Unit 3 - Neurophysiology and neural communication PDF
Document Details
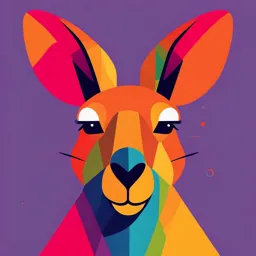
Uploaded by FeasibleNewYork5003
Universidad Europea
Tags
Summary
This document covers neurophysiology and neural communication, including resting potential, action potentials, synapses, and neurotransmitters. It is lecture material from the Universidad Europea.
Full Transcript
Unit 3 – Neurophysiology and neural communication 1. Neurophysiology: resting potential....
Unit 3 – Neurophysiology and neural communication 1. Neurophysiology: resting potential. 2. Action potential 3. Neural communication: synapses. 4. Neurotransmitters Degree in Physiotherapy – Structure and Function: Systems I Department of Rehabilitation 1. NEUROPHYSIOLOGY 1.1 Basal situation of neurons 1.2 Equilibrium potential 1.3 Resting membrane potential 1. NEUROPHYSIOLOGY 1.1. Basal situation of neurons. PREVIOUS BACKGROUND Composition of intracellular and extracellular fluids Gradients Ionic transport down or against the gradient Semipermeable membrane: Only allows the passing of certain molecules Unit 3 – Neurophysiology and neural communication 1. NEUROPHYSIOLOGY 1.1. Basal situation of neurons. PREVIOUS BACKGROUND: COMPOSITION [PO-4] (ICF) Extracellular fluid (ECF) [Cl-] [CO2] [Na+] [Glucose] [O2] (ECF) [aas] [HCO3-] Membrane (ECF) [Proteins-] [PO-4] [Na+] [aas] (ICF) Intracellular fluid [Mg+] (ICF) [Cl-] [K+] [HCO3-] Unit 3 – Neurophysiology and neural communication 1. NEUROPHYSIOLOGY 1.1. Basal situation of neurons. PREVIOUS BACKGROUND: GRADIENTS Chemical gradient: Responsible for the movement of particles from one side to other of a semipermeable membrane. It depends on the final CONCENTRATION of each particle on both sides. Electrical gradient: Responsible for the movement of charged particles from one side to the other of a semipermeable membrane. It depends on the final balance of ELECTRICAL CHARGES in each compartment. ELECTROCHEMICAL GRADIENT Unit 3 – Neurophysiology and neural communication 1. NEUROPHYSIOLOGY 1.1. Basal situation of neurons. PREVIOUS BACKGROUND: GRADIENTS Electrochemical gradient: Responsible for the movement of particles from one side to the other of a semipermeable membrane. It depends on the final balance of charges and concentration, on both compartments Unit 3 – Neurophysiology and neural communication 1. NEUROPHYSIOLOGY 1.1. Basal situation of neurons. PREVIOUS BACKGROUND: TRANSPORT Ionic channels: Membrane proteins that allow the passing of ions (Na+, K+, Cl‐, etc…) from one side of the membrane to the other. There are two types depending on the conformation of the inner pore: Passive channels: They are always open. Gated channels: They can be either open or closed. Gating depends on the type of channel: LIGAND-GATED CHANNELS VOLTAGE-GATED CHANNELS Unit 3 – Neurophysiology and neural communication 1. NEUROPHYSIOLOGY 1.1. Basal situation of neurons. PREVIOUS BACKGROUND: TRANSPORT LIGAND-GATED CHANNELS Unit 3 – Neurophysiology and neural communication 1. NEUROPHYSIOLOGY 1.1. Basal situation of neurons. PREVIOUS BACKGROUND: TRANSPORT VOLTAGE-GATED CHANNELS Voltage sensor: each cannel opens or closes at a certain membrane voltage. Unit 3 – Neurophysiology and neural communication 1. NEUROPHYSIOLOGY 1.1. Basal situation of neurons. PREVIOUS BACKGROUND: SELECTIVE PERMEABILITY Depends on: The electrochemical gradient of ions The channels present on the membrane The open or closed state of those channels Unit 3 – Neurophysiology and neural communication 1. NEUROPHYSIOLOGY 1.1. Basal situation of neurons. Initial state: Electrical neutrality in both compartments but uneven charge distribution in each side of the membrane 3 Na+ [Na ] + [K+] [Na ] + 2 K+ - + - + - + [K+] - + Proteins (- charge) + charges = - charges + charges = - charges 0 mV -70 mV (difference in electric potential between the exterior and the interior) The exterior is positively charged compared to the interior Unit 3 – Neurophysiology and neural communication 1. NEUROPHYSIOLOGY 1.1. Basal situation of neurons. The cell is like a small battery: Charge difference: voltage – Positive terminal: exterior Changes less than 