Wk 9 - MC Questions - Cardiology Dysrhythmias - PDF
Document Details
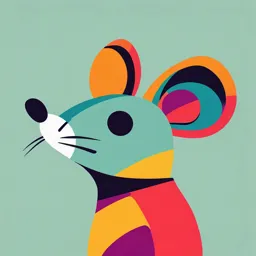
Uploaded by DivineRed
Canadian College of Naturopathic Medicine
Tags
Summary
This document contains learning outcomes, pre-assessment questions, and a review of electrocardiograms (ECGs). It covers topics such as heart rate, intervals (P-R, QRS, Q-T), normal waveforms, and common dysrhythmias. Information on ECG leads and interpretation is also included.
Full Transcript
Cardiology: Dysrhythmias and Basic ECG’s BMS200 Week 9 Learning Outcomes Analyze the following characteristics of a normal ECG: ∙ Heart rate and determination of rhythm ∙ P-R, QRS, Q-T intervals ∙ Normal waveforms in the P, QRS, and T waves Describe how triggered activity, abnorma...
Cardiology: Dysrhythmias and Basic ECG’s BMS200 Week 9 Learning Outcomes Analyze the following characteristics of a normal ECG: ∙ Heart rate and determination of rhythm ∙ P-R, QRS, Q-T intervals ∙ Normal waveforms in the P, QRS, and T waves Describe how triggered activity, abnormal automaticity, and re-entry contribute to the pathophysiology of the most common dysrhythmias Describe the basic epidemiology, pathogenesis, clinical features, ECG findings, and prognosis of the following supraventricular dysrhythmias: ∙ Atrial fibrillation, atrial flutter, sinus tachycardia, and paroxysmal supraventricular tachycardia Learning Outcomes Describe the basic epidemiology, pathogenesis, clinical features, ECG findings, and prognosis of the following ventricular dysrhythmias: ∙ Premature ventricular contraction, idioventricular rhythm, ventricular tachycardia, ventricular fibrillation, torsades de pointes Describe the basic epidemiology, pathogenesis, clinical features, ECG findings, and prognosis of the following types of conduction block: ∙ 1st degree heart block, 2nd degree heart block, 3rd degree heart block Describe the ECG findings that are typical of cardiac ischemia and relate these findings to basic vascular territories Briefly describe the pharmacologic mechanism of action and related adverse effects of common anti-arrhythmic medications Discuss the pathophysiologic contribution of chronic inflammation and myocardial fibrosis to the development of common dysrhythmias What do you think is happening? Did you “catch” a serious You’ve dysrhythmia just purchased in your a brand-new electronic colleague? stethoscope that records heart sounds and can transmit a simple two-lead ECG to your smartphone. The audio is great when you test it on yourself, but the appearance of the ECG seems to change drastically depending on where you place it on your chest. Your classmate asks you to try it out on them – you record the following trace when the device is placed on their chest: Pre-Assessment: What electrical event is occurring during the Q-T interval of an ECG? A. The plateau phase of the atrial myocyte action potential B. The initial depolarization of the atrial myocyte C. The plateau phase of the ventricular myocyte action potential D. Repolarization of the ventricular myocyte Pre-Assessment: Where in the heart would you find the cells that usually perform the role of the cardiac pacemaker? A. The right atrium, close to the entrance of the superior vena cava B. The left atrium, close to the entrance of the right pulmonary veins C. Close to where the tricuspid valve attaches next to the interventricular septum D. Close to where the mitral valve attaches next to the interventricular septum Review: Electrocardiogram (ECG) Recording of Electrical Impulses: An electrocardiogram (ECG or EKG) captures electrical activities produced by the heart by placing electrodes on the skin, which detect the voltage changes resulting from the depolarization and repolarization of the cardiac muscle. Size of the Waves: The amplitude of the waves on an ECG represents the amount of electrical activity occurring in the heart muscle. Larger waves indicate greater amounts of electrical activity, which typically correspond to larger areas of myocardial tissue being activated. This can give insights into the health and functionality of the heart Review: Electrocardiogram (ECG) Direction of Voltage Changes: The upward or downward deflections from the baseline reflect the direction of electrical conduction through the heart. An upward deflection indicates that the electrical impulse is moving toward the electrode a downward deflection suggests that it is moving away. This is crucial for understanding how different parts of the heart are activating Conducting/Automatic Tissues: The conducting tissues (like the SA node, AV node, and bundle branches) are smaller and generate less electrical activity than the myocardial tissues, which makes them difficult to register on an ECG. The ECG primarily reflects the activity of the larger myocardial cells that contract and pump blood. Review: Electrocardiogram (ECG) Duration of the Waves: The duration of the waves (P wave, QRS complex, T wave) provides important information about the timing of electrical events in the heart. For example, a prolonged QRS complex may indicate a delay in ventricular conduction a prolonged QT interval could suggest a risk of arrhythmias. Monitoring the duration of these waves is essential for assessing cardiac health and function. Review: What does ECG measure? ECG leads measure the extracellular current that travels from depolarized cells to polarized (resting) cells Differences in charge at the extracellular border of the plasma membrane ECG leads only “notice” changes in membrane potential “plateaus” do not register as waves (i.e. phase 4, phase 2 of a myocyte) Technically, they compare the difference in voltage between different