Week 4 Stereochemistry Workbook - Monash - PDF
Document Details
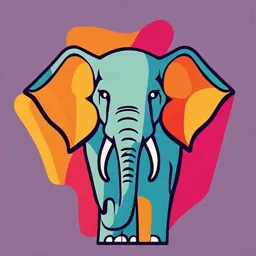
Uploaded by ExceedingChrysoprase7632
Monash University
2024
Kaltham Alzaabi
Tags
Summary
This document is a workbook on stereochemistry, part of a Chemistry II course at Monash University for the S2 2024 semester. It covers topics including enantiomers, chiral and achiral molecules, and assigning configurations.
Full Transcript
8/11/24, 6:20 AM Week 4: Stereochemistry - workbook | MonashELMS1 Week 4: Stereochemistry - workbook Site: Monash Moodle1 Printed by: Kaltham Alzaabi...
8/11/24, 6:20 AM Week 4: Stereochemistry - workbook | MonashELMS1 Week 4: Stereochemistry - workbook Site: Monash Moodle1 Printed by: Kaltham Alzaabi Unit: CHM1022 - Chemistry II - S2 2024 Date: Sunday, 11 August 2024, 6:19 AM Book: Week 4: Stereochemistry - workbook https://learning.monash.edu/mod/book/tool/print/index.php?id=2779905 1/20 8/11/24, 6:20 AM Week 4: Stereochemistry - workbook | MonashELMS1 Table of contents 1. Pre-workshop material 1.1. Enantiomers 1.2. Molecules with more than one stereocentre 1.3. Chirality 1.4. Importance of chirality 1.5. Assigning configuration 1.6. Optical Activity 1.7. Assigning absolute configuration video 1.8. Activity 1 1.9. Solution: Activity 1 1.10. Three-dimensional structure of molecules 2. Preparation quiz 3. Online lectures https://learning.monash.edu/mod/book/tool/print/index.php?id=2779905 2/20 8/11/24, 6:20 AM Week 4: Stereochemistry - workbook | MonashELMS1 1. Pre-workshop material Isomerism Isomerism is a concept introduced to you in week 1. Isomers are molecules which possess the same molecular formula but differ in structural connectivity. Stereochemistry is the of the study of three-dimensional shape of molecules and how this affects chemical and physical properties. Certain molecules have the same connectivity but differ in how they arrange themselves in space. These optical isomers, also called stereoisomers, differ in the way they rotate plane-polarised light. There are two types of stereoisomers that we will encounter: enantiomers and diastereoisomers. Please read Sections 17.1-17.7 of Chemistry, Blackman et al. (4th ed.) https://learning.monash.edu/mod/book/tool/print/index.php?id=2779905 3/20 8/11/24, 6:20 AM Week 4: Stereochemistry - workbook | MonashELMS1 1.1. Enantiomers Enantiomers An enantiomer is one of the two molecules that are mirror images of each other and are non-superimposable. One way to imagine what this would look like is to look at your own hands. Your left hand is a mirror image of you right hand (and vice versa) (ignoring fine detail of course!). Your hands, however, cannot be superimposed upon one another. The same is true for enantiomers. Take Figure 1 for example; no matter how you rotate the molecules shown (which are mirror images of one another), they will never be able to be superimposed. If this is difficult for you to imagine, a model kit will help you visualise this. Molecules that are non-superimposable mirror images of one another are said to be chiral. Chirality is a property found in DNA, proteins and sugars and many other molecular species. Chirality is a property which can also be found in everyday objects too. Figure 1: Two enantiomers and their non-superimposable nature. A compound or object which is superimposable on its mirror image is referred to as achiral. Achiral molecules have a plane of symmetry (Figure 2). Figure 2: Achiral molecules and their superimposable nature. Can you see the key difference between the molecules in Figure 1 and Figure 2 which renders the molecule chiral or achiral? You may have figured out by comparing Figure 1 and Figure 2 that in order for a tetrahedral centre to be chiral it must have four different substituents attached to it and thus have no plane of symmetry. The most common type of enantiomerism in organic molecules is the tetrahedral carbon atom with four different substituents. This centre can also be referred to as an asymmetric carbon centre or as a stereogenic centre. In order for a carbon to be achiral, the carbon must bear two identical substituents in order for the mirror image of itself to be superimposable. https://learning.monash.edu/mod/book/tool/print/index.php?id=2779905 4/20 8/11/24, 6:20 AM Week 4: Stereochemistry - workbook | MonashELMS1 We represent chemical structures with lines representing bonds, however, this representation gives no information