Week 1 - Introduction to Inherited Neurological Disorders (HMG 44110A) Course Outline PDF
Document Details
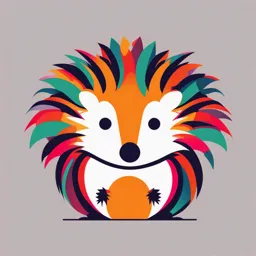
Uploaded by GrandAphorism
Abu Dhabi University
Dr. Merin Thomas
Tags
Related
Summary
This document is an introduction to a course on inherited neurological disorders at Abu Dhabi University. It details neurotransmitter function, neural circuits, neuroplasticity, neurogenesis, and the role of genetics in neurological disorders. The document also includes contact information and course guidelines.
Full Transcript
Week 1 Introduction to the Course: Inherited Neurological Disorders (HMG 44110A ) Review of neurotransmitters, Neural circuits, Neuroplasticity and Neurogenesis in the CNS. Role of genetics in neurological disorders Dr. Merin Thomas...
Week 1 Introduction to the Course: Inherited Neurological Disorders (HMG 44110A ) Review of neurotransmitters, Neural circuits, Neuroplasticity and Neurogenesis in the CNS. Role of genetics in neurological disorders Dr. Merin Thomas [email protected] Office hours : Monday & Wednesday, 3.00pm to 5.00pm; Tuesday & Thursday 1.00pm to 3.00pm 1 Contact details & Office Hours E-mail - [email protected] Office hours : Monday & Wednesday, 3.00pm to 5.00pm You are welcome to drop by for any clarifications outside office hours BUT it is advised to take prior appointment via Teams messenger Kindly avoid calling via Teams; meet me in person for all clarifications and queries - easier to answer doubts in person rather than via text/call. For instructions/queries related to assignments/exam portions etc. check blackboard before you reach out. 2 Rules for my classroom PUNCTUALITY & DISCIPLINE! Mode of Conducting Classes FACE TO FACE CLASSES Active participation from all students Each class - 1hour45min on Tuesdays & Thursdays Lecture notes will be uploaded in PDF format prior to lecture Attendance of utmost importance – Attendance is marked from day 1. Assessments will be written and on paper Deadlines Modes of Assessment QUIZZES ASSIGNMENT 2 quizzes will be given based on 800-word essay on given topic topics covered Topic, rubrics, guidelines will be 10-15min/Quiz ; 1 before midterm 1 discussed in week 5 after midterm Responses to be submitted week 9 FINAL PROJECT Group presentation on an Inherited MID TERM & FINAL EXAM neurological disorder of choice (not Midterm Exam - Week 6 covered in lectures) Final Exam - end of semester Rubrics, specific guidelines will be discussed in week 6/7 Presentation in week 12 & 13 Assessment Tool Weight Description Quiz 15% TWO quizzes 10-15 minutes each will be distributed across the semester, i.e. 1 quiz before the Midterm Exam and 1 quiz after the Midterm Exam. 7.5% weightage for each quiz Midterm Exam 20% Midterm exam will examine knowledge and understanding of the concepts and issues discussed in weeks 1-6 Assignment 15% Students will prepare an individual essay on a given course-relevant topic. Specific guidelines to be disseminated and discussed. Final Project 20% Student will submit an abstract and present, as a group, on an Presentation Inherited neurological disorder of choice. Specific guidelines to be disseminated and discussed Final exam 30% Final exam will examine knowledge and understanding of the concepts and issues involved in syllabus covered after midterm exam. TYPES OF QUESTIONS FORMAT MARKS EXAMPLE True or False 1 mark 1. Symptoms of Alzheimer’s may not become noticeable until after changes in the brain occur a. True. b. False Multiple Choice 1 mark 1. What is often the first symptom of Parkinson disease? Questions A. Headache B. Nausea C. Shaking of a hand or foot D. Turning of the head Mix and Match 4 marks 1. Match type of neurotransmitter to example i. Monoamines a. Oxytocin ii. Amino acids b. Acetylcholine iii. Peptides c. Histamine iv. Other. d. GABA Short answers/ 2 /3/4 1. Name two motor features and two non-motor features Case studies marks Parkinson’s disease? Two motor features are tremors and rigidity. Two non-motor features are sleep disorders and urinary problems. TIMELINE OF ASSESSMENTS REFERENCE BOOKS Beart, P., Robinson, M., Rattray, M., & Maragakis, N. J. (2017). Neurodegenerative diseases: Pathology, Mechanisms, and Potential Therapeutic Targets. Springer. Wu, Z. (2017). Inherited neurological disorders: Diagnosis and Case Study. Springer. Greenstein, B., & Greenstein, A. (2011). Color Atlas of Neuroscience: Neuroanatomy and Neurophysiology. Thieme. Learning Objectives Neurotransmitters Neural Circuits Neuroplasticity and neurogenesis in the CNS. Role of genetics in neurological disorders What are Neurotransmitters? Neurotransmitters