UTZ-PRELIM_edited.pdf
Document Details
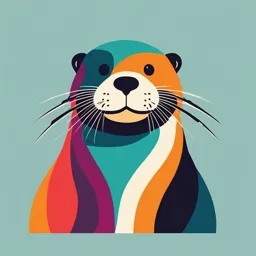
Uploaded by GracefulAlgorithm
Tags
Full Transcript
Canteras, C.f. LESSON 1 stones, he turned to ultrasound to detect the masses when...
Canteras, C.f. LESSON 1 stones, he turned to ultrasound to detect the masses when they were embedded in soft tissue. His analysis in using ULTRASONOGRAPHY (relevant terminologies) these soundwaves on animal tissues helped the next scientist PIEZOELECTRIC EFFECT down the line. the ability of certain materials to generate an electric charge It was Ian Donald who first fused ultrasound with diagnostic in response to applied mechanical stress. medicine in 1956. Naturally, his first use of the device was to VELOCITY determine the diameter of the head of a fetus. In only two the speed and the direction of motion of an object. years, technology helped create a way to visualize the FREQUENCY findings of an ultrasound, increasing sits life-saving diagnostic the number of waves that pass a fixed point in unit time; also, potential. the number of cycles or vibrations undergone during one unit How Ultrasound Works of time by a body in periodic motion. The device held close to the body is known as a WAVELENGTH transducer. The transducer contains special crystals that can distance from the "crest" (top) of one wave to the crest of the create soundwaves when they are activated with an electrical next wave. field. Not only that, but they can also receive soundwaves VELOCITY reflected by our body’s tissue. These tissues have different measures the number of wave cycles (or frequency) passing densities, which reflect varying soundwaves. through a given point in a second. The reflected soundwaves are calculated by a SOUNDWAVE computer and interpreted by a sonogram. By measuring how pattern of disturbance caused by the movement of energy quickly a soundwave is reflected to the transducer, the traveling through a medium as it propagates away from the machine can generate an image based on the data. This is source of the sound. shown on the screen attached to the sensor. ATTENUATION The GEL makes sure there are no air pockets refers to any reduction in the strength of a signal. between your skin and the transducer. Such air pockets could ACOUSTIC IMPEDANCE block the soundwaves and prevent the ultrasound from resistance to the propagation of ultrasound waves through imaging correctly. tissues. Ultrasonographic Biological Effects ACOUSTIC MISMATCH used to describe the reflection and transmission of acoustic Ultrasound produces biological effects by two tissue waves at interfaces of two materials with mismatched interactions: heating and cavitation. Heating is caused by the impedances. mechanical friction of the tissue moving during passing of the SOUND INTENSITY NOTATION ultrasonic wave. Cavitation is the production and collapse of the subjective perception of sound pressure. small bubbles in the inter- and intracellular tissue fluid. History The use of ultrasound in medicine began during and shortly after the 2nd World War in various centers around the world. The work of Dr. Karl Theodore Dussik in Austria in 1942 on transmission ultrasound investigation of the brain provides the first published work on medical ultrasonics. Although other workers in the USA, Japan and Europe have also been cited as pioneers, the work of Professor Ian Donald PHYSICAL UNITS OF MEASURE and his colleagues in Glasgow, in the mid-1950s, did much to facilitate the development of practical technology and UNITS OF LENGTH applications. This led to the wider use of ultrasound in KILOMETER (KM) | METER (M) | CENTIMETER (CM) medical practice in the subsequent decades. *Circumference is the perimeter of a circle and is also expressed as a Using soundwaves to determine the position of objects first unit of length (mm, cm, or m). cm2 is the unit of area. Circumference appeared in the 1794 work by Lazaro Spallanzani, an Italian is simply a distance. physicist. He studied how bats managed to fly in total UNITS OF AREA darkness and theorized that the nocturnal creatures were SQUARE METER (M2) | SQUARE CENTIMETER (CM2) dependent on using sound to navigate. UNITS OF VOLUME It wasn’t until 1880, almost ninety years after Spallanzani’s CUBIC METER (M3) | SQUARE MILLIMETER (MM3) theory was published that the first machine that could UNITS OF TIME