regions of the heart Review: ECG Timing How do the ECG waves and intervals correspond to the excitation along the conduction pathway? Recall Cardiac Physiology lecture and the role of the AV delay: Allowing time for atrial contraction and optimizing ventricular filling ECG: Amplitude and Vectors Amplitude: The amplitude refers to the height of the waves seen on the ECG. It indicates the magnitude of the electrical potential difference across different parts of the heart. Length of vector: The length of the vector corresponds to the magnitude of the electrical potential difference (size of difference) between two points in the heart during depolarization or repolarization. The direction of the vector indicates the orientation of the electrical activity (which way the electrical impulse is traveling). Placement of ECG Leads - 1 ECG leads are placed to give a “3-D” view of the electrical activity of the heart Coronal view (left and right arms, left leg) Cross-sectional view (precordial leads) The vector of depolarization is displayed relative to the placement of the ECG lead Placement of ECG Leads - 2 Bipolar leads compare voltage changes between leads. i.e., lead I compares voltage changes between the right arm and the left arm “Unipolar” (precordial leads) leads compare voltage changes between a lead (surface of chest wall) and the center of the heart ECG: X and Y axis Electrical potential changes across the heart over time Time – x-axis, in seconds Electrical potential changes – voltage in mV, y-axis “little box” – 0.1 mV high, and 0.04 seconds wide Each “big box” is 0.5 mV by 0.2 seconds Approach to Interpreting ECG Best to approach them in an organized fashion: 1. Determine rate (Step 1) Two methods: Divide 300 by number of large boxes between R-waves (R-R interval) = rate 5 large boxes = 1 second, 50 large boxes = 10 seconds, so # of R waves in 50 large boxes X 6 (60 seconds in 1 minute) (somewhat cumbersome) 2. Determine rhythm (Step 2) Where are the impulses coming from (P-wave before the QRS, or something else going on)? Is it regular? If irregular, is it regularly irregular, or irregularly irregular? Is it a sinus rhythm? (what is a sinus rhythm?) Normal Sinus Rhythm? Step 2 – Rhythm Regular rhythm or regularly irregular Best determined by looking at a rhythm strip rhythm Each P-wave is Usually lead II, often a 10-second recording (50 big boxes) followed by a QRS FYI - P-wave Regular or irregular? regular – can be a normal finding, artificial has a normal pacemakers can give a regular rhythm as well axis and is the irregular – irregularly irregular or regularly same shape irregular? each beat regularly irregular can be normal, as PR interval is heart rate varies with respiration – there is a pattern to constant the irregular rhythm irregularly irregular is the hallmark of a QRS interval is < number of tachycardias, most 100 ms (2.5 small commonly atrial fibrillation and is boxes) abnormal Each QRS has a P- wave before it A Normal 12-Lead ECG Rhyth m Step 3 – Intervals (1) 3. Look at the intervals Are they widened or shortened? Often a change in an interval is a result of a conduction system problem Major intervals of interest: P-R interval – usually prolongation = AV-nodal dysfunction It can be decreased, though in situations where there are abnormal “connections” between the atria and ventricles QRS interval – delay in ventricular excitation QT interval – repolarization abnormalities (as a risk factor for torsades de pointes) We consider S-T intervals in the “waves” portion Step 3 – Intervals (2) The intervals can often tell you much about where the conduction problem lies narrow QRS = usually normal intraventricular conduction pathways normal QT = normal ventricular repolarization normal P-R = no abnormal delays/conduction QT at the AV node varies with heart rate… so Interval Seconds Small boxes how do you P-R (P-Q) 0.12 – 0.2 3-5 figure out a normal QT?? QRS 0.08 – 0.10 2 – 2.5 QT QT QTc = QT/√R-R Less than half of R-R corrected = usually good QT___ √R-R QT interval Step 4 – Waves 4. Look at the waves Are they positioned normally (up or down)? Are they “funny-shaped” or do they change morphology from beat to beat? Are the waves larger/smaller than normal? Are there waves present that normally aren’t present in a healthy person? NOTE: when examining the waves, it is important to look at them in all leads – they will change from lead to lead Step 4 – Q-Waves Abnormal Q-waves – if these abnormalities are seen, indicates current or prior MI: “significant” Q-waves are: wide (> 1 small box or 0.04 sec) large (> 2 mm deep or > 25% of the total size of the QRS) or > 1/3 height of the R wave in leads V1 – V3 are abnormal, always Since MI is so common, it is key to recognize these Step 4 – ST segments Helps to think about ST segment with waves instead of intervals usually with intervals you’re thinking about times that are shorter or longer than normal with the ST segment you’re concerned about elevation or depression Your biggest concern is ruling out an infarct, but many things can cause ST depression or elevation ST Depression and ST Elevation S-T depression S-T elevation NSTEMI or opposite STEMI or opposite lead lead from site of STEMI from site of NSTEMI digoxin pericarditis hypokalemia LBBB RBBB or LBBB LVH RVH or LVH normal if small implanted pacemaker implanted pacemaker Raised ICP (intracranial hyperkalemia pressure) PE Raised ICP Step 4 – T-Waves The differential for T- wave changes is large, and depends T-waves – many abnormalities can occur, on a few things: but they should be upright in all of the age – T-wave leads except for V1 Some basic abnormalities inversions are tall T-waves normal in