are substances/chemical messengers that allow nerve cells (neurons) to communicate with each other and with their target tissues in the process of synaptic transmission (neurotransmission). A neurotransmitter signal travels from a neuron, across the synapse, to the next neuron/ nerve, muscle or gland cell What are Neurotransmitters? Neurotransmitters play a role in nearly every function in the body. Neurotransmitters are important in boosting and balancing signals in the brain and for keeping the brain functioning. Without neurotransmitters, the body can’t function. Too high or too low levels of specific neurotransmitters results in specific health problems. Medications work by increasing or decreasing the amount of or the action of neurotransmitters. What body functions do neurotransmitters help to control? Neurotransmitters help manage automatic responses such as breathing and heart rate, as well as they have psychological functions such as learning, managing mood, fear, pleasure, and happiness. What body functions do neurotransmitters help to control? Heartbeat and blood pressure. Breathing. Muscle movements. Thoughts, memory, learning and feelings. Sleep, healing and aging. Stress response. Hormone regulation. Digestion, sense of hunger and thirst. Feel sensations (response to sight, hearing, feel, touch and taste). Respond to all information the body receives from other internal parts of the body and the environment. How do neurotransmitters work (neurotransmission) ? – A look at the neuron How do neurotransmitters work (neurotransmission) ? – A look at the neuron Cell body. The cell body is vital to producing neurotransmitters and maintaining the function of the nerve cell. Dendrite. Branched cytoplasmic projections of the cell body that receive signals from the axons of other neurons. Axon. The axon carries the electrical signals along the nerve cell to the axon terminal. Axon terminal. This is where the electrical message is changed to a chemical signal using neurotransmitters to communicate with the next group of nerve cells, muscle cells or organs. ELECTRICAL SIGNALS IN A NEURON A synapse is the functional junction between one neuron and another, or between a neuron and an effector such as a muscle or a gland. Signal transmission at Synapses Presynaptic neuron refers to a nerve cell that carries a nerve impulse toward a synapse. It is the cell that sends a signal. Postsynaptic cell is the cell that receives a signal. A nerve cell is called a postsynaptic neuron when it carries a nerve impulse away from a synapse OR An effector cell that responds to the impulse at the synapse Fig 12.22 ,Examples of Synapses Principles of Anatomy and Physiology , 16th edition, authored by Gerard J. Tortora, Bryan H. Derrickson ; Wiley publications, Nov 2020 How do neurotransmitters work (neurotransmission) ? Signal transmission at Synapse - Chemical Synapse The plasma membranes of presynaptic and postsynapic neurons in a chemical synapse are close but they do not touch - separated by the synaptic cleft, a space that is filled with interstitial fluid. Nerve impulses cannot conduct across the synaptic cleft, so an alternative, indirect form of communication occurs. In response to a nerve impulse, the presynaptic neuron releases a neurotransmitter that diffuses through the fluid in the synaptic cleft and binds to receptors in the plasma membrane of the postsynaptic neuron. The postsynaptic neuron receives the chemical signal and in turn produces a postsynaptic potential, a type of graded potential. Neurotransmitters are stored within thin-walled sacs called synaptic vesicles. Each vesicle can contain thousands of neurotransmitter molecules. What happens to neurotransmitters after they deliver their message? After neurotransmitters deliver their message, the molecules must be clearedfromthesynapticcleft. Theydo thisin oneof threeways: Diffusion Reuptake (Reabsorbed and reused by the nerve cell that released it) Degradation (broken down by enzymes within the synapse) Released Binding Deactivate Nerve impulse Neurotransmitter Neurotransmitter reaches pre- bind to receptors is cleared from synaptic button in post-synaptic the synaptic cleft. membrane. Ca channels open Diffusion Ion channels Increased Ca ions Reuptake open- inflow of fuse to pre- synaptic Na and K ions. Degradation membrane Depolarization & release of formation of neurotransmitters action potential. into synaptic cleft. How many different types of neurotransmitters are there? Scientists haveidentified more than 100neurotransmitters and suspect thereare manyothersthat haveyetto bediscovered. They can be grouped into