generate soundwaves was made. Renowned French SECONDS (S) | MILLISECONDS (MS) scientists, Jacques and Pierre Curie determined that by UNIT OF POWER applying electrical currents to quartz crystal they produce WATTS (W) sound, more specifically, ultrasonic waves. UNITS OF SPEED Sy Sokolov, a Russian physicist, was the first to conceptualize METER PER SECONDS (M/S) | MILLIMETERS PER using ultrasound for imaging techniques in 1928. However, MICROSECOND he was more interested in using the method to find UNIT OF WORK imperfections in metallic structures than for saving lives JOULE (J) through diagnostics. However, the device he invented did UNIT OF ACOUSTIC IMPEDANCE create shadow images that could be interpreted. RAYLS An American scientist, George Ludwig was one of the first to use ultrasound imaging techniques. In his research about gall Canteras, C.f. SONOGRAPHY RAREFACTIONS ✓ Comes from Latin sonus (sound) and the Greek Regions of LOW pressure and density graphien (to write) COMPRESSIONS ✓ Medical imaging that uses non-ionizing, high Regions of HIGH pressure and density frequency soundwaves to generate image of a WAVE ANATOMY particular structure. WAVELENGTH (λ lambda) OTHER TERMS: distance between two (2) consecutive identical positions in Diagnostic Ultrasound the wav. Diagnostic Medical Sonography Unit: mm History of Ultrasound FREQUENCY the number of cycles per second performed by the particles in the medium in response to wave passing through it. A patient being scanned in a Unit: Hertz (Hz) water bath EARLY 1950’s. FREQUENCY RANGE INFRA LATE 1950’s Is from the Latin word meaning “below”. The first contact ULTRA compound B- Is from the Latin word scanner which meaning “beyond”. uses olive oil as a lubricant was developed. RATIONALE HIGHER FREQUENCY are ABSORBED MORE rapidly than Single crystal lower frequencies. transducer mounted on an articular arm. LOW FREQUENCIES have BETTER PENETRATION. HIGHER FREQUENCIES have SHORTER WAVELENGTH. Early ultrasound equipment visual Shorter wavelengths can distinguish between reflectors that displays used oscilloscopes which are closer together. Therefore, higher frequencies have better produced bi-stable (black and white) spatial resolution but limited penetration. images. POINTERS TO PONDER 1970 As the frequency of the ultrasound INCREASES: Gray scale imaging was introduced. 1. The ability to resolve small objects improve. 2. The penetrability of the beam decreases. 3. The beam becomes more collimated and directional. MID 1970'S Real time scanning systems were introduced. MID 1980'S T - PERIOD Application of doppler the time it takes for one cycle to occur. techniques were introduced. Ultrasound common unit: microsecond Note: Period and frequency is inversely proportional. PHYSICAL PRINCIPLES OF ULTRASOUND 𝟏 𝒑𝒆𝒓𝒊𝒐𝒅 = 𝒇𝒓𝒆𝒒𝒖𝒆𝒏𝒄𝒚 WAVE period DECREASES as frequency INCREASES. is a traveling variation in or more quantities such as pressure. Waves are usually produced by something moving back and forth or vibrating. TWO CATEGORIES OF WAVE PHENOMENA 1. Mechanical Wave 2. Electromagnetic Wave MECHANICAL WAVE Can be transmitted and produced by matter in any form; solid, liquid, or gas. Can travel only through matter. PHASE ELECTROMAGNETIC WAVE refers to the relationship of one wave to another. Can travel either through matter or through empty space. Ultrasound waves whose wavefronts are at the SAME WAVES ARE DIVIDED INTO TWO BASIC TYPES position, are said to be in phase. LONGITUDINAL Ultrasound waves whose wavefronts are OUT of Motion of particles in a medium is PARALLEL to the direction position, one to the other, are said to be out of of wave propagation. phase. TRANSVERSE Sound waves demonstrate interference phenomena or the Motion of the particles in a medium is PERPENDICULAR to superposition of waves (algebraic summation). the direction of wave propagation. INTERFERENCE SOUND IS A TRAVELING VARIATION OF The interaction of two or more ultrasound beams having PRESSURE AND DENSITY different frequency and/or phase. Canteras, C.f. A. CONSTRUCTIVE INTERFERENCE SOUND PROPAGATION MEDIA PROPERTIES Occurs when ultrasound waves of the same frequency are in Elasticity Density phase, resulting in increased amplitude. It increases the Refers to the ability of an Mass per unit volume. intensity of the ultrasound beam. object to return to its if all other physical B. DESTRUCTIVE