1 dV: enough to maintain physiological processes. – Negative terminal: interior All the cells posses their own membrane potential. Only a few can change it in response to stimuli: excitable cells. Unit 3 – Neurophysiology and neural communication 1. NEUROPHYSIOLOGY 1.1. Basal situation of neurons. Chemical or concentration gradients elicit ionic movement across the membrane. This induces an uneven charge distribution across the membrane, generating also an electrical gradient. Chemical gradient + electrical gradient = electrochemical gradient. The electrochemical gradient generates an electrical potential that is called resting membrane potential. Unit 3 – Neurophysiology and neural communication 1. NEUROPHYSIOLOGY 1.2. Equilibrium potential. What would happen if the cell was permeable to only one ion? Defined by the Nerst Equation Considers the concentrations of the ion in both sides of the membrane (only concentrations, not permeability) Eion = 2,303 RT/zF log [ion]o/[ion]i Eion: equilibrium potential; R=constant: 0,082;T=temperature; Z=ionic valence; F=Faraday constant Equilibrium potential: the value of electrical potential that compensates the chemical gradient. The ion flux is in equilibrium (net flux=0). Unit 3 – Neurophysiology and neural communication 1. NEUROPHYSIOLOGY 1.2. Equilibrium potential. Potassium moves outwards according to its concentration gradient, K+ - + but this is against its electrical gradient (is + and goes to the positive side). 150 mM 5 mM K+ equilibrium potential: potential value when the concentration and electrical gradients are even. Ek =61mV log 5 mEq/L / 150 mEq/L = - 90 mV Na+ Sodium moves inwards according to its concentration gradient, and this is also down its electrical gradient (is + and goes to the - + negative side). 12 mM 145 mM Na+ equilibrium potential: potential value when the concentration and electrical gradients are even. ENa =61mV log 145 mEq/L / 12 mEq/L = +66 mV Unit 3 – Neurophysiology and neural communication 1. NEUROPHYSIOLOGY 1.3. Resting membrane potential. Potential difference: negative interior maintained by: – Na+/K+ pump: takes out 3 charges (+) and only introduces 2. – Lots of (‐) charges inside (proteins). Presence of two gradients: chemical and electrical. – Permeability to K+ is higher due to more abundant channels. – Its movement is slower because is against the electric gradient. Unit 3 – Neurophysiology and neural communication 1. NEUROPHYSIOLOGY 1.3. Resting membrane potential. Why the resting membrane potential in neurons is ‐70 mV? Because of the uneven distribution of ions in both sides of the membrane. Each ion contributes to the potential difference according to: – 1. Its concentration gradient – 2. Its permeability. Cell membranes are more permeable to K+, thus membrane potential is mainly determined by the potassium gradient. Unit 3 – Neurophysiology and neural communication 1. NEUROPHYSIOLOGY 1.3. Resting membrane potential. If the membrane was only permeable to K+ resting membrane potential would be -90 mV, the value for the equilibrium potential of K+. This is the case for most of the cells in our bodies, including muscle cells. However, neurons also have non‐gated channels (permeability) for Na+, although K+ channels are much more abundant. Therefore, if the cell is permeable to more than one ion, concentration in both sides of the membrane is not enough to calculate the potential (Nerst equation). Permeability also needs to be considered. Unit 3 – Neurophysiology and neural communication 1. NEUROPHYSIOLOGY 1.3. Resting membrane potential. When there is permeability for more than one ion, we use the GOLDMAN‐ HOLDGKIN‐KATZ equation to calculate the membrane potential. Vm = 61,54 mV log (Pk+[K+]o + PNa+[Na]o / PK+ [K+]i + PNa+[Na]i) It considers both the membrane’s relative permeability for the different ions and their concentrations in both sides of the membrane. If a cell is only permeable to one ion, its resting membrane potential is the value for the equilibrium potential of the ion. Unit 3 – Neurophysiology and neural communication 1. NEUROPHYSIOLOGY 1.3. Resting membrane potential. Resting membrane potential for a neuron in ‐70mV, the main ion responsible for this value is K+, but it doesn’t match its equilibrium potential because neurons are also permeable to Na+. 