about stereochemistry associated with the molecule. Take the example shown in Figure 3. The pictures on the left-hand side of the page give no information on how the substituents are arranged in space. These representations only provide information on connectivity of atoms. In order to represent spatial arrangements of bonds, chemists have come up with the system which utilises wedge-dash notation in order to represent molecules as being three-dimensional. In this notation, two bonds are drawn as sticks to represent them being in the plane of the page, one bond is drawn as a solid wedge to represent the bond being projected towards the viewer and one dashed bond to represent the bond being projected away from the viewer. An absolute configuration refers to the spatial orientation of the components of chiral chemical compounds. R and S are used to describe the stereochemical orientation and will be discussed in depth later. Figure 3: Notation used to represent three-dimensional spatial arrangement of molecules. Please see Section 17.2 of Chemistry, Blackman et al (4th ed.) https://learning.monash.edu/mod/book/tool/print/index.php?id=2779905 5/20 8/11/24, 6:20 AM Week 4: Stereochemistry - workbook | MonashELMS1 1.2. Molecules with more than one stereocentre The previous section was restricted to molecules with one stereocentre. However, it is possible for a molecule to have more than one stereocentre. In general, a molecule with n stereocentres will have a maximum of 2n stereoisomers. Consider 2,3,4-trihydroxybutanal (Figure 4). It has two stereogenic centres, therefore the maximum number of stereoisomers possible is 22 = 4 as shown in Figure 4. There are two pairs of enantiomers: (a) and (b) are one pair, the other pair is (c) and (d). (a) and (b) are enantiomers and are non- superimposable mirror images. Likewise, (c) and (d) are enantiomers and are non-superimposable mirror images. In order to discuss the relationship of (a) and (c) for example, we must introduce another type of stereoisomerism called diastereoisomerism. Diastereoisomers are stereoisomers which are not enantiomers (not mirror images of each other). Diastereoisomer pairs in this case are: (a) and (c), (a) and (d), (b) and (c) and (b) and (d). The absolute configuration (R/S) is denoted in the following way using (a) as an example (2R, 3R)-2,3,4-trihydroxybutanal meaning the configuration at carbon 2 is R and R at carbon 3. Figure 4: Stereoisomers of 2,3,4-trihydroxybutanal In the case of 2,3-dihydroxybutanedioic acid, known as tartaric acid (Fig. 5), there are two stereogenic centres. You may expect there to be 22 = 4 stereoisomers. However, the two chiral centres of tartaric acid have the same four substituents and are equivalent. Due to this, two of the four possible stereoisomers (c) and (d) are identical due to the plane of symmetry as shown in Figure 5. Consequently, there are only three stereoisomeric tartaric acids. Two of these are enantiomers, (a) and (b). The third is an achiral disastereomer referred to as a meso compound. These meso compounds (c) and (d) are the same molecule orientated differently and are superimposable. Meso compounds are achiral compounds with two or more stereocentres and are optically inactive. Figure 5: Stereoisomers of tartaric acid 1,2-Cyclopentanediol has two stereocentres, again using the 2n rule you may expect there to be 22 = 4 stereoisomers (Figure. 6). However, as we saw with the previous case, only three stereoisomers exist for this compound. The cis-isomer possesses a plane of symmetry and hence an achiral meso compound because it is superimposable on its mirror image. The trans isomer shown on the right-hand side is a pair of enantiomers. https://learning.monash.edu/mod/book/tool/print/index.php?id=2779905 6/20 8/11/24, 6:20 AM Week 4: Stereochemistry - workbook | MonashELMS1 Figure 6: Stereoisomers of 1,2-cyclopentanediol Please see Section 17.4 of Chemistry, Blackman et al. (4th ed.) https://learning.monash.edu/mod/book/tool/print/index.php?id=2779905 7/20 8/11/24, 6:20 AM Week 4: Stereochemistry - workbook | MonashELMS1 1.3. Chirality Watch this short TED-Ed video by Micheal Evans that introduces the concept of chirality. https://learning.monash.edu/mod/book/tool/print/index.php?id=2779905 8/20 8/11/24, 6:20 AM Week 4: Stereochemistry - workbook | MonashELMS1 1.4. Importance of chirality Many molecules in nature exhibit chirality with a certain “handedness” displayed. Chirality