kids hyperkalemia ischemia and early myocardial infarction “metabolic” strain small T-waves – i.e. the heart is hypokalemia working hard with Inverted T-waves not enough to myocardial infarction ventricular hypertrophy “feed” it electrolyte abnormalities Step 4 – P-Waves P-wave abnormalities P-wave shapes that change from beat-to-beat (indicates the FYI - Atria enlarge for “pacemaker” is not the same each beat) the same reason P-wave absence – think atrial other heart chambers fibrillation More P-waves than QRS complexes – enlarge heart block Increased atrial FYI: P-waves that indicate atrial hypertrophy “afterload” see diagram for right and left atrial i.e., a stenotic hypertrophy patterns many ECGs will also determine an AV valve atrial axis – between 0 and +75 Atria are degrees “stretched” by more fluid than General Pathophysiology of Dysrhythmias (1) Re-entry: Normal depolarization wave enters a pathological space in the heart (i.e., area of ischemic injury), contraction cannot occur because the tissue is not functioning properly BUT it may allow a slower conduction of this wave towards healthy tissue IF healthy cardiac tissue have completed their original refractory period, then this wave of depolarization can trigger an action potential called “re-entry” and resulting in tachycardia (1) conditions that slow conduction but not block (2) conditions that shorten refractory period of healthy cells General Pathophysiology of Dysrhythmias (2) Ectopic Foci or Abnormal Automaticity: Scar tissue (i.e., post-MI or other injury) changes local plasma electrolyte concentrations or their movements across channels resulting occurrence of automaticity in previously non- pacemaker cells – ectopic foci Inhibition of Na+/K+ pump results in intracellular accumulation of Na+ and Ca2+ which partially depolarize the membrane Can occur in healthy individuals occasionally Situations that promote abnormal automaticity: (1) Increase of intracellular Ca2+ (2) Decrease of K+ conductance at rest Catecholamines (fatigue, stress), hyperkalemia, hypercalcemia, heart distention General Pathophysiology of Dysrhythmias (2) Ectopic Foci or Abnormal Automaticity: Cardiac Metabolism: IR K+ channels get inactivated by intracellular ATP (when metabolism is ok, no extra K+ is effluxing from the myocyte) and get activated by intracellular ADP (when the cardiac myocyte is metabolically stressed i.e., ischemia) allowing K+ to efflux and thus reducing the refractory period General Pathophysiology of Dysrhythmias (3) Triggered Activity: Ventricular arrhythmia, whereby a normal action potential is followed by an abnormal depolarization of ventricular muscle that occurs before the original action potential has completed its course Example: premature ventricular contractions (PVC) Conditions that favor this: Situations that prolong action potential duration Bradycardia and reduced or prolonged phase 3 (K+ efflux/ repolarization) Tachycardia and increased calcium accumulation within myocyte Chronic Inflammation and Myocardial Fibrosis and Dysrhythmias Chronic inflammation Immune cells (macrophages, mast cells, T-cells) can promote myocardial repair (as in acute inflammation) OR fibrosis by releasing cytokines that direct function of cardiac fibroblasts Cardiac fibroblasts are triggered to transform into myofibroblasts This transformation is supported by fibrosis-promoting factors: angiotensin II, aldosterone, catecholamines, connective tissue growth factor, endothelin, platelet- derived growth factor, reactive oxygen species, TGF-Beta and inflammatory cytokines Myofibroblasts produce more ECM and secrete substances that promote fibrosis Myocardial fibrosis leads to remodeling of myocardial collagen May cause local delay in portion of the heart Promotes reentry Supraventricular Dysrhythmias Atrial fibrillation Most common type of arrhythmia Risk Factors: age, hypertension, chronic lung or heart disease, congenital heart disease, increased alcohol, sleep apnea Pathophysiology: atrial structural (ECM, fibrous tissue deposition) and electrical (tachycardia, shortening of refractory period) remodeling leading to ectopic foci Once fibrillation occurs 🡪 (1) turbulent blood flow (2) reduced effectiveness of heart’s function (3) risk increased thrombus formation Sx: asymptomatic OR chest pain, palpitations, dyspnea, tachycardia, nausea, dizziness, diaphoresis, fatigue ECG: narrow complex “irregular irregular” pattern with no distinguishable p-wave Prognosis: leading cardiac cause of stroke Atrial Fibrillation ECG 1 Narrow complex “irregular irregular” pattern with no distinguishable p- wave Atrial Fibrillation ECG 2 Narrow complex “irregular irregular” pattern with no distinguishable p- wave Supraventricular Dysrhythmias Atrial flutter VERY common Pathophysiology: re-entry mechanism due to fibrosis (1) Different refractory period (2) Fast and slow conductions – together these allow for re-entry ECG: Fast atrial rate (300 bpm) with fixed or variable ventricular rate Flutter waves without an isoelectric line in between QRS complex SX: fatigue, palpitations, syncope Supraventricular Dysrhythmias Sinus tachycardia Normal rhythm, heart beats faster and results in increased cardiac output Often due to exercise or stress; concerning when occurs at rest Physiological (normal): catecholamines due to exercise, stress, pain, or anxiety Pathological (concerning) Cardiac causes include myocarditis, coronary artery syndrome and others Non-cardiac: pulmonary embolism, hypoxia, hypoglycemia, dehydration, infection, electrolyte imbalance, shock and others Supraventricular Dysrhythmias Paroxysmal supraventricular tachycardia (SVT) Intermittent (paroxysmal) episodes of supraventricular