INTERFERENCE original shape and volume properties are maintained Occurs when ultrasound waves of the same frequency are out after a force is no longer unchanged, then an of phase, resulting in decreased amplitude. This interference acting on it. increase in density will contributes to ultrasound attenuation. impede the rate of sound WAVE CHARACTERISTICS propagation through the ACOUSTIC VARIABLE medium. Acoustic variables are measurable quantities that vary within velocity of sound in a a medium as sound propagates through the medium medium is inversely (acoustic being derived from Greek word for hearing.) proportional to the square PRESSURE root of the density of the DENSITY medium. PARTICLE MOTION TEMPERATURE Compressibility (K) Bulk Modulus PRESSURE DENSITY Indicates the fractional Reciprocal of Amount of force in a given Concentration of matter decrease in volume when compressibility. area. (mass per unit volume) pressure is applied to the As the bulk modulus Unit: Pascal (Pa) Unit: kg/m3 or g/cm3 material. increases and the Other units: pounds per The easier a medium is to compressibility decreases, square inch (lbs/sq. in) and reduce in volume, the the velocity of sound in the (N/m2) higher is its compressibility. medium increases. The velocity of sound Some authorities refer to TEMPERATURE PARTICLE MOTION through a medium is also this property as the Concentration of heat distance moved by the inversely proportional to "stiffness" of the medium. energy. molecules in the medium. the square root of the Unit: degrees Fahrenheit, Unit: unit for distance, compressibility of the Celsius, or Kelvin scale typically nanometer medium. STIFFNESS AMPLITUDE Resistance of a material to compression. It is the maximum variation that occurs in an acoustic variable. inverse of compressibility. measure of how far variable gets away from its normal, "Hardness" undisturbed value. Stiffer media have higher sound speeds. Thus, POWER propagation speeds are lower in gases. The rate at which work is done or the rate of flow of energy through a given area. Unit is Watt. INTENSITY Power per unit area. Unit is W/cm2. 𝒑𝒐𝒘𝒆𝒓 𝒊𝒏𝒕𝒆𝒏𝒔𝒊𝒕𝒚 = 𝒂𝒓𝒆𝒂 Increasing power, increases intensity. Decreases area increases intensity because power is more concentrated. Increasing area, decreasing intensity because power is less concentrated. Ultrasound intensity is proportional to the amplitude square. PROPAGATION SPEED C = λF Speed at which sound moves through a medium. Equals to the product of wavelength and frequency. Units: Meters per second (m/s) or millimeters per second It is a tradeoff between stiffness and density. (mm/μs) The stiffer the medium, the higher the propagation SOUND PROPAGATION PROPERTIES speed. PROPAGATION SPEED The denser the medium, the lower the propagation Elasticity speed. Density Solids propagate sound faster than liquids or gases Bulk Modulus because while they are denser (which would slow Compressibility down the speed) they are much stiffer. Canteras, C.f. ACOUSTIC IMPEDANCE (Z) ↓ F = ↑ P = ↑ W inversely proportional Property of a substance, which describes how particles of that In Ultrasound: substance behave when subjected to a soundwave. ✓ Air = can give artifacts that can diagnosed as Z = PC pathologies. Where: ✓ The lower the frequency, the better the image in P = density UTZ, because we capture soft tissues. The denser, C = speed the better. Z = acoustic impedance ✓ 99% heat, 1% light. ✓ Uses mechanical energy. ✓ Acoustic Impedance = uses echoes to create sonographic In substance with the same Z, image. Bouncing back of sounds. there is total transmission of ✓ Sonographer = professionals who perform ultrasound ✓ Sonography = the study of ultrasound energy and no reflections. ✓ Sonographic Image = the result of the procedure ✓ MU = μ ✓ frequency = cycle/seconds LESSON 2 ULTRASONOGRAPHY In substance with small difference ULTRASOUND INTERACTION AND ATTENUATION in acoustic impedance, a small ACOUSTIC BOUNDARIES amount of energy is reflected but Also known as TISSUE INTERFACE majority is transmitted. Position within the tissue where the values of acoustic impedance change. ATTENUATION Weakening of the sound as it propagates through a In substance with small medium. difference in acoustic Reduction in amplitude and intensity as sound impedance, there is a large travels. amount reflected and small Unit of attenuation is DECIBEL. amount of transmitted. MEDIUM 1 INTENSITY REFLECTION COEFFICIENT (R) MEDIUM 2 Gives the proportion of energy reflected from an interface between two substances. MEDIUM 3 (𝑍2 − 𝑍1 )2 FACTORS AFFECTING ATTENUATION 𝑅= (𝑍2 + 𝑍1 )2 1. Medium INTENSITY TRANSMISSION COEFFICIENT (ITC) 2. Frequency Fraction of the incident intensity transmitted into the second ✓ Where the molecules of the tissue are densely medium. 