3 Na+ [Na ] + [K+] [Na ] + 2 K+ - + - + - + [K+] Proteins (- charges) - + + charges = - charges + charges = - charges -70 mV 0 mV Unit 3 – Neurophysiology and neural communication 1. NEUROPHYSIOLOGY 1.3. Resting membrane potential. But at that potential value (‐70mV) neither sodium nor potassium are at equilibrium: – Na+ still goes inwards down electrochemical gradient. – K+ goes outwards more than inwards: the driving force of the electric potential is less powerful than the generated by the chemical gradient. If this situation goes on, final Na+ concentration inside the cell would increase over acceptable limits, and the same would happen for K+ (would decrease its inner concentration below physiological values). So there has to be something that prevents this situation, and that maintains the chemical gradients for Na+ and K+, and keeps their concentrations within a physiological range both in the extracellular and intracellular fluid. Unit 3 – Neurophysiology and neural communication 1. NEUROPHYSIOLOGY 1.3. Resting membrane potential. And that something is the Na+/K+ pump that immediately restores all the incoming Na+ by taking it out, and pumps in the K+, with an energy input in the form of ATP. That’s why this pump, together with non‐gated channels, has a key role in the generation and maintenance of the resting potential. Unit 3 – Neurophysiology and neural communication 2. ACTION POTENTIAL 2.1 Characteristics of the action potential 2.2 Propagation of the electrical signal 2. ACTION POTENTIAL 2.1. Characteristics of the action potential. Excitable cells (neurons, muscle cells) are able to modify their resting membrane potential. These cells can generate an action potential in response to a stimulus that reaches a certain threshold. Threshold: voltage limit that has to be reached in the membrane to trigger an action potential. The action potential is a depolarizing voltage change (potential reaches more positive values than ‐70mV), but: ‐ Not all voltage changes are depolarizing. ‐ Not all depolarizations are action potentials Unit 3 – Neurophysiology and neural communication 2. ACTION POTENTIAL 2.1. Characteristics of the action potential. The potential changes due to changes in the permeability of the membrane: gated channels open, altering the basal permeability. Types of potential changes: -30mV -50mV Depolarization Repolarization -70mV -90mV Hyperpolarization Depolarization: Voltage change that turns the potential more positive than -70mV. Hyperpolarization: Voltage change that turns the potential more negative than -70mV. Repolarization: Voltage change that returns the potential to resting values (-70mV). Unit 3 – Neurophysiology and neural communication 2. ACTION POTENTIAL 2.1. Characteristics of the action potential. It depends on the voltage‐gated channels located on the axon: they are only open when a neuron needs to propagate information to the following cell (neuron or not). It needs an stimulus over the threshold: depolarization over ‐55 mV (causing the opening of voltage‐gated Na+ channels). “All‐or‐none” principle: – Once the threshold is reached, the shape, amplitude and duration of action potentials is always the same, disregarding the intensity of the stimulus. Stronger stimuli increase the triggering frequency, not the amplitude, that is always 100 mV. – The amplitude does not diminish with the propagation. Unit 3 – Neurophysiology and neural communication 2. ACTION POTENTIAL 2.1. Characteristics of the action potential. 1. Resting membrane potential. 2. Depolarizing stimulus (effect of the neurotransmitters received by the dendrites, enough depolarization to reach the threshold). 3. At the threshold (-55 mV), voltage-gated Na+ channels open (increase permeability) 4. Rapid Na+ entry (down electrochemical gradient) depolarize the neuron up to +30 mV. 5. Voltage-gated Na+ channels close and voltage-gated K+ channels open. K+ moves outwards down its chemical gradient. Repolarization begins. Unit 3 – Neurophysiology and neural communication 2. ACTION POTENTIAL 2.1. Characteristics of the action potential. 