Week 2 - Sedimentation, Elfo, MS Booklet PDF 2022
Document Details
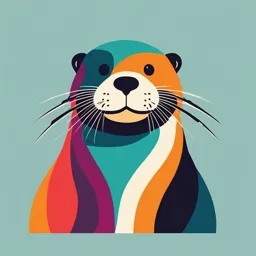
Uploaded by ComfortingAestheticism
University of Debrecen
2022
Tibor G. Szántó
Tags
Summary
This document provides an overview of sedimentation, electrophoresis, and mass spectrometry techniques in biophysics, molecular, and cellular diagnoses of diseases. It covers principles, methods, and medical relevance. The document is aimed for a postgraduate level learning environment.
Full Transcript
Sedimentation, electrophoresis and mass spectrometry The text under the slides was written by Tibor G. Szántó 2022 This instructional material was prepared for the biophysics lectures held by the...
Sedimentation, electrophoresis and mass spectrometry The text under the slides was written by Tibor G. Szántó 2022 This instructional material was prepared for the biophysics lectures held by the Department of Biophysics and Cell Biology Faculty of Medicine University of Debrecen Hungary https://biophys.med.unideb.hu 2 The slide summarizes the main aims and topics of the lecture. Cells and their macromolecules play a crucial role in vital processes of living organisms. You are going to get introduced the the procedures and physical methods that serve to reveal the structure of molecules, macromolecules and macromolecular complexes. Therefore, these methods help to explore the molecular background of structural distortion of certain diseases. During the lecture you will see the principles of different sedimentation techniques, gel electrophoresis and isoelectric focusing. Furthermore, we are going to discuss how mass spectrometers work, since mass spectrometers are more and more used both in research and diagnostics nowadays. The major aims are to understand the basic concepts of the frequently used physical and biological methods that are used to separate and identify biologically relevant macromolecules such as proteins and nucleic acids based on their mass and/or charge as well as the determination of the composition of macromolecules such as the amino acid sequence of proteins. 3 In biophysics and cell and molecular biology it is often required to separate biologically relevant macromolecules based on their size or to characterize them based on their size and density. Sedimentation techniques achieve these aims by examining the sedimentation of molecules in a strong „gravitational” field in centrifuges. Why do we need a strong gravitational field for this? An accepted method for the determination of mass is based on the measurement of the acceleration of the mass of interest exposed to the force of gravity. However, for dissolved particles the force of gravity is not the most practical method due to the thermal motion of solvent molecules. For example, as the calculations show if a particle with a mass of M covers a distance of 10 cm upon sedimentation the gravitational potenial energy is similar in magnitude to the thermal energy. Consequently, molecules do not sediment in the Earth’s gravitational field. Much stronger gravitational field can be provided by fast spinning. Centrifugation results in much higher acceleration than g. According to the equations, if the angular speed is set to 1000 RPM (revolutions per minute) and the radius of centrifuge is 10 cm, the centripetal acceleration will be 112-times greater than the gravitational acceleration. 4 Sedimentation techniques can be classified into the following three types: sedimentation velocity method, density gradient centrifugation and the sedimentation equilibrium method. The table shows those properties that can be determined by each technique. The sedimentation velocity method is an analytical ultracentrifugation method that measures the rate at which molecules move due to a centrifugal force. Let us consider a vial containing a solution of molecules with a mass of m spinning about a vertical axis with an angular velocity of . Let the density of the medium be 0, while the density of the solvent/particle is . Three forces are acting on a molecule sedimenting in a centrifuge tube: the centrifugal force pointing away from the axis of rotation, and both frictional force and buoyant force pointing towards the axis of rotation. Each force component can be expressed using basic physical concepts (see Equations). In equilibrium the net force on the particle is zero thus, the particle does not accelerate, it sediments at constant speed. More specifically, the velocity of the particle increases only in proportion to the increasing distance from the axis of rotation. 