_2→CO$ $ΔH°_2= – 110.5 kJ$ <br> - $CO+1/2O_2→CO_2$ $ΔH°_3= −283.0 kJ$ (reference data) <br> - $- 393.5 = -110.5-283.0$ ### Hess's law is widely used in physiology, in particular to determine the caloric content of foods. <br> The body is not a source of energy, all types of work in it are performed at the expense of energy, standing outduring oxidation of substancesobtained from food, oxygen. <br> The caloric content of foods corresponds to the energy released during their use.combustion in oxygen The bulk of the available energy from food is retained in the body as chemical energy and is not released as heat. ### Classification of reactions by thermal effect - **exothermal reactions** occur with the release of heat: +Q or ∆H° reactions< 0 $C_2H_5OH + 3O_2→ 2CO_2+ 3H_2O + Q$<br> $C_2H_5OH + 3O_2→ 2CO_2+ 3H_2ΟΗ, ΔΗ0reactions<0$ - **endothermal reactions** occur with the absorption of heat: -Q or ∆H0reactions> 0 $N_2+O_252NO - Q$ <br> $N_2+O_252ΝΟ, ΔΗ reactions>0$ ### Consequences of Hess's Law 1. The heat effect of the direct reaction is equal to magnitude and opposite sign thermal effect of the reverse reaction (Lavoisier-Laplace law). 2. The thermal effect of a chemical reaction is equal to the difference between the sums of the standard heats of formation of the reaction products and the starting materials (ΔΗ°arr.), multiplied by the stoichiometric coefficients (и). In this case, the standard heats of formation of simple substances are equal to zero. $ΔH° = \sum (ν_i \cdot ΔH°_{form})_{products} - \sum (ν_i \cdot ΔH°_{form})_{reagents}$ For a hypothetical reaction aA + bB→ xX + yY $ΔH°_{reaction} =(xΔH°_{form,X} +y∆H°_{form,Y})-(a∆Η°_{form,A} +b∆H_{form,B})$ 3. The thermal effect of a chemical reaction is equal to the difference between the sums of the standard heats of combustion of the starting materials and the reaction products (ΔΗburnt), multiplied by the stoichiometric coefficients (u). At the same time, the standard heat of combustion of substances that do not support combustion is zero (water, carbon dioxide and most other oxides). $ΔH° = \sum(ν_i \cdot ΔH°_{burn})_{reagents} - \sum (ν_i \cdot ΔH°_{burn})_{products}$ For the hypothetical reaction aA + bB→ xX + yY $ΔH°_{reaction} = (aΔH°_{burn,A} +bΔH°_{burn,B})) - (xΔH°_{burn,X} +yΔH°_{burn,Y})$ ### Standard values: ΔΗ°, ∆S°, AG° Standard conditions: t = 25°C = 298 K; P = 1 atmosphere = 101.325 kPa ### Standard enthalpy formation - the thermal effect of the reaction of formation of 1 mol of a complex substance from simple substances under standard conditions. ### Standard enthalpycombustion - the thermal effect of the combustion reaction in oxygen of 1 mole of a substance with the formation of a higher oxide. ### Question. Select substances from the list: <br> a) the standard enthalpies of formation of which are equal to zero <br> b) the standard enthalpies of combustion of which are equal to zero. <br> In your answer, give the numbers in order. 1) H2O (f) 2) H2O (gas) 3) O2(gas) 4) Br2(gas) 5) CO (gas) 6) CO2(gas) 7) Fe(t) 8) Na (melt) ### Task number 1.Calculate the heat of reaction, complete oxidation of ethanol with oxygen, if standard conditions are known heat of formation of substances: ethanol (I) -278.1 kJ/mol, carbon dioxide (g) -393.5 kJ/mol, water (l) -285.9 kJ/mol. Describe the thermal effect of this reaction. ### Task number 2.Standard enthalpy of formation gaseous carbon dioxide -393.5 kJ/mol, standard enthalpy of reaction of interaction of graphite with nitrogen(I) oxide is -557.5 kJ. Calculate the heat of formation of N2O (g.). ### Task number 3.Calculate the heat of reaction esterification of ethanoic acid with ethanol according to known standard heat of combustion: ethanol (g) -1366.6 kJ/mol, ethanoic acid (I) -871.5 kJ/mol, ethyl ethanoate (I) -1802.9 kJ/mol. ### The second law of thermodynamics. Entropy. Entropy (S)-is a measure of the probability of the existence of a system in a given state (a measure of the disorder of the system). Unlike other thermodynamic parameters (H, G), it is possible to calculate the absolute value of the entropy of a substance. ### Boltzmann equation S= k·lnW where k is the Boltzmann constant: $k = \frac{R}{N_A}$, where R is the universal gas constant, 8.31 J/ mol K N₁- Avogadro's number, 6,02·10-23 mole-1 k=1,38·10-23;J/K; W'- thermodynamic probability of microstates that determines a given macrostate ### The second law of thermodynamics IN isolated system only flow such processes in which entropy either remains unchanged or increases, reaching a maximum when established thermodynamic equilibrium. △ S ≥ 0 ### Open systems In open systems, processes accompanied by a decrease in entropy are possible (∆ S < 0): * crystallization * vapor condensation * chemical transformation of gases into liquids and crystals. ### Qualitative determination of AS - When a substance passes from one aggregate state to another, the entropy of the system changes: AS>0 AS>>0 solid ↔ liquid ↔ gaseous AS<0 AS<<0 - Example: | Aggregate state | Standard entropy J/mol K | |---|---| | Ice | 39.7 | | Liquid water | 70.08 | | Water vapor | 188.72 | ### Qualitative determination of AS (continuation) - When formed during