tachycardia with sudden onset and termination Originate either in the atria or AV node and can create either regular or irregular rhythms MANY Etiologies: hyperthyroidism, caffeine, cocaine, structural heart disease, rheumatic dz, PE, pneumonia, anxiety, MVP, myocarditis, pericarditis… Pathophysiology: most often re-entry, sometimes due to increased automaticity or trigger Re-entry circuits: SA node, atria, AV node or accessory pathway SX: dizziness, syncope, nausea, dyspnea, palpitations, neck pain, chest discomfort, anxiety, diaphoresis Prognosis: good if no structural problems, otherwise can lead to heart failure, MI or pulmonary edema Paroxysmal supraventricular tachycardia (SVT) – ECG ECG: Regular rhythm Fast heart rate (150-250 bpm) Often narrow QRS complex Ventricular Dysrhythmias Premature ventricular contraction (PVC’s) Heartbeat is initiated by Purkinje fibers Can occur in isolation or as a double (Normal beat (QRS) – PVC – PVC – Normal beat (QRS).) or triplet (Normal beat (QRS) – PVC – PVC – PVC - Normal beat (QRS).) More than three PVC’s are termed ventricular tachycardia Common (FYI 1-4% of population) Etiology: often unknown, caffeine, excess catecholamines, anxiety, electrolyte imbalance, alcohol, sleep deprivation, structural heart disease, anemia, hyperthyroidism Pathophysiology: Ectopic nodal automaticity Re-entry Triggered activity Ventricular Dysrhythmias Premature ventricular contraction (PVC’s) SX: “Skipped heartbeat” followed by fluttering sensation (palpitation), though majority are asymptomatic Lightheadedness, chest pain, chest discomfort, dyspnea, anxiety (syncope very rare) Prognosis: if no heart disease – no problem, if heart disease than increased mortality risk ECG: abnormal and wide QRS complex occurring earlier than expected in cardiac cycle Ventricular Dysrhythmias Idioventricular rhythm Slow regular ventricular rhythm with a rate of less than 50 bpm (typically), absence of P waves, and a prolonged QRS interval – the SA node isn’t working, and the ventricle takes over! Etiology: heart block, electrolyte abnormalities, medications, during reperfusion after MI Pathophysiology: Suppression of SA and AV nodes that allow ventricles to take over and generate rhythm (50 bpm) SX: mostly asymptomatic OR palpitations, lightheadedness, fatigue or syncope ECG: wide QRS complex, rate < 50 (idioventricular) or 50-100 (accelerated idioventricular rhythm) Ventricular Dysrhythmias Ventricular Tachycardia (VT) Most commonly due to ischemic heart disease SX: palpitations, chest pain, dyspnea, syncope, cardiac arrest ECG: Greater than 3 consecutive ventricular beats with rate of 100-250 bpm and wide QRS complex Potentially life threatening (sudden cardiac death due to progression to ventricular fibrillation) Pathophysiology: Diverse group of tachycardia’s Re-entry (most common) – scar related Triggered activity and enhanced automaticity Ventricular Dysrhythmias Ventricular tachycardia (VT) Pathophysiology and Prognosis: Leads to low cardiac output due to significant reduction in preload and thus stroke volume If heart is hypoperfused it may lead to ventricular fibrillation or cardiomyopathy, heart failure or other complications Ventricular Dysrhythmias Ventricular Fibrillation Irregular (uncoordinated) electrical activity, ventricular rate >300 bpm, which compromises cardiac output significantly! Leads to sudden cardiac death (fatal within minutes if not treated – defibrillator and CPR) Etiology: myocardial infarction, electrolyte abnormalities, congenital QT abnormalities, alcohol use, cardiomyopathies, hypothermia and others Pathophysiology: Increased automaticity by Purkinje cells Triggered activity Possible re-entry Ventricular Dysrhythmias Ventricular Fibrillation SX: chest pain, dyspnea, nausea, vomiting prior to sudden collapse (unconscious, unresponsive, no pulse) ECG: (1) fibrillations of various amplitudes and shapes (2) no identifiable P wave, QRS complex or T wave (3) heart rate between 150-500 bpm Ventricular Dysrhythmias Torsades de pointes Form of ventricular tachycardia ECG: polymorphic; “twisting” varying in amplitude QRS complexes Associated with QTc prolongation (>500 ms) Congenital or acquired (LOTS of medications can cause the QTc to be prolonged) Rhythm may terminate spontaneously or progress to ventricular fibrillation Pathophysiology is not fully understood but believed to be due to prolonged repolarization phase due to delay in K+ efflux. If an ectopic beat occurs during this time, it can trigger Torsades de pointes SX: most asymptomatic OR syncope, palpitations, dizziness Cardiac death is the presenting sx in 10% of cases Conduction Block Types of arrhythmias characterized by a delay or complete block in the electrical conduction system of the heart, particularly affecting the atrioventricular (AV) node or the bundle branches. They can lead to inadequate heart rate and may cause symptoms such as dizziness, fatigue, or syncope. Types of Heart Blocks First-Degree AV Block: Description: Prolonged PR interval (>200 ms) on ECG but every impulse is conducted to the ventricles. Symptoms: Usually asymptomatic and often benign. Second-Degree AV Block: Type I (Wenckebach): Description: Progressive prolongation of the PR interval until a QRS complex is dropped. Symptoms: Often asymptomatic but can cause occasional dizziness. Type II: Description: Consistent PR intervals with sudden drops of QRS complexes (can be more serious). Symptoms: May lead to bradycardia and require monitoring or pacemaker. Types of Heart Blocks Third-Degree AV Block (Complete Heart Block): Description: No impulses from the atria reach the ventricles, resulting in independent atrial and ventricular