types based on their chemical nature and based on their size. CLASSIFICATION OF NEUROTRANSMITTERS BASED ON CHEMICAL NATURE WITH EXAMPLES Neurotransmitters Monoamines Amino acids Peptides Other Dopamine Glutamate Endorphins Acetylcholine Noradrenaline Adrenaline GABA Oxytocin Nitric oxide Histamine Purines Serotonin CLASSIFICATION OF NEUROTRANSMITTERS BASED ON SIZE WITH EXAMPLES Neurotransmitters SMALL MOLECULE NEUROPEPTIDES NEUROTRANSMITTERS SUBSTANCE P ACETYLCHOLINE HYPOTHALAMIC INHIBITING & AMINO ACIDS RELEASING HORMONES Glutamate, Aspartate, GABA BIOGENIC AMINES ENDORPHINS Norepinephrine, Epinephrine, Dopamine, Serotonin NITRIC OXIDE ANGIOTENSIN II CARBON MONOXIDE CHOLECYSTOKININ What action or change do neurotransmitters transmit to the target cell? The neurotransmitters bind to receptor proteins in the cellular membrane of the target tissue. The target tissue gets excited, inhibited, or functionally modified in some other way, depending onthespecificneurotransmitter. What action or change do neurotransmitters transmit to the target cell? Excitatory Inhibitory Modulatory or These These neuromodulators neurotransmitters neurotransmitters have These neurotransmitters can affect a have an excitatory/ an inhibiting the large number of neurons at the stimulating effect neurons. Inhibitory same time, as well as being able to on the neurons. If a neurotransmitters block influence the effects of other neurotransmitters. These can neurotransmitter is or prevent the chemical activate multiple receptors as there excitatory, it will message from being is not just one receptor for each type increase the passed along any of neurotransmitter. likelihood that the farther. It decreases the neuron will fire neuron firing action Its action is dependent on the receptor it binds to on the post- action potential. potential/stimulation. synaptic neuron. Examples of neurotransmitters Excitatory Inhibitory neurotransmitters Neuromodulators neurotransmitters Glutamate (Glu) gamma-Aminobutyric Dopamine (DA) acid (GABA) Acetylcholine (ACh) Serotonin (5-HT) Serotonin (5-HT) Histamine Acetylcholine (ACh) Dopamine (DA) Dopamine (DA) Norepinephrine (NE) Histamine [noradrenaline (NAd)] Epinephrine (Epi) Norepinephrine (NE) [adrenaline (Ad)] Examples of neurotransmitters Excitatory Inhibitory neurotransmitters Neuromodulators neurotransmitters Glutamate (Glu) gamma-Aminobutyric acid Dopamine (DA) (GABA) Acetylcholine (ACh) Serotonin (5-HT) Serotonin (5-HT) Histamine Acetylcholine (ACh) Dopamine (DA) Dopamine (DA) Norepinephrine (NE) Histamine [noradrenaline (NAd)] Epinephrine (Epi) Norepinephrine (NE) [adrenaline (Ad)] Examples of neurotransmitters Inhibitory Excitatory neurotransmitters neurotransmitters Neuromodulators Glutamate (Glu) gamma-Aminobutyric Dopamine (DA) acid (GABA) Acetylcholine (ACh) Serotonin (5-HT) Serotonin (5-HT) Histamine Acetylcholine (ACh) Dopamine (DA) Dopamine (DA) Norepinephrine (NE) Histamine [noradrenaline (NAd)] Epinephrine (Epi) Norepinephrine (NE) [adrenaline (Ad)] Examples of neurotransmitters Excitatory Inhibitory neurotransmitters Neuromodulators neurotransmitters Glutamate (Glu) gamma-Aminobutyric Dopamine (DA) acid (GABA) Acetylcholine (ACh) Serotonin (5-HT) Serotonin (5-HT) Histamine Acetylcholine (ACh) Dopamine (DA) Dopamine (DA) Norepinephrine (NE) Histamine [noradrenaline (NAd)] Epinephrine (Epi) Norepinephrine (NE) [adrenaline (Ad)] Examples of neurotransmitters Inhibitory Excitatory neurotransmitters neurotransmitters Neuromodulators Glutamate (Glu) gamma-Aminobutyric Dopamine (DA) acid (GABA) Acetylcholine (ACh) Serotonin (5-HT) Serotonin (5-HT) Histamine Acetylcholine (ACh) Dopamine (DA) Dopamine (DA) Norepinephrine (NE) Histamine [noradrenaline (NAd)] Epinephrine (Epi) Norepinephrine (NE) [adrenaline (Ad)] Why would a neurotransmitter not work as it should? Too much or not enough of one or more neurotransmitters are produced orreleased. Thereceptoron thereceivercell (thenerve,muscleor gland)isdefective. The cell receptors are not taking up enough neurotransmitter due to inflammation and damageofthesynapticcleft. Neurotransmittersarereabsorbed too quickly. Enzymes limit the number of neurotransmitters from reaching their targetcell. Existingdiseasesormedicationsmayaffect neurotransmitters. Diseases associated with neurotransmitters Whenneurotransmittersdo not functionwell,diseasecan occur. Acetylcholine Serotonin Dopamine Glutamate GABA High - Epiliptic Alzheimer Autism Schizophrenia Huntington seizures Low - Parkinsonism How do medications affect the action of neurotransmitters? Medications can block the enzyme that breaks down a neurotransmittersothat moreofit