4𝑍1 𝑍2 packed, attenuation will be much greater than in less 𝐼𝑇𝐶 = (𝑍 2 densely packed tissue. 1 + 𝑍2 ) ACOUSTIC IMPEDANCE MISMATCH ✓ If path length increases, attenuation increases. Difference in acoustic impedance between two substances. NOTE! NOTE! ✓ Attenuation will not only occur in the beam of sound ✓ FOR MOST SOFT TISSUE INTERFACES, PERCENTAGE produced by the transducer as it produces OF ENERGY REFLECTED IS 1% OR LESS propagates through tissue, but also in returning 1. WHY CAN'T ULTRASOUND GO THROUGH AIR? echoes as they travel back to the transducer. ✓ Because almost all sound is reflected at an air-water ✓ Higher frequencies are more attenuated than lower interface. frequencies. Lower frequencies penetrate deeper 2. WHY CAN'T UTZ GO THROUGH BONE? than higher frequencies because they are attenuated ✓ It does but because of impedance mismatch to a lesser degree. between bone and soft tissue, causing most of the ATTENUATION COEFFICIENT sound to be reflected at the interface. Attenuation that occurs with each centimeter the sound wave travels. Unit is dB/cm. If the attenuation coefficient increases, attenuation increases. For soft tissues, the typical value for attenuation coefficient is 0.5 dB/cm. o Soft Tissue – term used to describe the average tissue that makes up the soft tissues of the human body (e.g., liver, kidney, spleen). Canteras, C.f. ATTENUATION Progressive weakening of the sound beam. Terms to describe when the beam is Absorption – converted to heat. perpendicular to the interface. Reflection – back to the transducer. Scattering – reflected in multiple directions. Refraction – re-direction of part of the sound beam. Divergence – power spread over a large area. ABSORPTION Process by which energy in the ultrasound beam is transferred to the propagating medium, where it is Denotes a direction of travel of the transformed into a different form of energy, mostly incident ultrasound that is not heat. The medium is said to absorb energy from the perpendicular to the boundary beam. between two media. The dominant factor contributing to attenuation of ultrasound in soft tissues. The rate of absorption is directly proportional to the SCATTERING frequency. Higher frequency results in increased Occurs when an ultrasound wave strikes a boundary ultrasound absorption. or interface between 2 small structures and the REFLECTION wave is scattered in different directions. Occurs when two large structures of significantly Is responsible for providing the internal texture of different acoustic impedance form an interface, the organs in the image. interface becomes a reflector and some of the wave RAYLEIGH SCATTERING energy is reflected back to the transducer. The When the scatter in equal in all direction energy remaining in the wave is decreased. REFRACTION The major interaction of interest for diagnostic The change in direction of a sound beam as it enters ultrasound. the medium. o INCIDENT ENERGY - the sound that hits an Transmission with a bend. acoustic interface. o If the angle of incidence is 90 degrees, no o ECHO - reflected beam. refraction will occur. The physics of As we have seen, the percentage of incident energy that is refraction are described by SNELL'S LAW. reflected depends upon the acoustic impedance mismatch. SNELL'S LAW REFLECTION Formula which gives the relationship between the angle of The values of Z for the soft tissues are quite similar incidence and the angle of refraction when a beam of sound to one another. passes through an interface between two tissues where the o We conclude that reflections at boundaries speed of sound is different. between soft tissue will give rise generally Where: small echoes. θi - angle of incidence The value of Z for air (and other gaseous material) is θt - transmitted angle much lower than the soft tissue. Ci - velocity of sound in the o We conclude that reflection from gas/soft incident medium tissue interface gives