is extremely important in biology because many receptors of organisms require a specific handedness. As a result of this, chemists when designing pharmaceuticals must take into account enantiomers when a specific receptor is being targeted. The importance of chirality was highlighted when a new drug called thalidomide was introduced to the market in 1957 as a sedative and sleeping drug for pregnant women. Thalidomide contains one chiral carbon and was marketed as the racemic mixture of the R and S enantiomers. Tragically, thalidomide was found to have dire side-effects with thousands of babies born with birth defects. It is now known that it is the S enantiomer is teratogenic and causes sever side-effects while the R enantiomer has a sedative effect. One key difference of enantiomers is the fact that they react differently towards other chiral molecules. Protein molecules are themselves chiral, hence they can interact differently with the enantiomers of a chiral drug. This specific case forced pharmaceutical companies to consider enantiomers are separate molecules rather than different forms of the same drug. https://learning.monash.edu/mod/book/tool/print/index.php?id=2779905 9/20 8/11/24, 6:20 AM Week 4: Stereochemistry - workbook | MonashELMS1 1.5. Assigning configuration Assigning configurations to stereogenic centres A chiral centre can have two possible configurations R and S. The letter R and S are derived from the Latin words for right and left, 'rectus' and 'sinister'. In order to assign a stereogenic centre as either R or S you need to follow three steps using Figure 7 as a visual guide: 1. Locate the stereogenic centre and identify the four different substituents. 2. Assign priorities (1 to 4) to all substituents attached to the chiral centre (see below for steps in order to do this). 3. Rotate the group or atom with the lowest priority (priority 4) so it is orientated away from you. Next, draw a curve from the first- priority substituent through to the second, and then the third substituent. Determine if the direction of this priority curve is clockwise (R) or anticlockwise (S). Figure 7: Visual guide of assigning R and S configurations to chiral centres Rules to follow in order to assign priorities of substituents: 1. Assign priorities of substituents according to the Cahn-Ingold-Prelog prioritisation scheme which gives the highest priority to the atom with the highest atomic number. H, C, N, O, F, S, Cl, Br, I (increasing priority) 2. If the two atoms or substituents are the same (for example if there are two CH2 groups attached to the stereogenic carbon), proceed to the next position of the chain. You may need to go even further until the first point of difference. 3. Groups with Π-bonds are given single-bond equivalents by duplication or triplication of multiply bonded atoms. e.g. Take the example 1-bromo-1-chloroethane for example (Figure 8). It has one stereogenic centre. Can you locate the stereogenic carbon? Figure 8: Structure of the chiral molecule 1-bromo-1-chloroethane https://learning.monash.edu/mod/book/tool/print/index.php?id=2779905 10/20 8/11/24, 6:20 AM Week 4: Stereochemistry - workbook | MonashELMS1 As this carbon has a stereogenic center, the name 1-bromo-1-chloroethane is incomplete as the configuration of the stereogenic centre is missing. Next we must assign the priorities according to the Cahn-Ingold-Prelog prioritisation scheme. Using this scheme Br (1) > Cl (2) > CH3 (3) > H (4). The hydrogen atom which is assigned the lowest priority (4) must be orientated away from the viewer. In this case, the hydrogen is already orientated away from us. Next we must draw a curve from the first-priority substituent through to the second, and then the third substituent. This is done in Figure 9 for you. As this curve is clockwise the configuration is R, therefore, (R)-1-bromo-1-chloroethane. Figure 9: Priority assignment of 1-bromo-1-chloroethane Assigning priorities may not always be straight forward as with the example shown in Figure 10. Let’s work through this example together. The stereogenic centre is shown for you but convince yourself this is correct. Figure 10: Structure of 3-ethyl-3-methyl octane Go to the substituents of the stereogenic centre. You will see that there are three CH2 groups attached and one CH3 group. As C > H in the prioritisation system the CH3 group shown in red, in this case has the lowest priority 4. Because there