reachesnervereceptors. Example: Donepezil and galantamine block the enzyme acetylcholinesterase, which breaks down the neurotransmitter acetylcholine. These medications are used to stabilize and improve memory and cognitivefunction in people withAlzheimer’s disease. How do medications affect the action of neurotransmitters? Medications can block the neurotransmitter from being received at its receptorsite. Example: Selective serotonin reuptake inhibitors are a type of drug class that blocks serotonin from being received and absorbed by a nervecell. Thesedrugs may be helpful in treating depression, anxiety and other mental healthconditions. Medications can block the release of a neurotransmitter from a nerve cell. Example:Lithium blocking norepinephrine release.Thisdrugused in thetreatmentofbipolar disorder. Modifying the Effects of Neurotransmitters Substances naturally present in the body as well as drugs and toxins can modify the effects of neurotransmitters in several ways: Neurotransmitter synthesis can be stimulated or inhibited. For instance, many patients with Parkinson’s disease receive benefit from the drug L-dopa because it is a precursor of dopamine. For a limited period of time, taking L-dopa boosts dopamine production in affected brain areas. Neurotransmitter release can be enhanced or blocked. Amphetamines promote release of dopamine and norepinephrine. Botulinum toxin causes paralysis by blocking release of acetylcholine from somatic motor neurons. Modifying the Effects of Neurotransmitters The neurotransmitter receptors can be activated or blocked. An agent that binds to receptors and enhances or mimics the effect of a natural neurotransmitter is an agonist. Isoproterenol (Isuprel®) is a powerful agonist of epinephrine and norepinephrine. It can be used to dilate the airways during an asthma attack. An agent that binds to and blocks neurotransmitter receptors is an antagonist. Zyprexa®, a drug prescribed for schizophrenia, is an antagonist of serotonin and dopamine. Modifying the Effects of Neurotransmitters Neurotransmitter removal can be stimulated or inhibited. For example, cocaine produces euphoria—intensely pleasurable feelings—by blocking transporters for dopamine reuptake. This action allows dopamine to linger longer in synaptic clefts, producing excessive stimulation of certain brain regions. NEURAL CIRCUITS NEURAL CIRCUITS A circuit typically refers to a set of interconnected components that together subserve a specific function. A neural circuit in the brain may be a cluster of neurons that receives electrochemical information that the circuit modifies and transmits to other circuits for further modification. Alternatively, a neural circuit may comprise a network of interconnected brain regions that together integrate vast amounts of information and perform more complicated cognitive and regulatory functions NEURAL CIRCUITS The CNS contains billions of neurons organized into complicated networks called neural circuits, functional groups of neurons that process specific types of information. In a simple series circuit, a presynaptic neuron stimulates a single post- synaptic neuron. The second neuron then stimulates another, and so on Most neural circuits are more complex. NEURAL CIRCUITS A single presynaptic neuron may synapse with several postsynaptic neurons. Such an arrangement, called divergence, permits one presynaptic neuron to influence several postsynaptic neurons (or several muscle fibers or gland cells) at the same time. In a diverging circuit, the nerve impulse from a single presynaptic neuron causes the stimulation of increasing numbers of cells along the circuit NEURAL CIRCUITS – Diverging Circuit For example: a small number of neurons in the brain that govern a particular body movement stimulate a much larger number of neurons in the spinal cord. Sensory signals are also arranged in diverging circuits, allowing a sensory impulse to be relayed to several regions of the brain. This arrangement amplifies the signal. NEURAL CIRCUITS In another arrangement, called convergence, several pre-synaptic neurons synapse with a single postsynaptic neuron. This arrangement permits more effective stimulation or inhibition of the postsynaptic neuron. In a converging circuit the postsynaptic neuron receives nerve impulses from several different sources NEURAL CIRCUITS – Converging Circuit For example, a single motor neuron that synapses with skeletal muscle fibers at neuromuscular junctions receives input from several pathways that originate in different brain regions. NEURAL CIRCUITS Some circuits are organized so that stimulation of the pre-synaptic