the reaction from solids, liquids or gases, and from liquids to gases, entropy increases. In the opposite case, it decreases. heating NH3 (gas) + HCl (gas) AS>0 NHCI (cryst.) → FeO (solid) AS<0 (gas) Fe(solid) + O2 ### Qualitative determination of AS (continuation) - When increasing during the reaction the number of moles of gaseous substances, the entropy increases. In the opposite case, it decreases: N2 (gas) + 3H2 (gas) AS<0 AS>0 2NH3 (gas) ------- ------- ------- 4 moles of gas 2 moles of gas ### Calculating the change in entropy The change in entropy in the process obeys the corollary of Hess's law: $ΔS = \sum (ν_i \cdot S_{products}) - \sum(ν_i \cdot S_{reagents})$ ### Task.Calculate the standard change entropy in the process 2SO2(g) + O2(G) ⇌ 2SO3(G) Reference data: | Substance | Standard entropy J/mol K | |---|---| | SO2(G) | 248.1 | | O2(G) | 205.04 | | SO3(G) | 256.23 | ### Gibbs equation In nature, two opposing tendencies have been identified: 1) The tendency of particles to form bonds, which is accompanied by the release of energy 2) The tendency of particles to destroy bonds, which is accompanied by an increase in entropy. These tendencies are opposite to each other, thereforea universal criterion is needed to assess the possibility of processes occurring without work being performed by external forces. ##### Universal criterion spontaneity of the process - Gibbs energy Gibbs energyG- maximum useful work done by the systemat **constant** pressure and temperature. Change in Gibbs energy - AG is called **isobaric-isothermal** potential - ### Gibbs equation $ΔG = ΔH - TΔS$ It has been established that in open systems only those processes proceed spontaneously processes that are characterized by a negative **isobaric - isothermal** potential value. ### Calculation of the change in Gibbs energy (isobaric-isothermal potential) Gibbs energy- is a state function, so it is calculated according to the consequences of Hess's law: $ΔG°_{reaction} = (\sum v_iΔG°_{formation~of~products}) - (\sum v_iΔG°_{formation~of~reagents})$ Standardchange in the Gibbs energy of formation the change in the Gibbs energy of formation of 1 mole of a substance of the corresponding simple substances under standard conditions is called the change in the Gibbs energy of formation of 1 mole of a substance of the corresponding simple substances under standard conditions. The standard Gibbs energies of formation of simple substances in stable aggregate states under standard conditions are taken to be equal to zero: $ΔG°_{form,H_2(gas)} = 0, ΔG°_{form,O_2(gas)} = 0.$ ### Calculation of the change in Gibbs energy (isobaric-isothermal potential) The Gibbs energy is a function of state, so it is calculated according to the consequences of Hess's law: $ΔG°_{reaction} = (\sum v_iΔG°_{formation~of~products}) - (\sum v_iΔG°_{formation~of~reagents})$ Standard change in Gibbs energy **combustion** VThe change in Gibbs energy during the combustion of 1 mole of a substance with the formation of a higher oxide that is stable under standard conditions is called the energy of a substance. The standard Gibbs energies of combustion of higher oxides stable under standard conditions are taken to be zero: $ΔG°_{comb,H_2O_{(l)}} = 0; ΔG°_{comb,CO_2(g)} = 0.$ ### Reversible processes Reversible processes are characterized by an isobaric-isothermal potential in a ***narrow range:*** * **-40 kJ ≤ AG ≤ +40 kJ** ### Criteria of spontaneity process: * △H< 0 - enthalpy factor * AS> 0 – entropy factor * ∆G< 0 – universal criterion ### Gibbs equation $AG = ΔH - TΔS$ | ΔH | AS | Possibility of a spontaneous process | |---|---|---| | - | + | The process occurs spontaneously at any temperature. | | - | - | The process can occur spontaneously at low temperatures. | | + | + | The process can occur spontaneously at high temperatures. | | + | - | The process does not occur spontaneously at any temperature. | ### •AG= 0 – thermodynamic equilibrium Thermodynamic equilibrium state- constancy of the properties of a system over time, while there are no flows of matter and energy. ### • Steady state- constancy of properties systems in time, which is supported by the exchange of matter, energy, information with the environment (homeostasis). ### Coupled reactions glucose + fructose → sucrose + H₂O Endergonic process AG > 0 driven reaction + 20.9 kJ/mol ATP + H2O → ADP +H3RO4 Ex.ergonic process ∆G< 0 leading reaction - 30.4 kJ/mol | ∆Gdriven reaction | < | Gleading reaction | Summary equation: ATP + glucose + fructose → sucrose + ADP + H3RO4 AG = +20.9-30.4=-9.5 kJ/mol AG< 0 coupled reactions occur spontaneously ### Application of knowledge of patterns chemical thermodynamics * determination of the fundamental possibility of the flow chemical interactions between these substances under certain conditions; * calculation of the amount of energy that will be released during carrying out a reaction or the costs required to carry it out; * prediction of the extent to which it will proceed a chemical reaction before chemical equilibrium is established under given conditions; * selection of optimal conditions for the process, ensuring the maximum yield of the desired product.