5 The difference of centrifugal and bouyant forces makes the particles move outward from the axis with accelerating movement until it reaches equilibrium with the counteracting frictional force. Let us define each force and then combine and rearrange them in order to express the sedimentation coefficient (S), i.e. the sedimentation velocity upon unit acceleration. The non-SI unit of sedimentation coefficient is the svedberg, 1 Sv = 10-13 s named after Theodor Svedberg who recieved the Nobel prize for his work on disperse systems. Once S is determined, it can be used to calculate m. The drawback of this expression is that it contains the form factor, f. It is known that f can be correlated with D using the formula shown at the bottom of the slide. Consequently, by combining diffusion measurements with the sedimentation velocity method one can calculate the molar mass of a molecule quite precisely without using any assumption regarding the shape of the molecule. This method may also be used for the determination of the size of molecules provided that certain data regarding the shape of a molecule is given. Experience shows that the sedimentation coefficient decreases with increasing concentration. To circumvent this problem m is determined from S that is extrapolated to zero concentration. 6 The sedimentation velocity method is used to separate molecules according to their sedimentation coefficient. In this method a very strong gravitational field is produced up to one million g. Molecules can be separated by parameters such as molecular weight and size, since the sedimentation coefficient is greatly affected by these parameters. In general, the larger a particle, the larger its sedimentation coefficient is. This method is suitable for separating antibody monomers and oligomers, as shown in the graph on the slide. The individual particles can be separated by centrifugation based on their sedimentation coefficients, and then their concentrations can be determined. 7 The sedimentation equilibrium method is used to determine the molecular weight of solvent molecules. In this method the average sedimentation velocity of the molecules should reach zero. Technically, the method is usually performed at lower revolutions per minute and/or for a long time (for several hours or more than one day) to achieve equilibrium between sedimentation and thermal motion/diffusion. If the centrifugal force is the only force affecting the molecules, they would gather at the bottom of the vial (the region of lowest energy). The thermal energy of Brownian motion opposes this and some of the molecules reach higher energy. The smaller a molecule is, the farther away it can diffuse from the bottom of the vial. The figure at the bottom of the slide shows the separation of two molecules having different molecular mass (MW). Since molecule #1 is lighter than molecule #2, it can move farther away from the bottom. The molecular weight can be determined from the slope of the curve, as the slope is proportional to the molecular weight. The adventage of this method is that the results are independent of the form factor and the diffusion coefficient. To the contrary, the sedimentation velocity method yields unreliable molecular weight, if there are no sufficient data regarding the shape of the molecule. A further problem with the sedimentation velocity method is that the molecules are solvated during sedimantation thus R is no longer the real molecular radius. The shape and the altered R due to the solvation shell influence the velocity of sedimentation. 8 Density gradient centrifugation is used to determine the density of a molecule or particle (such as cells, cell organelles etc.) or to separate molecules based on their density. In this method the centrifugation is performed in a density gradient. The tube is filled with a solution of low molecular weight molecules with high density (e.g., CsCl) resulting in a density gradient in such way that the concentration of the loaded CsCl solution decreases evenly, i.e., the concentration is the highest at the bottom and the lowest at the top of the tube. The sample is layered on the top layer. When macromolecules are centrifuged in such medium, they stop when they reach a region in which their density () is the same as that of the medium (0). At this point the net force acting on the molecules becomes zero (i.e., the centrifugal force (Fc) is equal to the buoyant force (Fb)). Therefore, the particles will "float", that is the particles accumulate in this layer. If the particle is above or below its equilibrium position, it will experience a net force such that it is accelerated toward its equilibrium position. The adventage of this method is that the components can be separated regardless of their size, solely based on their density. Homogeneity of different samples can also be studied this way. 9 Density gradient centrifugation can be used to separate the cells in the blood that is often needed in clinical diagnostics and research. Blood consists of plasma (approx. 56%) and blood cells (approx. 44%). There are three main types of blood cells having different densities: red blood cells (erythrocytes), white blood cells (leukocytes) and thrombocytes (platelets). The density of lymphocytes and monocytes is the smallest, the density of granulocytes is higher and the density of red blood cells is the highest. Layering diluted blood onto the top of a separation fluid, whose density is higher than that of mononuclear cells but smaller than the density of granulocytes, and applying high gravity by centrifugation mononuclear cells can be separated. Mononuclear cells stay on top of the separation fluid, but the other cells sediment to the bottom of the tube. The figure on the right shows the result of such a density gradient centrifugation of blood: at the end of the centrifugation the plasma is situated on the top, the thin opaque layer between the plasma and the separation fluid contains the mononuclear cells. The bottom layer contains the erythrocytes and the granulocytes. Mononuclear cells can be harvested from the opaque layer. Granulocytes can be separated form erythrocytes by dextran sedimentation of the bottom layer (pellet). 