rates. Symptoms: Can cause severe bradycardia, syncope, or heart failure; often requires pacemaker insertion. Conduction Block 1st degree AV Heart Block Abnormally slow conduction through the AV node resulting in a prolonged P-R interval (>.20 seconds) without any disruptions of atrial or ventricular conduction Typically, asymptomatic and does not require treatment as typically does not result in complications Possible caused by increased vagal tone (younger patients) or fibrotic changes in cardiac conduction (elderly patients) Conduction Block 2nd degree Heart Block Delay in conduction of the AV node resulting in prolonged PR interval and missing QRS Mobitz Type 1 (aka Wenckebach) No structural problem, high vagal tone but can be due to cardiac problems ECG: progressively prolonged PR interval until finally the action potential wave can’t get through AV node and there is not QRS complex following P wave, then process restarts Mobitz Type 2 Mostly seen in patients with structural heart disease Can progress to complete heart block (3 degree) rd ECG: constant PR interval with 1 P wave being dropped every certain number of beats (but only 1!), as in its not followed by a QRS Conduction Block 2nd degree Heart Block SX: can be asymptomatic OR fatigue, dyspnea, chest pain, presyncope or syncope or even sudden cardiac arrest (Mobitz Type 2) Conduction Block 3rd degree (Complete) Heart Block Complete loss of communications between atria and ventricles via AV node (fairly rare) Cardiac output is significantly reduced – fatal if not treated promptly (FYI most treated with pacemaker) Either the AV node or the Purkinje fibers step in to act as pacemakers for the ventricles Bradycardia 25% REM theta-like movements, almost will report dream content. More REM Adults - ~ 25% no MSK movement late in the sleep session, less early on. Elderly - < 25%, often (R) Need to be in N2 or N3 prior to very little entering REM How are sleep-wake states regulated? In general, there seems to be an arousal system (green circles) that keeps us awake The arousal system seems to “open the gate” of the thalamus and allow input from the outside world to the cortex The arousal system also communicates widely to hypothalamic areas that regulate sleep cycles as well as to the cortex in general How are sleep-wake states regulated? Major components of the arousal system include brainstem nuclei that extend mostly through the midbrain and pons (lots of green circles) Locus ceruleus - norepinephrine Raphe nucleus - serotonin Tuberomamillary body- histamine Acetylcholine has multiple nuclei in the brainstem that are important in arousal Periaqueductal gray - dopamine How are sleep-wake states regulated? A nucleus in the hypothalamus – the ventrolateral pre-optic nucleus (VLPO) seems to be one of the major sleep-promoting nuclei ▪ Releases inhibitory NTs like GABA and galanin REM sleep is likely regulated differently – in the brainstem there are “REM-on” (lateral pontine areas) and “REM-off” groups (pons portion of the locus ceruleus) How are sleep-wake states regulated? The suprachiasmatic nucleus (SCN): Communicates with the retina Is the most likely “site” of our circadian rhythm ▪ Without light/dark stimulation, we have a rhythm that lasts just a little over 24 hours Responsible for “entraining” our circadian rhythm based on light and dark information Communicates indirectly with arousal systems and sleep-inducing centers How are sleep-wake states regulated? Finally, there are the “stabilizing” nuclei The lateral hypothalamus has a population of neurons that secrete two major chemicals: ▪ Orexin – projects to both the arousal systems and to the VLPO ▪ Melanin-concentrating hormone (MCH) – also projects to arousal systems Orexin and MCH are important for keeping a subject in a “stable” sleep state A more detailed image - arousal systems and VLPO projections Neurobiology of the Sleep-Wake Cycle: Sleep Architecture, Circadian Regulation, and Regulatory Feedback, Patrick M. Fuller, Joshua J. Gooley, and Clifford B. Saper, Journal of Biological Rhythms 2006 21:6, 482-493 See notes for details Neurophysiology of Sleep General model: We don’t want any “halfway between awake and asleep” situations ▪ Therefore the sleep centers will directly and indirectly inhibit the arousal centers ▪ The arousal centers also directly inhibit the sleep centers There are two drivers that make us sleepy ▪ The circadian rhythm that tells us it’s bedtime ▪ The homeostatic signal that builds the longer we go without sleep Neurophysiology of Sleep What pushes us towards wakefulness? Stimuli – whether it’s circadian or homeostatic or some other stimulus in general – activate our arousal systems ▪ In this diagram they’ve included the tuberomammillary nucleus (histaminergic), the locus coeruleus (noradrenergic), and the raphe nuclei (serotonergic) The arousal system directly inhibits the VLPO nuclei Orexin activity is increased – and orexin also activates the arousal nuclei Orexin + inhibition of the VLPO 🡪 a durable waking state Neurophysiology of Sleep What pushes us towards sleep? Stimuli – whether it’s circadian or homeostatic activate the VLPO ▪ The VLPO directly inhibits both the arousal systems and orexin release from the lateral hypothalamus Since orexin release from the lateral hypothalamus is silenced (orexin stimulates the arousal system) AND increased activity of the VLPO inhibits the arousal The thalamocortical system directly, then a durable sleep state is maintained oscillations that generate EEG waves are also turned back on A more detailed image - arousal systems and VLPO projections Neurobiology of the Sleep-Wake Cycle: Sleep Architecture, Circadian Regulation, and