6. K+ moves outwards looking for its equilibrium potential (-90mV). 7. When the potential reaches the resting value (-70 mV), K+ channels close but slowly, so K+ can still move out of the neuron, driving the hyperpolarization. 8. Slow voltage-gated K+ channels are already closed, so less K+ goes out of the neuron. The Na+/K+ pump drives a second repolarization. 9. The neuron recovers its resting permeability (only non-gated channels are open now) and the Na+/K+ pump restores the resting membrane potential. Unit 3 – Neurophysiology and neural communication 2. ACTION POTENTIAL 2.1. Characteristics of the action potential. The amount of sodium that enters the neuron and potassium that leaves it are restored by the activity of the Na+/K+ pump, that never stops (as long as ATP is available). These amounts are insignificant for the concentration gradients, but enough to induce the electrical changes. Unit 3 – Neurophysiology and neural communication 2. ACTION POTENTIAL 2.1. Characteristics of the action potential. Potassium voltage-gated channel A B Exterior Interior K+ K+ Sodium voltage-gated channel Unit 3 – Neurophysiology and neural communication 2. ACTION POTENTIAL 2.1. Characteristics of the action potential. Ionic movement and voltage-gated channels during the phases of the action potential Unit 3 – Neurophysiology and neural communication 2. ACTION POTENTIAL 2.1. Characteristics of the action potential. When an area of the membrane is triggering an action potential, it becomes refractory: unable to respond to a second stimulus. There is a minimum period of time that prevents the generation of a second action potential before the first one has finished: REFRACTORY PERIOD. Ensures the propagation of action potentials in only one way. Unit 3 – Neurophysiology and neural communication 2. ACTION POTENTIAL 2.1. Characteristics of the action potential. We can differentiate two sub periods within the refractory period: Absolute: voltage‐gated Na+ channels are closed AND inactive (unable to open). There can’t be a depolarization so the neuron can’t trigger a second action potential. Relative: due to continuous outflow of K+ during hyperpolarization. Voltage‐ gated Na+ channels are closed but can be opened. The neuron can trigger a second action potential if the stimulus is stronger than the first one (enough to reach the threshold, that is now farther). Unit 3 – Neurophysiology and neural communication 2. ACTION POTENTIAL 2.2. Propagation of the electrical signal. After an action potential is triggered in the axon hillock, it has to travel along the axon until it reaches the axon terminal, where the neurotransmitter vesicles are stored: PROPAGATION OR CONDUCTION. Propagation only happens in one direction (from axon hillock to axon terminal) thanks to the refractory period. Unit 3 – Neurophysiology and neural communication 2. ACTION POTENTIAL 2.2. Propagation of the electrical signal. Propagation speed is directly related to: Axon diameter. More diameter, higher speed. Myelination Distance between nodes of Ranvier (1‐2mm). The longer the distance, the higher the speed. Temperature Diameter (µm) Propagation speed (m/s) Function examples Sensory: muscle position Motor somatic axons Sensory: touch, pressure Sensory: pain, temperature Autonomous axons towards ganglia Autonomous axons towards smooth and cardiac muscle Unit 3 – Neurophysiology and neural communication 2. ACTION POTENTIAL 2.2. Propagation of the electrical signal. MYELIN Substance produce by certain glial cells that coats axon to speed up the propagation of the action potential. Oligodendrocytes (CNS) Schwann cells (PNS) Produce myelin for the axons in the Produce myelin for the motor axons white matter (in the gray matter there (sensory axons are mostly unmyelinated). are unmyelinated axons). Each Schwann cell coats only one axon. Each oligodendrocyte coats several axons. Unit 3 – Neurophysiology and neural communication 2. ACTION POTENTIAL 2.2. Propagation of the electrical signal. The presence and the amount of myelin present on an axon determines the type of propagation or conduction. 