10 Under physiological conditions most biologically relevant macromolecules are charged. Placing such a charged molecule into an electric field will result in its migration in a direction according to its electric charge. Translational movement of particles and molecules in a solution due to an electric field is called electrophoresis. A molecule with ze charge placed in an electric field characterized by field strength of E is affected by a (Coulomb) force equal to Zfe. The Coulomb force exerted by the electric field forces the molecule to move with increasing velocity until its the frictional force, proportinal to its velocity, counterbalances the Coulomb force. Based on the equations shown on the slide we can introduce a new quantity called electrophoretic mobility (u) that is given as u=v/E, or u=ze/f, where f is the form factor and v is the speed of the particle. The form factor is proportional to the hidrodynamic radius of the molecule and consequently the molecular weight shown by the Equations. By definition, the electrophoretic mobility is the electrophoretic velocity in unit electric field. Since electrophoretic mobility depends on the charge (Q) and the size (that correlates to the molecular weight) of molecules, electrophoresis is used for separating molecules based on their charge and size. 11 Free electrophoresis is a process that does not use any solid phase such as a gel, thus charged particles move in a solution. The sensitivity of the technique is so high that even a charge difference of one electron per molecule can be detected. The drawbacks of this technique are the difficult interpretation of mobility data since diffusion is not negligible due to the low viscosity of the medium (aqueous solution) and furthermore, the high price of the electrophoretic chamber. It cannot be applied for preparative purposes because only small amount of sample can be isolated i.e., the fastest and slowest moving components. Therefore, free electrophorersis is rarely used nowadays. Gel electrophoresis is a technique that is widely used for separating DNA, RNA, and protein molecules according to their molecular weight. In this method, electrophoresis takes place in a matrix (or a gel) that almost completely eliminates diffusion of molecules. Thus, the gel serves as an antidiffusive and sieving medium. With gel electrophoresis the components of a very small amount of sample can be fully separated with very high reproducibility. The separated fractions then can be fixed, stained with different techniques or labelled and evaluated by one of numerous methods. For the separation of nucelic acids the gel is made of agarose. In contrast, for proteins polyacrylamide gel electrophoresis is used. 12 Agarose gel electrophoresis is the easiest and most popular way of separating and analyzing DNA. Agarose is a polysaccharide obtained from the red algae Porphyra umbilicalis. Agarose makes an inert matrix. The other component of agar is agaropectin, that is a heterogeneous mixture of oligosaccharides. The figure below shows the construction of an electrophoresis tank containing an agarose gel slab. The electrophoresis tank is filled with a buffer solution with proper ionic strength to cover the gel completely. Then, nucleic acid is loaded into a well close to the negative electrode with a pipette. As the charge of nucleic acids is negative, they migrate to the anode (positively charged electrode) if voltage is applied. Since nucleic acids are not visible in natural light, the progress of electrophoresis is monitored using colored dyes. 13 Nucleic acids are negatively charged at neutral pH due to the presence of phosphate groups. The migration rate of DNA is mainly affected by factors such as size of the DNA, agarose concentration used and conformation of the DNA. The rate of migration of molecules in gel electrophoresis is inversely proportional to the size of the molecule. Large molecules migrate more slowly than small ones that can move more easily through gel pores. In other words, the gel sieves molecules based on their size. Thus, large components accumulate farther away from the positive electrode after switching off the electric field. 