Regulatory Feedback, Patrick M. Fuller, Joshua J. Gooley, and Clifford B. Saper, Journal of Biological Rhythms 2006 21:6, 482-493 See notes for details Neurophysiology of Sleep Since the sleep-inducing and the arousal groups of neurons inhibit each other, we’re usually either sleeping or awake, not in-between ▪ Like a light-switch – this is known as a “flip-flop switch” ▪ MCH release from the lateral hypothalamus predominates during REM sleep – it inhibits the monaminergic arousal system (keeps you asleep during REM) Neurophysiology of Sleep This is the case for REM sleep as well ▪ REM-on neurons inhibit the REM-off neurons, and REM-off neurons inhibit REM-on neurons ▪ REM-on neurons project widely and are stimulated by cholinergic inputs cause the previously-mentioned inhibition of movements as well as the brain activity in the association areas and limited parts of the forebrain ▪ REM-off neurons tend to be stimulated by norepinephrine and serotonin inputs AND they are also stimulated by orexin release from the lateral hypothalamus REM, Non-REM, and Wakefulness model See description below A brief segue – melatonin and circadian rhythms Melatonin is produced in the pineal gland, a tiny, pea-sized portion of the epithalamus right above the colliculi in the midbrain attached to the▪ Itroof of the stimulated is indirectly 3rd ventricle (no BBB) to produce melatonin during periods of darkness Melatonin is an interesting metabolite of serotonin (which a.a.?) that may have other functions other than entrainment of circadian rhythms https://upload.wikimedia.org/wikipedia/commons/6/6b/ Regulation of melatonin secretion In the absence of light 🡪 retinohypothalamic fibres relay “dark info” to the SCN 🡪 lifting of the inhibition of the PVN by the SCN In the absence of light, the PVN then activates the sympathetic nervous ▪ system: Intermediolateral horn cells excite post- ganglionic neurons in the superior cervical ganglion ▪ NE is released at the pineal gland 🡪 melatonin synthesis and release Regulation of melatonin secretion Serotonin is synthesized in the pineal gland With catecholamine stimulation (beta1-receptors) the activity and production of AANAT and the availability of serotonin increases 🡪 more melatonin synthesized With withdrawal of catecholamines, AANAT is degraded by proteosomes What does melatonin do, sleep-wise? Although the SCN has its own endogenous cycle that is just over 24 hours long, it needs to be entrained ▪ The retinohypothalamic fibres can relay light information and “synch up” the SCN to day/night periods ▪ However, melatonin also seems to have a role, and activation of melatonin receptors (most likely MT-2 type receptors are more important) helps the entrainment of the SCN to light-dark cycles Melatonin can both decrease sleep latency and increase the amount of time that a subject spends sleeping ▪ Thought that MT-1 receptors decrease sleep latency and MT-2 receptors help maintain sleep for longer (may be oversimplified) Melatonin secretion entrains a number of physiologic/endocrinologic cycles to day-night cycles: ▪ Cortisol secretion and TSH secretion ▪ Core body temperature ▪ Heart rate variability Melatonin plays a key role in the entrainment of these circadian rhythms to light-dark stimuli https://www.ncbi.nlm.nih.gov/books/NBK550972/#:~:text=The%20main Homeostatic and Allostatic Regulators of Sleep We now are aware of melatonin and the SCN as important circadian regulators of sleep-wake cycles However, the longer we go without sleep, the more we desire it and the more likely we are to lapse into N1 (nod off) when we close our eyes ▪ This is known as the homeostatic drive for sleep ▪ Although knowledge is still developing, it may be that extracellular adenosine build-up during wakefulness may be responsible for the homeostatic drive Caffeine is an adenosine receptor (A2a) antagonist A1 receptors are thought to inhibit arousal pathways, A2a are thought to facilitate sleep-promoting pathways Homeostatic and Allostatic Regulators of Sleep Allostasis = the response to stressors (physical, psychologic) that cannot be adequately managed by homeostatic mechanisms ▪ People who endure psychologic stress have difficulty sleeping (no surprise there) ▪ The ascending monaminergic arousal system is excessively activated in these situations This is of course adaptive if it keeps you from falling asleep in a stressful situation, but impairs sleep quality over long periods of time Common disorders of sleep Narcolepsy Restless legs syndrome & periodic limb movement disorder Obstructive sleep apnea Selected parasomnias Narcolepsy Thought to affect 1 in 2000 people ▪ Likely underdiagnosed, so could be higher Characterized by the following features: ▪ Excessive daytime sleepiness ▪ Symptoms that suggest intrusion of REM sleep characteristics into wakefulness Cataplexy (in > 50% of sufferers) Sleep paralysis Dream-like hallucinations while still awake Pathogenesis: ▪ 50% of those with narcolepsy & cataplexy have a clear cause – loss of orexinergic neurons ▪ Pathogenesis is less clear for those without cataplexy Narcolepsy – Clinical Features Cataplexy = sudden muscle weakness without a loss of consciousness ▪ This can be mild – partial weakness of the face or neck ▪ Can be severe – individuals collapse to the ground for minutes, immobile ▪ Often precipitated by an emotional response to something – laughing at a good joke, for example ▪ Very diagnostically useful when present – almost no other disease does this Excerpt from Harrison’s: ▪ “Many disorders can cause feelings of weakness, but with true cataplexy patients will describe definite functional weakness (e.g., slurred speech, dropping a cup, slumping into