cell causes the postsynaptic cell to transmit a series of nerve impulses. One such circuit is called a reverberating circuit. In this pattern, the incoming impulse stimulates the first neuron, which stimulates the second, which stimulates the third, and so on. Branches from later neurons synapse with earlier ones. NEURAL CIRCUITS This arrangement sends impulses back through the circuit again and again. The output signal may last from a few seconds to many hours, depending on the number of synapses and the arrangement of neurons in the circuit. Inhibitory neurons may turn off a reverberating circuit after a period of time. NEURAL CIRCUITS – Reverberating Circuit Among the body responses thought to be the result of output signals from reverberating circuits are breathing, coordinated muscular activities, waking up, and short-term memory. NEURAL CIRCUITS A fourth type of circuit is the parallel after-discharge circuit. In this circuit, a single presynaptic cell stimulates a group of neurons, each of which synapses with a common postsynaptic cell. A differing number of synapses between the first and last neurons imposes varying synaptic delays, so that the last neuron exhibits multiple excitatory post-synaptic potentials (EPSPs) or inhibitory post-synaptic potentials (IPSPs) If the input is excitatory, the postsynaptic neuron then can send out a stream of impulses in quick succession. NEURAL CIRCUITS - parallel after-discharge circuit Parallel after-discharge circuits may be involved in precise activities such as mathematical calculations. NEURAL CIRCUITS Alternatively, a neural circuit may comprise a network of interconnected brain regions that together integrate vast amounts of information and perform more complicated cognitive and regulatory functions Neural circuits process sensory information, generate motor output, and create spontaneous activity NEURAL CIRCUITS Let's study with an example: The neural circuits responsible for the control of movement can be divided into four distinct, highly interactive subsystems 1. Local circuitry within the gray matter of the spinal cord and the comparable circuitry in the brainstem 2.Neurons whose cell bodies lie in the brainstem or cerebral cortex 3. The Cerebellum 4. Basal Ganglia: embedded in the depths of the forebrain The problems associated with disorders of basal ganglia e.g. Parkinson's disease and Huntington's disease, attest to the importance of this complex in the initiation of voluntary movements NEUROPLASTICITY The ability of the nervous system to change its activity in response to intrinsic or extrinsic stimuli by reorganizing its structure, functions, or connections. These changes can either be beneficial (restoration of function after injury), neutral (no change), or negative (can have pathological consequences). A change could be temporal(functional) or spatial(structural). A temporal change is further divided into short-term and long-term change and takes place at individual neurons. A spatial change takes place either at synapses, within neurons or within glial cells. NEUROPLASTICITY Neuroplasticity is also a phenomenon that aids brain recovery after the damage produced by events like stroke or traumatic injury. This ability to manipulate specific neuronal pathways and synapses has important implications for physiotherapeutic clinical interventions that will improve health. A better understanding of the mechanisms governing neuroplasticity after brain damage or nerve lesion will help improve the patient's quality of life NEUROGENESIS NEUROGENESIS Neurogenesis, the birth of new neurons from undifferentiated stem cells—occurs regularly in some animals. Until recently, the dogma in humans and other primates was “no new neurons” in the adult brain. Then, in 1992, researchers published their unexpected finding that the hormone like protein epidermal growth factor (EGF) stimulated cells taken from the brains of adult mice to proliferate into both neurons and astrocytes. NEUROGENESIS Previously, EGF was known to trigger mitosis in a variety of non neuronal cells and to promote wound healing and tissue regeneration. In 1998, scientists discovered that significant numbers of new neurons do arise in the adult human hippocampus, an area of the brain that is crucial for learning. NEUROGENESIS The nearly complete lack of neurogenesis in other regions of the brain and spinal cord seems to result from two factors: 1. Inhibitory influences from neuroglia, particularly oligodendrocytes 2. Absence of growth-stimulating cues that were present during fetal development. Axons in the CNS are myelinated by oligodendrocytes rather than