rise to a very large Ct - velocity of sound in the echo. transmitted medium The Z value for bone is several times higher than the REFRACTION soft tissue average. o Therefore, a reflection from a soft tissue/bone interface produces a large echo. ANOTHER FACTOR THAT AFFECTS THE STRENGTH OF THE REFLECTION IS THE SIZE OF THE REFLECTOR Reflectors can either be SPECULAR or DIFFUSE. If propagation speed 2 is less than propagation 1. SPECULAR REFLECTOR speed 1, then the transmission angle is less than the Occurs when the boundary is smooth and larger incident angle. than the beam. If propagation speed 2 is greater than propagation Angle of incidence = angle of reflection speed 1, the transmission angle is greater than the 2. DIFFUSE REFLECTOR incident angle. Occurs when the reflecting interface is irregular REMEMBER! in shape and its dimensions are smaller than The two requirements for REFRACTION to occur are: the diameter of the ultrasound beam. 1. Oblique incidence Incident beam is reflected in many different 2. Different propagation speed on either side of the directions. boundary. SPECULAR REFLECTOR DIVERGENCE The angle of incidence of ultrasound beam will always equal As a beam of ultrasound travels through it will the angle of reflection. diverge. This divergence will result in the same Canteras, C.f. power spread over larger area. The intensity of the PIEZOELECTRICITY beam will therefore be reduced. Derived from the Greek term piezo (to press) The rate of divergence (and rate of intensity and elektron (amber, which is an organic plant reduction) depends on the diameter of sound resin that was used in early electrical study). source (more pronounced as the source is made Was described 1880 by Pierre and Jacques Curies. smaller). States that some materials produced a voltage when LESSON 3 deformed by an applied pressure. Conversely, TRANSDUCER piezoelectricity also results in the production of a Convert one form of energy to another. pressure when an applied voltage deforms these ELECTRICAL ENERGY > ULTRASOUND ENERGY materials. BACKING/ DAMPING MATERIAL Eliminate the vibrations from the back face and to control the length of vibrations from the face of the crystals. Consists of tungsten powder and plastic or epoxy resin. Attached to the back face of the crystal. MATCHING LAYER GENERAL COMPOSITION OF AN ULTRASOUND TRANSDUCER: Sandwiched between the piezoelectric crystal and 1. Physical housing the patient. 2. Electrodes Has acoustic impedance value halfway between 3. Piezoelectric elements that of the crystal and soft tissue. This results in 4. Backing material more transmitted energy entering the patient and 5. Impedance matching improves the signal strength of returning echoes, layer which in turn improves UTZ system's sensitivity and resolution. PHYSICAL HOUSING ULTRASOUND BEAM Contains all the Area through which the sound energy emitted from individual components including the crystals, the transducer travels. electrodes, matching As sound travels, the width of the beam changes later, and backing starts out as exactly the same size as the transducer material. diameter and gets narrower until it reaches smallest Provides structural diameter and then diverges. support and acts as an SUBDIVIDED INTO 2 REGIONS: electrical and acoustic insulator. A. Near field (Fresnel Zone) ELECTRODES Region nearest the transducer face, characterized by a highly a. Outside Electrode collimated beam with more uniform intensity. o "Grounded electrode" B. Far Field (Fraunhofer Zone) o Protects patients from electric shock. Region farthest from the transducer and characterized by the b. Inside Electrode divergence of the beam with great variation in intensity. o "Live Electrode" o Abuts against a thick backing block. FOCUS OR FOCAL POINT These electrodes are connected to the UTZ machine which The location where the generates the short burst of electrical pulses to excite the beam reaches its crystals. minimum diameter. PIEZOELECTRIC ELEMENT SIDE LOBES o Other term: transducer Energy from the transducer that element, active element, radiates at various angles from crystals the transducer face. o Most important component ARRAY o Approx. 