a chair) that has consistent emotional triggers such as laughing at a joke, happy surprise at unexpectedly seeing a friend, or intense anger.” Video example: https://accessmedicine-mhmedical-com.ccnm.idm.oclc.or g/MultimediaPlayer.aspx?MultimediaID=20083904 Narcolepsy – Clinical Features Excessive daytime sleepiness is kind of an understatement ▪ Several times a day, usually after eating or when bored, the affected person falls asleep – naps usually last 15 minutes or less, and the person is easily aroused ▪ The napping is pretty much irresistible, and it happens 2 – 6 times/day ▪ Automatic behaviour can occur – patient does routine tasks, kind of looks awake, but doesn’t respond appropriately to questions – during attacks The sleep attacks make driving exceptionally hazardous Patients who have other sleep disorders can have a similar presentation, but the napping is usually not as irresistible as in narcolepsy Narcolepsy – Clinical Features Sleep paralysis and hallucinations around the time of falling asleep (hypnagogic hallucinations) or upon awakening (hypnopompic hallucinations) are actually common ▪ Occur in 20% of the general population on an occasional basis ▪ However, usually more frequent in those with narcolepsy Sleep paralysis occurs on awakening, and is often terrifying ▪ Patients can’t move at all for minutes after awakening, some feel that they can’t breathe ▪ Outside stimuli (touch or someone walking into a room) can help terminate the episode Narcolepsy - Pathogenesis Easy to see how loss of orexinergic neurons in the lateral hypothalamus can lead to almost every symptom See poor sleep quality on next slide Rapid transitions into and out of sleep states with “blurring of the borders” between REM and wakefulness Narcolepsy – sleep quality The healthy individual has a long period of NREM sleep before entering REM sleep Narcoleptic enters rapid eye movement (REM) sleep quickly at night and has moderately fragmented sleep Patient with During the day, the healthy subject stays awake narcolepsy from 8:00 dozes off A.M. until midnight frequently, with many daytime naps that include REM sleep Narcolepsy – Pathogenesis and Treatment Why does a patient with narcolepsy lose orexinergic neurons? ▪ Usually autoimmune - HLA DQB1*06:02 (an MHC II gene) is found in > 90% of people with narcolepsy with cataplexy ▪ Molecular mimicry of orexin with common respiratory tract pathogens, including viral influenza and streptococcal species ▪ Narcolepsy with cataplexy is known as Type I narcolepsy Treatment usually involves antidepressants that increase noradrenergic or serotonergic tone – these NTs stimulate REM-off neurons ▪ Methylphenidate or modafinil increase the availability of dopamine at the synapse – this helps stimulate the “arousal nuclei” in the brain stem RLS/PLMD Restless legs syndrome (RLS) – hard to describe, unpleasant, irritating compulsion to move the legs that is triggered by rest, drowsiness, or sleep ▪ Usually interferes with sleep – many report daytime sleepiness due to interruption of sleep Makes it difficult to fall asleep – the symptoms only happen when the subject is awake ▪ Very common – 5 – 10% of adults, more common in women and in older people Periodic limb movement disorder (PLMD) occurs during sleep – movements (often large movements) that frequently involve the legs ▪ Looks like kicking movements RLS/PLMD Pathogenesis is poorly-understood, but knowledge is developing ▪ Strongly associated with iron deficiency, but most patients with RLS do not have peripheral signs of iron deficiency (low ferritin) or anemia ▪ However, studies seem to indicate that deficiency in iron content in the brain is a common finding in RLS patients (established through sampling CSF) ▪ Also seems to associated with abnormalities in dopaminergic signaling – MRI studies suggest that iron transport is particularly impaired in dopaminergic areas such as the substantia nigra RLS/PLMD Pathogenesis is poorly-understood, but knowledge is developing cont… ▪ Abnormalities in iron transport/metabolism may impact a range of dopamine- related processes, including synthesis (tyrosine hydroxylase) and changes in dopamine receptors and transporters ▪ Although overall dopamine metabolites are increased in the CSF, effective treatment involves dopamine agonists ▪ Researchers hypothesize that excessive dopamine activity in the morning and inadequate dopamine activity at night may be the cause RLS/PLMD Movement disorders are the usual clinical consequence of issues that impact the basal ganglia and substantia nigra (extrapyramidal system) ▪ The extrapyramidal system is dependent on dopaminergic transmission ▪ However, how dopamine neurochemistry is related to the discomfort of RLS or the movements of PLMD is not fully understood ▪ The circadian rhythm of dopamine release from midbrain structures that impact movement (lower at night) seems to be exaggerated in those that suffer RLS Obstructive Sleep Apnea Obstructive Sleep Apnea What is it? How is it diagnosed? ▪ Daytime symptoms Excessive daytime sleepiness – difficulty maintaining alertness or involuntary periods of napping ▪ Can actually resemble narcolepsy if sleep deprivation is severe (but no cataplexy, and less likely to experience sleep-associated hallucinations or sleep paralysis) Reports of an unsatisfying or unrefreshing sleep Fatigue and morning headaches Difficulty concentrating, irritability, mood disturbances May also report dry mouth, heartburn, nocturia, and diaphoresis in the upper body OSA - Pathogenesis Inspiration results in negative pressures within the pharynx ▪ We rely on pharyngeal dilator muscles to keep our upper pharyngeal airway open ▪ As neuromuscular