14 Agarose gel electrophoresis is one of the traditional methods of separating and analyzing nucleic acid in molecular biology. This method is available for separating both single-stranded and double-stranded DNA and RNA based on their size. The size of the fragments can be determined by running a standard DNA ladder (marker) in parallel. The size of DNA is given in bp (base pair). The length of DNA in the experimental sample is compared to the known length of DNA in the marker. Nucleic acids can be visualized in the gel by the addition of ethidium bromide that fluoresces strongly under UV exposure when bound to double- stranded nucleic acids. Each fluorescent band corresponds to nucleic acid with a certain size. 15 In contrast to DNA molecules, proteins are structurally very diverse due to the huge variation in their amino acid compositions and the distribution of amino acids in their folded structures. Because proteins are so diverse with respect to their surface charges and geometries, the molecular weights of folded proteins cannot be simply determined by their migration rate in an electric field. In order to solve this problem, proteins must be converted to a uniform geometry and they must be given a uniform charge. In SDS-PAGE, the proteins are denaturated by boiling them with the anionic detergent, sodium dodecyl sulfate (SDS) and 2- mercaptoethanol. The combination of heat and detergent is sufficient to break the many noncovalent bonds that stabilize protein folds, and 2-mercaptoethanol breaks any covalent bonds between cysteine residues. Like other detergents, SDS is an amphipathic molecule, consisting of a hydrophobic 12-carbon chain and a hydrophilic sulfate group. The hydrocarbon chain permeates the protein interior and binds to hydrophobic groups, reducing the protein to a random coil, coated with negatively charged detergent molecules all along its length. On average one SDS molecule binds to every second amino acid. Then, the SDS-coated proteins are electrophoresed in a gel made of polyacrylamide. The polyacrylamide gels are formed by the chemical polymerization of acrylamide and a cross-linking reagent, N,N’methylenebisacrylamide. The size of the pores in the gel can be controled by adjusting the concentration of acrylamide, as well as the ratio of acrylamide to bisacrylamide. 16 During electrophoresis the uniformly negatively charged proteins migrate at different rates depending on their size. The mobility of the SDS-denaturated proteins is approximately inversely proportional to the logarithm of their molecular weight. Thus, the proteins are separated by their molecular weight. Similar to the agarose gel electrophoresis of nucleic acids, markers are used for determining molecular weight. A molecular weight ladder containing proteins with well-defined molecular weight is run in the same gel with sample. On the basis of the migration of these „standard proteins” a calibration curve can be drawn, which is used to read the molecular weight of the proteins to be determined. SDS- polyacrylamide gel electrophoresis is the most common, inexpensive method for proteins not only for determining their molecular weight but also for the separation of proteins based on their weight. It is also possible to perform quantitaive measurments by staining the proteins in the gel. Proteins can be detected by autoradiography or chemiluminescence. 17 Proteins are amphoteric macromolecules as they contain both acidic and alkaline functional groups. Therefore, they can alter their net charge as a result of changes in the pH of the medium. There is a specific pH value at which their net charge is zero as the number of positive and negative charges is equal. This pH is called the isoelectric point. Above the isoelectric point proteins possess negative charge, while at low pH they are positively charged. The aim of this technique is to find the isoelectric point of a protein that is isoelectric focusing is an electrophoretic separation based on charge. 18 During isoelectric focusing the separation of proteins is based solely on their different charge, more precisely their isoelectric point. A polyacrylamide gel is prepared in which there is a stable pH gradient. Electrophoresis of native proteins is carried out in the pH gradient in the absence of SDS. If electrophoresis is carried out in a medium that has a pH gradient, the macromolecules migrate until they reach their isoelectric point, i.e., where their net charge becomes zero. If the negative electrode is at the high pH part of the gel, the proteins will move toward the pH range equal to their isoelectric point. At their isoelectric point they will stop moving, since they do not have charge. When the molecule leaves the isoelectric point by diffusion, it gains a charge and electrophoresis drives it back. As a consequence macromolecules with different isoelectric points are focused in well separated lines. This highly sensitive technique is widely used for both analytical and preparative purposes. 19 The resolution limit of conventional, one-dimensional electrophoretic methods is limited since optimally 80 to 100 proteins can be separated. The resolution of two-dimensional polyacrylamide gel electrophoresis is much better. In this method isoelectric focusing in the first dimension is followed by SDS-PAGE in the second one. That is, proteins are separated by charge in first dimension and then by size in the second dimension. At the end of the analysis proteins appear as spots on the image of the gel after visualization (staining, autoradiography). Specific proteins can be visualized by immunological methods. 