6-19 mm in The arrangement of crystals within the transducer. diameter and 0.2-2 mm in thickness o Crystalline materials composed of dipolar molecules. TYPES OF ELECTRONIC ARRAY TRANSDUCER Quartz - naturally occurring material with piezoelectric All the different types of transducers currently available can properties. be characterized into 3 main types: LEAD ZIRCONATE TITANATE (PZT) o Commonly used materials LINEAR ARRAY o man-made ceramic o more efficient, better sensitivity and can be easily shaped. CURVILINEAR (Sector) ARRAY TRANSDUCER Canteras, C.f. 3. Probes and probe cords should be cleaned both PHASED ARRAY TRANSDUCER before and after use. o High level disinfection (HLD) - is a more thorough cleaning method that often recommended for cleaning Endo cavitary ❖ footprint of a probe refers to the physical size of probes after use. the part of the ultrasound that contacts the TRANSDUCENT CARE AND CLEANING patients. 4. Probe covers and sheaths – protect probes from ❖ The field of view is the width of the image that contamination and the patient from potential is seen on the screen. infection. For example: COUPLING MEDIUM The field of view of a linear array probe is constant ✓ Usually an aqueous gel. and is the size of the probe's footprint. The field of view of a ✓ Improves sound transmission into and out of curved array probe diverges as it exits the probe and is not the patient by eliminating air reflection. constant. ✓ Composition: EDTA, Carbomer, Propylene LINEAR ARRAY glycol, Trolamine, distilled water Produces parallel scan lines and has a rectangular LESSON 4 field of view. DIAGNOSTIC ULTRASOUND Used to image superficial structures and vessels and INSTRUMENTATION AND OPERATION therefore operate at frequencies above 4MHz. RESOLUTION Extensively used for vascular, small parts and The ability of an imaging system to differentiate between musculoskeletal applications. structures, images, or events and display them as a separate entities. Imaging resolution has three aspects: CURVILINEAR ARRAY TRANSDUCERS 1. Spatial Resolution Similar to the linear array but the transducer face is 2. Contrast Resolution formed into a curve (convex in shape) which 3. Temporal Resolution provides a wide field of view which diverges with SPATIAL RESOLUTION depth. The ability to display two structures situated close together Sometimes referred to as SECTOR ARRAYS. as separate image. Operate at lower frequencies, typically around 3.5 Divided into two components: MHz and are best suited to image deep lying A. Axial Resolution structures. B. Lateral Resolution Main applications are in abdominal and obstetric AXIAL RESOLUTION scanning. ✓ Ability to display small targets along the path of the beam as separate entities. ✓ Resolution along the axis of the beam. PHASED ARRAY ✓ Sometimes called as LONGITUDINAL resolution. ✓ Depends on SPL Axial Resolution (mm) = Spatial Pulse Length / 2 LATERAL RESOLUTION INVASIVE TRANSDUCERS ✓ Ability to distinguish two separate targets Transducer designed to enter the body via the vagina, rectum, esophagus or a blood vessel perpendicular to the beam path (same distance (catheter-mounted type). from the transducer). ✓ Sometimes called as AZIMUTHAL resolution. ✓ Depends upon the width of the ultrasound beam (best where the beam is narrowest) Axial Resolution (mm) = Spatial Pulse Length / 2 TRANSDUCER CARE AND CLEANING CONTRAST RESOLUTION ✓ Ability of the imaging system to differentiate 1. Ultrasound transducers should not be sterilized. between tissues and display them as different o Curie point is the temperature at which shades of gray. polarization in a crystal is lost. Heating the TEMPORAL RESOLUTION piezoelectric crystal above the curie ✓ Ability of the imaging system to display events which temperature renders the crystal useless. For occur at different times as separate images. this reason, transducers must never be ✓ Particularly important in echocardiography. sterilized in an autoclave. ✓ Determined by frame rate. The approximate Curie points for several crystals are as ✓ Frame rate - no. of images per second. follows: ✓ Quartz - 573 degree Celsius ✓ Ba. Titanate - 100 degree Celsius LESSON 5 ✓ PZT-4 - 328 degree Celsius INSTRUMENTATION AND CONTROLS ✓ PZT5A - 365 degree Celsius SECTIONAL VIEWS 2. UTZ transducers should not be dropped. AND IMAGE ORIENTATION Canteras, C.f. ULTRASOUND SCANNING MODE DEPTH BI-STABLE SCANNING ✓ The depth controls how much distance into the ✓ Displays images in black and white combinations body the image displays in the far field. only. ✓ Used