Chapter 08 Control Of Gene Expression PDF
Document Details
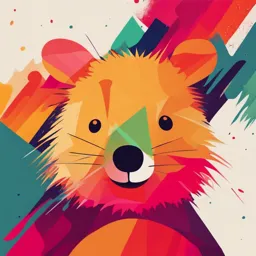
Uploaded by IncredibleNephrite
Queens College, CUNY
Tags
Summary
This document discusses the control of gene expression in various organisms, including eukaryotes and prokaryotes. It investigates the processes involved in gene expression, such as transcription, translation, and regulation, highlighting the role of transcription factors in regulating gene activity.
Full Transcript
Chapter 08 Control of Gene Expression The human neuron and liver cell have same genome •But express different RNAs and proteins •A typical cell expresses ~20-50% of its 25,000 genes •A cell can change the expression of its genes in response to external signals Figure 8-1 Eukaryotic gene expressi...
Chapter 08 Control of Gene Expression The human neuron and liver cell have same genome •But express different RNAs and proteins •A typical cell expresses ~20-50% of its 25,000 genes •A cell can change the expression of its genes in response to external signals Figure 8-1 Eukaryotic gene expression can be controlled at many steps from DNA --> RNA --> Protein •Transcriptional control is most common control point Figure 8-3 Protein concentration regulation Lodish textbook Differentiated cells contain all the genetic info to form a complete organism •Cells can change the genes they express without altering the nt sequence of their DNA Figure 8-2c How do we determine which genes are expressed? — identifying all mRNAs found in certain conditions Change in conditions during expt. •Yeast grown in glucose deplete it as ethanol is made, they then use ethanol as a carbon source. Yeast at early stages will express genes for glucose utilization and later for ethanol utilization How do we determine which genes are expressed? — identifying all mRNAs found in certain conditions •mRNAs from cells in glucose are made green; mRNAs from cells in EtOH/no glc are made red •DNA chip (microarrays) made with many dots, each dot containing only 1 gene •Intensity of red or green binding to a spot containing one gene indicates number of mRNAs expressed • yellow spots indicate mRNAs from both cells expressed •Blank spots indicate genes not expressed How do we determine which genes are expressed? — identifying all mRNAs found in certain conditions •mRNAs from cells in glucose are made green; mRNAs from cells after glucose depleted are made red •DNA chip (microarrays) made with many dots, each one containing only 1 gene •Intensity of red or green binding to a spot containing one gene indicates number of mRNAs expressed • yellow spots indicate mRNAs from both cells expressed •Blank spots indicate genes not expressed Results of DNA microarray Green– genes expressed when glucose utilized Red-- genes expressed when EtOH utilized Yellow-- mRNA equal to both conditions Black-- no mRNAs for that gene present Note: colors here are not the mRNA colors but represent the relative levels of expression of each gene. Catabolized by glycolysis Catabolized by TCA cycle Ethanol decreasing with time TCA genes used for ethanol, not glucose (glycolysis) TCA (Krebs) cycle genes Currently we do direct sequencing of cDNAs made from mRNA Identifying tumor source by which genes are expressed <--1800 different genes--> <--142 different tumors --> •Higher than average expression are red and lower than average are green • Each class of tumors has its characteristic spectrum of expressed genes. •In this case the unk type yellow tumor was judged to be a lung cancer because its pattern of gene expression was like those of a series of already-identified lung cancers. •RNAP II + general transcription factors = low-level expression •Specific transcription factors determine: -- which promoters assemble preinitiation complexes -- rate at which RNAP initiates new rounds of transcription Transcription is controlled by proteins binding to regulatory DNA sequences • gene expression begins at the promoter, where transcription is initiated. • In selective gene transcription a “decision” is made about which genes to activate. • DNA regulatory sequences are used to switch genes on/off • Transcriptional regulators bind to these sequences (~10% of protein encoding genes are devoted to transcription activators) • In the most general sense, two types of regulatory proteins may bind DNA— repressor proteins and activator proteins. Turning on a gene Positive Regulation -- Activators Enhancer regions Positive regulation —Gene is not normally transcribed; an activator protein binds to stimulate transcription. Negative Regulation -- Repressors Silencer regions Negative regulation —Gene is normally transcribed but binding of a repressor protein prevents transcription. Binding of a transcriptional regulator to the major groove of the DNA helix •Surface of protein fits tightly against special nt-specific surface features of the double helix in that region •Usually binds to major groove with ionic, hydrogen and hydrophobic interactions without disrupting the base pair hydrogen bonds •Only one contact is shown, but usually 10-20 base