Wagih Ultrasound Imaging 1 PDF
Document Details
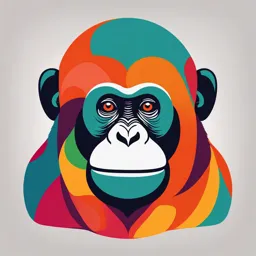
Uploaded by UndisputedThorium
Assiut University
Tags
Summary
This document provides an introduction to ultrasound imaging, covering its principles, instrumentation, and transducer design, including acoustic impedance and matching layers.
Full Transcript
Ultrasound Imaging Part one 4.1 Introduction Ultrasound is a mechanical wave, with frequencies between 1 and 30 MHz for clinical use. The speed of sound in tissue is 1540 m/s, and so the range of wavelengths of ultrasound in tissue is between 0.05 and 1.5 mm. The ultrasoun...
Ultrasound Imaging Part one 4.1 Introduction Ultrasound is a mechanical wave, with frequencies between 1 and 30 MHz for clinical use. The speed of sound in tissue is 1540 m/s, and so the range of wavelengths of ultrasound in tissue is between 0.05 and 1.5 mm. The ultrasound waves are produced by a transducer, as shown in Figure 4.1, which typically has an array of up to 512 individual active sources. In the simplest image acquisition scheme, small subgroups of these elements are fired sequentially to produce parallel ultrasound beams. As the ultrasound passes through tissue, a small fraction of the energy is reflected from the boundaries between tissues which have slightly different acoustic and physical properties: the remaining energy of the beam is transmitted through the boundary. The reflected waves are detected by the transducer and the distance to each tissue boundary is calculated from the time between As soon as the signal from the deepest tissue boundary has been detected from one beam, the next adjacent beam of ultrasound is emitted, and this process is repeated until the entire image has been acquired. Due to the relatively high speed of ultrasound through tissue, entire images can be acquired in fractions of a second, allowing real-time imaging to Ultrasound imaging can also be used to measure blood flow via the Doppler effect, in which the frequency of the received signal is slightly different than that of the transmitted signal due to blood flow either towards or away from the transducer. Figure 4.1 (left) Basic principle of ultrasound imaging. A transducer sends a series of pressure waves through the tissue. At boundaries between tissues, a small fraction of the energy is backscattered towards the transducer where it is detected. Using the speed of sound through tissue, the depth of the tissue boundary can be determined. Electronic steering of the beam across the sample builds up successive lines which form the image. (right) The intensity of each pixel in the image is proportional to the strength of the detected signal reflected from that point. Instrumentation 4.4 Instrumentation Figure 4.5 shows a block-diagram of the basic components of the ultrasound imaging system. The input signal to the transducer comes from a frequency generator. The frequency generator is gated on for short time durations and then gated off, thus producing short periodic voltage pulses. These pulsed voltage signals are amplified and fed via a transmit/receive switch to the transducer. The purpose of the transmit/receive switch is to completely isolate the transmit and receive circuits, which is essential to allow the transducer both to transmit high power pulses and also receive very low intensity signals. The amplified voltage is converted by the transducer into a mechanical pressure wave (ultrasound wave) which is transmitted into the body. Reflection and scattering from boundaries and structures within tissue then occur. 4.5 Single element ultrasound transducers The active element of all ultrasound transducers is shaped piezoelectric material. It is formed from a composite of lead zirconate titanate (PZT). In the case of a single element transducer, the element is usually disk- shaped or formed into a spherical or cylindrical shell. The two faces of the element are coated with a thin layer of silver and connected via bonding wires to a coaxial cable leading to the transmit-receive switch, as shown in Figure 4.6. Figure 4.6 (left) A transducer with a flat PZT element. (right) Flat and hemispherical PZT elements. A PZT-based transducer has a Z value of ~30 x 105 g cm-2 s- 1 , compared to the value of skin/tissue of ~1.7 x 105 g cm-2 s-1. Due to the large difference in the characteristic acoustic impedance between the transducer crystal and skin, a large amount of energy is reflected from the patient’s skin, and the efficiency of coupling the mechanical wave into the body would be very low. A ‘matching layer’ is therefore added to the external face of the crystal to provide acoustic coupling between the crystal and the patient, with the value of Z of this matching layer (Z matching layer) being intermediate between that of the transducer element (Z PZT) and the skin (Z skin). Transducer Design Matching layer(s) Only about 20% of the wave’s power would be transmitted through the front PZT–patient interface means there is also a potential problem of poor sensitivity. First, the matching layer should have a thickness equal to a quarter of a wavelength. Second, it should have an impedance equal to , where z PZT is the impedance of PZT and z T is the impedance of tissue. The End