Schwann cells, and this CNS myelin is one of the factors inhibiting regeneration of neurons. NEUROGENESIS Perhaps this same mechanism stops axonal growth once a target region has been reached during development. Also, after axonal damage, nearby astrocytes proliferate rapidly, forming a type of scar tissue that acts as a physical barrier to regeneration. Thus, injury of the brain or spinal cord usually is permanent. NEUROGENESIS Ongoing research seeks ways to improve the environment for existing spinal cord axons to bridge the injury gap. Scientists also are trying to find ways to stimulate dormant stem cells to replace neurons lost through damage or disease and to develop tissue-cultured neurons that can be used for transplantation purposes. Genetics and Neurological Disorders: The Connection Genetics and Neurological Disorders: The Connection Neurological disorders consist of a broad array of conditions that impact the brain, spinal cord, and nerves. Ranging from Alzheimer's disease and Parkinson's to epilepsy and multiple sclerosis, the variety is extensive. These conditions can result from a mix of genetic, environmental, and lifestyle elements. Some neurological disorders exhibit a recognized genetic inclination, stemming from gene mutations or a blend of genetic factors that heighten susceptibility. Primary genetic causes of neurological disorders SINGLE GENE MUTATIONS (MENDELIAN DISORDERS): Some neurological conditions are caused by mutations in a single gene. These disorders often follow Mendelian inheritance patterns, such as autosomal dominant, autosomal recessive, or X- linked. Examples include: Huntington’s disease (autosomal dominant mutation in the HTT gene) Friedreich’s ataxia (autosomal recessive mutation in the FXN gene) Duchenne muscular dystrophy (X-linked mutation in the DMD gene) Primary genetic causes of neurological disorders MULTIPLE GENE (POLYGENIC) INVOLVEMENT: Some disorders arise from the combined effects of mutations in multiple genes, often in conjunction with environmental factors. These are termed polygenic or complex disorders. Alzheimer’s disease, for instance, has several genetic risk factors, with the APOE ε4 allele being the most prominent. Primary genetic causes of neurological disorders COPY NUMBER VARIATIONS (CNVS): CNVs are alterations in the DNA that result in the cell having an abnormal number of copies of one or more sections of the DNA. CNVs can be associated with various neurological disorders, including some forms of epilepsy and autism spectrum disorders Primary genetic causes of neurological disorders MITOCHONDRIAL DNA MUTATIONS: The mitochondria have their own DNA, separate from the nuclear DNA. Mutations in mitochondrial DNA can lead to neurological disorders since the brain is highly dependent on the energy produced by mitochondria. Examples include Leber’s hereditary optic neuropathy and some forms of mitochondrial myopathy. Primary genetic causes of neurological disorders REPEAT EXPANSION DISORDERS: Some neurological disorders are caused by the abnormal repetition of short sequences of DNA. Over time, these repeats can expand, leading to disease. Examples include: Huntington’s disease (CAG repeat expansion) Fragile X syndrome (CGG repeat expansion) Spinocerebellar ataxias (various repeat expansions) Primary genetic causes of neurological disorders EPIGENETIC CHANGES: Epigenetics refers to changes in gene activity that don’t involve alterations to the underlying DNA sequence. These changes can affect gene expression and are implicated in some neurological conditions. Rett syndrome, for instance, involves mutations in the MECP2 gene, which plays a role in epigenetic regulation. Primary genetic causes of neurological disorders PRION DISEASES: These are caused by misfolded proteins called prions. While most prion diseases are sporadic, some, like familial Creutzfeldt- Jakob disease, have a genetic component. REFERENCES Tortora, Gerard J. And Bryan H Derrickson. Principles of Anatomy and Physiology. John Wiley & Sons, 2020 Beart, P., Robinson, M., Rattray, M., & Maragakis, N. J. (2017). Neurodegenerative diseases: Pathology, Mechanisms, and Potential Therapeutic Targets. Springer. Puderbaugh M, Emmady PD. Neuroplasticity. [Updated 2023 May 1]. In: StatPearls [Internet]. Treasure Island (FL): StatPearls Publishing; 2024 Jan-. Available from: https://www.ncbi.nlm.nih.gov/books/NBK557811/ https://www.physio-pedia.com/Neuroplasticity https://www.physiopedia.com/Neural_Circuit Tau, G. Z., & Peterson, B. S. (2009). Normal development of brain circuits. Neuropsychopharmacology, 35(1), 147–168. https://doi.org/10.1038/npp.2009.115