20 A novel technique was developed for the identification of nucleic acids by Edward Southern in 1975. He combined the electrophoresis, nucleic acid hybridization, and isotope labelling. According to this, firstly purified DNA from a biological sample (such as blood or tissue) is digested with a restriction enzyme(s), and the resulting DNA fragments are separated by gel electrophoresis. Then the DNA fragments are transferred out of the gel onto a sheet of cellulose-acetate paper („blotting”), and then the DNA fragments are identified by their radioactive labelling. In a similar fashion, RNA samples and proteins labelled with specific antibodies can also be identified. These techniques are called as Northern and Western blotting, respectively. 21 A mass spectrometer is an instrument capable of determining the mass of ions in a gas phase with high sensitivity and accuracy. The aim of mass spectrometry is to determine the constituents of a molecule, e.g., what kind of amino acids are present in a protein. The method is capable of studying macromolecules with a size of 300 kDa with picomolar accuracy, molecules smaller than 0.5 kDa can be analyzed in the attomolar range. Therefore, the importance of mass spectrometry for both basic research and medical diagnostics continues to grow. Moreover, mass spectrometers can be combined with further, widely used separation techniques, such as HPLC (High Pressure Liquid Chromatography or GC (Gas Chromatography) allowing the analysis of complex systems and mixtures. Each mass spectrometer consists of three essential parts: an ion source that transfers the molecules of the sample being tested into gas phase and ionizes them, an analyzer that accelerates the ions and separates them by an electric or magnetic field according to their m/z ratio, and a detector that collects the ions. The importance of detectors is negligible compared to that of the ion source or the analyzer. The ions are detected by a scintillation counter or an electron multiplier. 22 For biological macromolecules there are two effective ways to produce gas phase ions: Electro Spray Ionization (ESI) and Matrix Assisted Laser Desorption/Ionization (MALDI). In mass spectrometers, a vacuum must be created so that the accelerated ions do not collide with the atoms of the gas on their way to the detector. In the electrospray (ESI) method, the sample is injected into this vacuum through a capillary and vaporized into small droplets. The droplets become charged, since there is a 2-4 kV electric potential difference between the capillary and the wall of the vacuum chamber. The solvent evaporates quickly and as the droplets become smaller the charge density increases. The repulsive electrostatic force eventually breaks the drops into even smaller droplets. Finally, only the charged and gas phased macromolecules remain. MALDI uses a short laser pulse to vaporize the sample into the gas phase. The sample to be examined is mixed with a matrix having small molecular weight (e.g., Salicylic acid or 2,5-Dihydroxybenzoic acid etc.) that highly absorbs the laser light used. The laser pulse ionizes the matrix first and then the ionized matrix molecules ionize the sample. The ions of the macromolecules remain after the entire matrix has been evaporated. 23 The analyzer separates the ions, that are first accelerated by an electric field, according to their mass to charge (m/q) ratio. The most common analyzers are magnetic devices. Their function is based on the fact that magnetic force causes a charged particle to move on a circular path if the magnetic field is perpendicular to the direction of the velocity of the ion. Thus, the magnetic force supplies centripetal force and consequently causes centripetal acceleration, which changes only the direction of v and not its magnitude. Because magnetic force produces the centripetal acceleration according to Newton’s second law, we can equate its magnitude, qvB in this case, to the mass of the particle multiplied by the centripetal acceleration, that is v2/r. Then, after rearranging the equation we can express the mass to charge ratio as m/q=rB/v. This equation predicts that the radius of the path is proportional to the momentum mv of the particle and is inversely proportional to the charge and the magnetic field. Thus, the position of particles is determined by the m/q ratio. Furthermore, the m/q ratio can also be expressed as a function of the accelerating voltage (U) and accordingly the kinetic energy of the ion. At a given B or U value, ions of different m/q values travel on circular paths of different radii. Ions with higher mass move on a circular path having a larger radius. On the contrary, by changing the magnetic field the ions with different kinetic energy can move on a circular path of the same radius, thus the ions can be detected at the same location, but at different times. 24 The time of flight method requires short ion pulses so it is used with MALDI ionization. The basis of the TOF method is that ions with a larger m/q ratio will reach a lower speed if accelerated with the same voltage. The velocity of the ions is determined by measuring the time it takes them to travel a certain distance (L) in the absence of an electric or magnetic L m field. The time of fligh (t) can be calculated as follows: t L from which the m/q v 2qU ratio can be determined. 25 26