pair to protein contact is made Figure 8-4 DNA binding motifs • • • Structural polypeptide domains found in most transcription regulators Homeodomains, zinc finger and leucine zipper Found in transcription regulators that control the expression of thousands of different genes A, B - homeodomain C - zinc finger D - leucine zipper Figure 8-4,5 Transcription Activators A cluster of bacterial genes can be activated by a single promoter •Coordinate expression of genes making enzymes in same pathway •If trp conc. low, bacterium expresses enzymes needed to make trp; if trp abundant, these genes are repressed Figure 8-6 Tryptophan operon - coordinate expression of 5 genes •Level of tryptophan regulates the activity of the polymerase transcribing the tryptophan operon •Inactive repressor is constitutively expressed Figure 8-7 Comparison of gene organization, transcription and translation All proteins in same metabolic pathway Lodish 6th Eukaryotic gene activation occurs at a distance 100-1000’s nt apart •Looping of DNA allows contact between activator protein bound to enhancer and transcription complex bound to promoter •In euk usually the TF bind indirectly via mediators •Promotes or represses assembly of initiation complex Figure 8-10 Transcription •Drawn to scale •In real time Activator protein Packing of promoter DNA into nucleosomes affects the initiation of transcription by modulating chromatin structure •Activator proteins can recruit histone modifying enzymes and chromatin-remodeling complexes to the promoter region •Acetyl groups can 1. attract proteins that promote transcription 2. Recruit chromatinremodeling complexes Figure 8-11 Regulation by alteration of chromatin structure • Nucleosomes contain DNA and histones in a tight complex, inaccessible to RNA polymerase. • Each histone has a positively charged “tail” at its N-terminus. • Histone acetyltransferases change the tail’s charge by adding acetyl groups to the amino acids (less “+” so lower affinity for “-” charge on DNA. • This opens nucleosomes and activates transcription. • Activators attract histone acetylases and repressors attract histone deacetylases See Figure 8-11 A single transcription regulator can coordinate the expression of many different genes •In eukaryotes, many genes involved in same pathway are on different chromosomes •Series of genes with activator proteins bound; however, not efficient activation •Additional transcription regulator (glucocorticoid receptor + hormone) binds to the regulatory sequence of these genes •Now efficient expression of genes as a set Figure 8-16 Example -- Transcription factors for PEPCK gene PEPCK -- phosphopenolpyruvate carboxykinase catalyzes a rate-controlling step in gluconeogensis How Is Eukaryotic Gene Expression Regulated After Transcription? • Eukaryotic gene expression can be regulated in the nucleus before/after mRNA export • Control mechanisms include alternative splicing of pre-mRNA, microRNAs, translation repressors, or regulation of protein breakdown. Alternative Splicing Results in Different Mature mRNAs and Proteins • • • Different mRNAs can be made from the same gene by alternative splicing. As introns and exons are spliced out, new proteins are made. A mechanism for generating proteins with different functions, from a single gene. mRNA translation can be regulated Protein and mRNA concentrations are not consistently related—governed by factors acting after mRNA is made. Cells can block mRNA translation or alter how long new proteins persist in the cell. Multiple ways to regulate mRNA translation: • miRNAs can inhibit translation • GTP cap on 5′ end of mRNA can be modified—if cap is modified mRNA is not translated • Repressor proteins can block translation directly mRNA Inhibition by MicroRNAs • MicroRNAs (miRNAs)—small molecules (~22 nt) of noncoding RNA—are important regulators of gene expression. • Humans have ~ 500 miRNAs that regulate ~ 1/3 of protein-coding genes • RISC (RNA-induced silencing complex) proteins guide miRNA to target mRNA— translation is inhibited and mRNA is degraded. • A single miRNA can regulate many mRNAs with common 5’ or 3’ UTR Figure 8-27 Gut epithelial cells can control which bacteria live in intestine Cell Host Microbe. 2016 Control of mRNA translation — ferritin translation regulation •Lots of free iron in cytoplasm is harmful to cells •ferritin protects cell by binding free iron •LOW IRON: IRP (iron regulatory protein) binds to IRE (iron-response element) in 5’ UTR (un-translated region) and blocks ribosome binding •HIGH IRON: IRP changes shape and releases from IRE; translation begins and ferritin made to bind free iron Regulation of protein stability • Protein longevity is regulated—protein content is a function of synthesis and degradation. • Ubiquitin attaches to a protein to mark for destruction • This complex binds to a proteasome —a large complex where the ubiquitin is removed and the protein is digested. Cytoplasmic localization of mRNAs •Cell needs actin near leading edge of cell for locomotion •mRNA for b-actin is inhibited until localized to leading edge