to adjust the size of the image so that organs ✓ Obsolete and adjacent structures or regions of interest are A-MODE equally well visualized. ✓ Amplitude mode ✓ When depth is decreased, superficial structures will ✓ A single dimension display consisting of a horizontal be magnified. baseline. ✓ Too much depth can be wasted space. ✓ This baseline represents time and or distance with GAIN upward (vertical) deflections (spikes depicting the ✓ Is a knob that adjust the overall UTZ echo signal. acoustic interface). ✓ As the gain increased, the strength of the returning ✓ Used in ophthalmology. echoes is amplified, which produces a brighter image. ✓ A decrease in gain will darken the image visualization on the monitor. TIME GAIN COMPENSATOR ✓ TGC may be set up as a column of sliding knobs or may be adjusted for near and far gain. ✓ Allows selective control of gain at different depths. UTZ waves returning from deeper structures have undergone greater attenuation. To compensate for the loss of signal intensity, TGC allows for stepwise increase in gain to B-MODE compensate for greater attenuation of ultrasound waves ✓ Brightness mode returning from deeper structures. Time gain compensation ✓ A two-dimensional display of the ultrasound. controls should be moved to the right in a stepwise fashion ✓ The A-mode spikes are electronically converted into to “amplify” the returning signal from deeper structures. dots and displayed at the correct depth from the ZOOM transducer. ✓ The zoom function allows for the magnification of ✓ Common to all clinical applications, also called as B- one area on the screen. scan or gray scale sonography. ✓ This control is used to magnify structures of interest. M-MODE KEYBOARD ✓ Motion mode ✓ Various capabilities as provided by the ✓ Used to visualize things that are physically moving. manufacturer. The motion occurring in a one-dimensional scan line FOCUS is displayed on the vertical axis over time on the ✓ Within transducers, there is a FOCUS which horizontal axis. concentrates the sound beam into a smaller beam ✓ It is used in conjunction with B-mode scanning. area than would exist otherwise. ✓ This mode is most commonly used in the evaluation ✓ This area of focus is where you will obtain your best of cardiac valves and fetal heart activity. images. ✓ It can be found on the monitor (arrow), on the vertical millimeter scale. DYNAMIC RANGE ✓ Determines how many shades of gray are demonstrated on an image. ✓ Decreasing dynamic range gives fewer grays and increases contrast. ✓ Increasing dynamic range gives a wider range of grays and decreases contrast. EXTENDED FIELD OF VIEW ✓ Determines how many shades of gray are demonstrated on an image. DOPPLER ✓ Decreasing dynamic range gives fewer grays and Doppler uses the frequency shift of sound waves to measure increases contrast. velocity, typically blood. ✓ Increasing dynamic range gives a wider range of The most common used modes: grays and decreases contrast. o Color flow doppler MANIPULATING AND SAVING THE IMAGE o CFD Power or Angiodoppler FREEZE o Spectral doppler (pulsed and continuous wave) ✓ The freeze button is used to create a still image on CONTROLS the monitor. This control captures a single frame ADJUSTING THE B-MODE IMAGE from a dynamic image. Designed to regulate the intensity echoes from ✓ Most machines capture several seconds of still various depths. images, allowing selection of a previous image after pressing the freeze button. Canteras, C.f. ✓ After freezing the scan, the sonographer can add text, make measurements or calculations, save or print the image. CINE LOOP ✓ The cine loop functions allow the operator to review a sequence of acquired images. Many frames can be stored in a computer memory for immediate playback using the cine loop. ✓ The slow motion (frame by frame) review capability allows the sonographer to select an appropriate image for the study. CALIPERS ✓ Available to measure distances. An added feature in some units is the ellipsoid measurement. A dotted line can be created around the outline of a structure to either the circumference or the area. ✓ Basic calculations, such as measurement, will be available on all images. ACQUIRING IMAGES ✓ Images may be captured for archival or export. Still images may be saved by freezing the image and then hitting the appropriate button (acquire, save, store, etc.)