tone decreases during sleep, the negative pressure causes the pharyngeal structures to collapse ▪ Sites of collapse include: Soft palate (most common) Tongue base Lateral pharyngeal walls and epiglottis ▪ Unsurprisingly, may be most severe during REM sleep OSA - Pathogenesis Obesity and OSA Excess adiposity, especially in the upper body (androgenic distribution) is strongly implicated ▪ Upper airway fat narrows the pharyngeal lumen ▪ Reduces chest wall compliance (the ease of expanding the chest wall) and can decrease lung volumes Result is that the lungs/chest wall don’t “pull” the upper airway structures downwards as well during inspiration (loss of caudal traction on upper airway structures) ▪ Estimated that 40 – 60% of OSA cases are due to excess weight – 10% weight gain is associated with a > 30% increase in the AHI, a scoring system that ranks apnea/hypopnea while breathing OSA - Pathogenesis Other factors in OSA pathogenesis Smaller or more collapsible pharyngeal lumen – collapses more easily during sleep, and requires more neuromuscular activation to keep patent ▪ Can be narrowed due to enlargement of tongue, palate, uvula ▪ Can be due to smaller jaws or retrognathia (mandible is positioned more posterior) ▪ Lower lung volumes outside of obesity exacerbate the problem (less caudal traction again) ▪ Septal deviation or nasal polyps reduce the pressures that promote air flow further down in the pharynx As well, poor nasal airflow 🡪 mouth breathing 🡪 tongue falls posteriorly and obstructs the pharynx OSA - Pathogenesis Note the seal that the tongue makes on the soft palate with the mouth closed ▪ When the mouth is open, the tongue is free to fall back and seal the upper airway ▪ Nasal obstruction therefore complicates pharyngeal obstruction, even though the mouth may be open OSA - Pathogenesis Other factors in OSA pathogenesis Sensitivity to carbon dioxide concentrations in the bloodstream can also play a role ▪ As carbon dioxide in the bloodstream increases, ventilatory drive (mostly stimulated by medullary nuclei) also increases ▪ In those without OSA, increases in carbon dioxide concentration result in appropriate increases in ventilatory drive and stiffening of upper airway muscles 🡪 patent pharynx If that isn’t enough, then accumulation of carbon dioxide wakes us up ▪ In those with OSA, a low arousal threshold (hypersensitivity) to hypercarbia 🡪 awakening before increasing pharyngeal stiffness Can also cause fluctuations in ventilatory drive that result in periodic airway obstruction and inadequate pharyngeal muscle activation ▪ As well, a high arousal threshold could result in prolonged apneas - not waking up even though the airway is obstructed and significant hypercarbia is occurring OSA – Risk Factors & Pathogenesis Already mentioned ▪ variance in jaw size/structure, upper airway structure, larger tonsils (children with larger tonsils can have OSA) ▪ obesity, variations in ventilatory drive with hypercarbia Genetics – first degree relatives have double the risk of having OSA Male sex – more likely to have upper body obesity, longer pharynx (easier to collapse) Age – 5 – 15% prevalence in middle-aged adults, but > 20% in the elderly Associated with diabetes, hypertension, and atrial fibrillation ▪ Chicken or the egg? OSA – Diagnostic Considerations Sleep history should include information from someone who has observed the patient sleeping, if possible Physical and further investigations should screen for: https:// ▪ Hypertension, cardiac abnormalities en.wikipedia.org/wiki/ Mallampati_score (i.e. signs of CHF or atrial fibrillation) ▪ Oropharyngeal exam should look for causes of obstruction Especially nasal polyps, septal deviation, intra-oral findings as already discussed FYI – higher Mallampati score (see right) increases the risk of OSA OSA – Diagnostic Considerations Gold Standard for OSA diagnosis – polysomnogram ▪ Home sleep tests are economical – they measure nasal air flow, abdominal/chest excursions during breathing, and pulse oximetry (at minimum) High rate of false negatives, and need to be confirmed with formal PSG Other testing – FYI ▪ More advanced cardiac testing (looking for atrial fibrillation, risk of CHF, evaluation of angina if present) ▪ Home BP monitoring ▪ Imaging (CT, MRI) or endoscopy of the upper airway Parasomnias Abnormal behaviours or experiences that arise from or occur during sleep ▪ Sleepwalking (Somnambulism) – automatic motor activities that occur during sleep Occur during N3 sleep, so tend to occur early I the evening Most common in children and adolescents No clear pathophysiology yet identified ▪ Sleep terrors – usually in young children – child awakens either screaming or moaning, and exhibits autonomic signs that indicate fear (also occurs during N3) Tachycardia, hyperventilation, sweating After the attack is over, the child does not remember anything beyond a (mildly) bad dream ▪ For both, often can be avoided by ensuring adequate sleep Parasomnias REM sleep behaviour disorder – one of the few parasomnias that occur during REM sleep ▪ Quite unpleasant – individuals “act out” their dreams and often results in violent movements (punching, kicking) that can injure bed partners or the patient ▪ Prevalence increases with age – unfortunately those with REM sleep behaviour disorder usually develop neurodegenerative disorders after (i.e. Parkinson’s, Lewy body dementia) Seems to predict the development of these later diseases, not cause them ▪ Thought to be caused by neurodegeneration in the interneurons that cause paralysis during REM sleep ▪ See here for a video: https://accessmedicine-mhmedical-com.ccnm.idm.oclc.org/MultimediaPlayer.a spx?MultimediaID=20083906