Learning Induces Long-Term Potentiation in the Hippocampus (Whitlock) PDF
Document Details
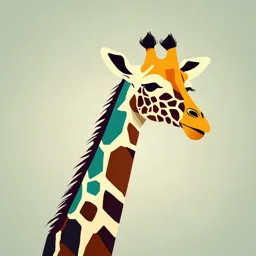
Uploaded by BallerGiraffe0118
Concordia University
Tags
Summary
This document explores the relationship between learning and long-term potentiation (LTP) in the hippocampus. It highlights the difficulty previous studies had in establishing a causal link and describes experiments to solve these issues.
Full Transcript
Learning Induces Long-Term Potentiation in the Hippocampus (Whitlock) Previous papers investigated hippocampal LTP but none were able to establish a causal link between hippocampal LTP and learning due to 3 issues: 1. many hippocampally dependent learning tasks are iterative, and so require many...
Learning Induces Long-Term Potentiation in the Hippocampus (Whitlock) Previous papers investigated hippocampal LTP but none were able to establish a causal link between hippocampal LTP and learning due to 3 issues: 1. many hippocampally dependent learning tasks are iterative, and so require many training trials to form a memory 2. synaptic changes that underlie hippocampally dependent learning may be sparse and widely distributed, which would make potentiated synapses difficult to detect 3. information can be stored effectively by long-term depression (LTD), as well as by LTP. Simultaneous induction of LTP and LTD at different synapses may cancel one another This papers solves them by: Problems 1&2 was solved by using the inhibitory avoidance (IA) paradigm since IA training creates a stable memory trace in a single trial and causes substantial changes in gene expression in area CA1 of dorsal hippocampus Problem 3 was solved with the use of new biomarkers that can detect LTP and LTD occurring at different synapses Exp 1: Hippocampal GluR are phosphorylated after IA training Design: IA training: allowing rats to cross from an illuminated chamber into a dark chamber where a foot shock was delivered Walk-through controls: entered the dark side without receiving a shock Shock-only controls: were given a foot shock in the dark side and immediately removed from the apparatus before they could form the context-shock association Naive: remained in the recording box without being handled To see if training-induced phosphorylation of Ser831, like LTP and memory, requires NMDA receptor activation: CPP group: rats injected with a selective NMDA receptor antagonist to block NMDAR Saline group: rats injected with saline AMPAR (GluR1 subunit) undergoes different phosphorylation events that alter the function of AMPAR and contribute to the expression of LTP/LTD & “mark” synapses as having recently undergone modification: Serine 831 is phosphorylated after LTP Serine 845 is phosphorelated after LTD Results: An increase in phosphorylation at Ser831 30 min following IA training (LTP) No difference in phosphorylation at Ser845 between trained and walk-through animals (no LTD) No increase in Ser831 phosphorylation observed in the CPP group = blocking of NMDAR blocks IA learning Increase seen in saline group Experiment #2: IA training causes delivery of AMPARs Synaptoneurosomes provide a way to study synaptic activity and protein synthesis at synapses LTP is associated with the delivery of AMPARs to synapses, and LTD is associated with the removal of AMPARs from synapses Results: IA-trained animals showed significantly elevated GluR1 and GluR2 protein levels increases in AMPAR protein levels were specific to associative learning Exp 3: IA training produces an enhancement of eld excitatory postsynaptic potential (fEPSP) slope in area CA1 in vivo Design: Stimulation of Schaeffer Collateral Axons via SE and implantation of RE array in the area CA1 of hippocampus Measure the changes in fEPSPS slopes pre- and post- IA training Results: Average of RE shows only a small increase in fEPSP slopes Why? Because only a subpopulation of RE in IA-trained group showed an increase in fEPSP slopes (Hebbian cell assembly theory: specificity) The increase persisted for up to 2h after training IA-training is the only group showing an increase in fEPSP slopes relative to baseline Ratio of all REs with changes greater or less than 1 standard deviation (SD) from the baseline Only IA-trained group shows a high ratio of REs of changes of over 1 SD above baseline Some REs show a change of over 1 SD below baseline, because: Habituation Parallel LTD-like changes (but no expression of Ser845) Some neurons are made to balance out LTP (similar to synaptic pruning) to prevent over-excitation of neurons that lead to excitotoxicity IA-trained group was the only group that showed a statistically significant within- variance increase (256%) Exp 4: Learning-related enhancements of fEPSP slope occlude subsequent LTP in vivo Design: fi Same experiment as Exp 3, but with an additional group that received a high- frequency stimulation to saturate LTP Results: Inverse correlation between the amount of behaviourally-induced potentiation and the amount of LTP induced by HFS REs where fEPSPs were enhanced after IA showed less subsequent LTP in response to HFS (occlusion) REs that did not exhibit enhancements of fEPSPs after IA training showed a greater magnitude of subsequent LTP Regulation of Synaptic E cacy by Coincidence of Postsynaptic APs and EPSPs (Markram) Primary Objective: how the timing between excitatory post synaptic potentials (EPSPs) and acton potentials (APs) in neocortical neurons affects synaptic strength, suggesting that precise timing could be key in synaptic modifications Temporal summation: EPSP from same pre- neuron add up over time Spatial summation: EPSP from many pre- neurons combine and add up HFS > increased EPSPs > LTP LFS > increased IPSPs > LTD Spike-timing Dependent Plasticity: timing between APs and EPSPs determines synaptic strength EPSPs is before AP = LTP EPSPs is after AP = LTD Exp 1: Design: Electrophysiology in vitro: Intracellular recordings in inter-connected neurons (necessary for this experiment) with a dual- electrode that provide simultaneous stimulation Phase 1: stimulation of pre-synaptic neuron = small EPSP in post-synaptic neuron Phase 2: after each AP from the pre-synaptic neuron, an artificial current is injected in the post-synaptic neuron Results: ffi APs from pre-synaptic neurons alone do not generate a change in EPSPs Artificial APs in post-synaptic neurons cause enhancements in EPSPs (LTP) Exp 2: Design: Pharmacological blocking of NMDAR with antagonist (Lidocaine) New group: Sustained depolarization condition: depolarization of the pre-synaptic neurons but right under threshold = no AP is triggered Results: The increase in EPSP amplitudes increases with frequency (above 10Hz) Frequency under 10Hz are not enough to induce EPSP = no LTP Sustained depolarization is not substantial enough for LTP Back-propagating APs facilitate synaptic strengthening Definition: electrical signal that travels backward from the axon into the neuron's dendrites, instead of just moving forward Blocked NMDAR = less Ca2+ into neurons Exp 3: Role of Timing Design: Stimulated burst of AP in Cell 1 causing EPSPs in Cell 2 Wait (10ms or 100ms) Stimulated burst of AP in Cell 2 causing EPSPs in Cell 1 Results: APs and EPSPs must coincide within 100ms to induce changes in EPSPs: Post-synaptic neuron’s APs 10ms before EPSPs = reduced average EPSP amplitude (LTD) Post-synaptic neuron’s APs 10ms after EPSPs = increased average EPSP amplitude (LTP) Discussion Post-synaptic neuron AP is essential for EPSP increase Post-synaptic membrane only shows an increase in ratio of AMPAR The increase in EPSPs is dependent on the frequency of AP-EPSP paired Sharp onset at 10Hz Back-propagating & BCM Theory NMDAR activation allows more Ca2+ into neuron BCM: the stronger the connection is, the more it needs Ca2+ to maintain it If not enough Ca2+ influx = AMPAR will be brought back to intracellular stores