is a tie between the other three carbon chains, we must move to the next position along the carbon chain. You will see that there is another tie between the green and blue positions which are substituted by a carbon and two hydrogens. The pink position has three hydrogen substituents, C > H rendering the pink position the priority of 3. https://learning.monash.edu/mod/book/tool/print/index.php?id=2779905 11/20 8/11/24, 6:20 AM Week 4: Stereochemistry - workbook | MonashELMS1 In order to assign the priority 1 and 2 we must move to the next point of difference. The green carbon has one carbon and two hydrogen substituents while the blue position has three hydrogen substituents. C > H rendering the green position priority 1 and the blue position priority 2. → Next, we position priority 4 away from us and draw a circle from 1 through 2 and finally through 3. This proceeds clockwise giving the R enantiomer. See Section 17.3 of Chemistry, Blackman et al. (4th ed.) https://learning.monash.edu/mod/book/tool/print/index.php?id=2779905 12/20 8/11/24, 6:20 AM Week 4: Stereochemistry - workbook | MonashELMS1 1.6. Optical Activity Chiral molecules are very similar to each other as they have the same molecular arrangements. They only differ in their spatial arrangement. Due to this enantiomers have the same boiling point, melting point and solubility. The only physical property in which enantiomers differ is how they interact differently with plane-polarised light. The plane of plane-polarised light is rotated which light passes through a solution of the enantiomer. This property of a chiral compound is called optical activity. The rotation is called optical rotation. What is plane-polarised light? In plane polarised light, waves are oscillating in a single plane perpendicular to the direction of propagation of light while in ordinary light, oscillations occur in all planes (Figure 11). Figure 11: Creation of plane-polarised light When light passes through a solution containing a chiral molecule, the light is able to interact with the molecule's electron cloud. It is this interaction that can lead to the rotation of plane-polarised light. Because the light in plane-polarised light is travelling in one direction, we are able to determine if a molecule is chiral if the direction of the plane-polarised light has been changed and becomes rotated. The direction and magnitude of rotation depends on the electron cloud of the molecule. Two enantiomers will rotate plane- polarised light by the same magnitude in opposite directions. This is why racemic mixtures (a mixture of equal amounts of two enantiomers) are optically inactive – the rotation is cancelled out as they rotate plane-polarised light in exactly equal amounts in opposite directions. The rotation of plane-polarised light of an enantiomer can be measured using a polarimeter. When a beam of plane-polarised light is rotated counterclockwise the molecule is the (-, L) form. When a beam of plane-polarised light is rotated clockwise the molecule is of the (+, D) form. Do not confuse the two notations of (-, L)/ (+, D) and R/S. The +/- indicate the direction that an enantiomer rotates polarised light and R- and S- groups indicate the arrangement of groups at the stereogenic centre. Thus optical activity sign (+/-) is independent of the R/S. For example, (R)-2-hydroxypropanoic acid and (S)-2,3-dihydroxypropanal are both negative. See Section 17.5 of Chemistry, Blackman et al. (4th ed.) https://learning.monash.edu/mod/book/tool/print/index.php?id=2779905 13/20 8/11/24, 6:20 AM Week 4: Stereochemistry - workbook | MonashELMS1 1.7. Assigning absolute configuration video Cahn-Ingold-Prelog convention The video is a visual aid in order to help you assign R and S absolute configuration. By the end of this video you should be able to identify chiral carbons, assign priorities using the Cahn-Ingold-Prelog convention and assign the absolute configuration. Cahn-Ingold-Prelog Convention (Determining R/S) https://learning.monash.edu/mod/book/tool/print/index.php?id=2779905 14/20 8/11/24, 6:20 AM Week 4: Stereochemistry - workbook | MonashELMS1 1.8. Activity 1 Identify the stereogenic centre of the following two molecules. https://learning.monash.edu/mod/book/tool/print/index.php?id=2779905 15/20 8/11/24, 6:20 AM Week 4: Stereochemistry - workbook | MonashELMS1 1.9. Solution: Activity 1 https://learning.monash.edu/mod/book/tool/print/index.php?id=2779905 16/20