1. Unmyelinated axons: The action potential has to regenerate in each portion of the axon, as the sodium-gated channels are equally distributed along. Slower propagation speed. CONTINUOUS CONDUCTION Unit 3 – Neurophysiology and neural communication 2. ACTION POTENTIAL 2.2. Propagation of the electrical signal. Continuous conduction The propagation follows only one direction, down to the axon terminal, because the axon membrane proximal to the soma remains in refractory period, so depolarization can only occur distally. Unit 3 – Neurophysiology and neural communication 2. ACTION POTENTIAL 2.2. Propagation of the electrical signal. 2. Myelinated axons. Myelin has insulating properties that prevent current leaks. Thus, where a myelin sheath is placed, the action potential can move from one naked area (node of Ranvier) to the next without losing properties. Sodium‐gated channels concentrated on these nodes to reinforce the depolarization and make the signal “jump” to the next node. This increases the propagation speed, as less action potentials are needed to reach the action terminal. SALTATORY CONDUCTION Unit 3 – Neurophysiology and neural communication 3. NEURAL COMMUNICATION: SYNAPSES 3.1 Definition and types 3.2 Chemical synapse mechanism 3.3 Outcome of the synaptic transmission 3.4. Signal integration Unit 2 – Nervous System 3. NEURAL COMMUNICATION: SYNAPSES 3.1. Definition and types. Synapse: communication mechanism between a neuron and a target cell. It involves and action potential in a neuron releasing neurotransmitters into the synaptic space, reaching the target cell and inducing a response. When a synapse occurs between two neurons, the neuron triggering the action potential is called PRESYNAPTIC, and the neuron receiving the neurotransmitters is called POSTSYNAPTIC. Types of synapses – Electrical: cells are physically coupled – Chemical: cell do not touch each other Unit 3 – Neurophysiology and neural communication 3. NEURAL COMMUNICATION: SYNAPSES 3.1. Definition and types. Electrical synapses Cells are electrically coupled through GAP junctions, pores that allow the flux of ions directly between cytoplasms. Occurs in: – Cardiac cells – Smooth muscle cells Unit 3 – Neurophysiology and neural communication 3. NEURAL COMMUNICATION: SYNAPSES 3.1. Definition and types. Chemical synapses Abundant in the CNS, where neurons do not touch each other or target cells. A presynaptic neuron releases a chemical messenger (neurotransmitter) The neurotransmitter (NT) binds specific receptors located on the target cell, inducing a response (changes in membrane potential when it is a postsynaptic neuron). Unidirectional Unit 3 – Neurophysiology and neural communication 3. NEURAL COMMUNICATION: SYNAPSES 3.2. Chemical synapse mechanism. Pre‐synaptic terminal (axon) The presynaptic cell is always a neuron. The postsynaptic cell is either a neuron or other target cell. Postsynaptic terminal (dendrite) Neurotransmitter vesicles Presynaptic neuron Postsynaptic neuron Unit 3 – Neurophysiology and neural communication 3. NEURAL COMMUNICATION: SYNAPSES 3.2. Chemical synapse mechanism. Binding of the NT to its receptor may induce changes in the membrane potential of the postsynaptic neuron: depolarization and hyperpolarization Unit 3 – Neurophysiology and neural communication 3. NEURAL COMMUNICATION: SYNAPSES 3.2. Chemical synapse mechanism. When the action potential of a presynaptic neuron reaches the axon terminal, exocytosis is triggered by calcium entrance, releasing the NT vesicles. NT NT cross the synaptic space until they reach the postsynaptic membrane with their receptors (Rc). Rc Unit 3 – Neurophysiology and neural communication 3. NEURAL COMMUNICATION: SYNAPSES 3.3. Outcome of the synaptic transmission. Binding of the NT to its receptor (ligand‐gated channel) changes the basal permeability and induces a voltage change in the postsynaptic neuron: unitary postsynaptic potential Postsynaptic potentials can be: – Excitatory postsynaptic potential (EPSP): the NT binds a receptor that is a Na+ channel, causing depolarization. NT sucha as glutamate or acetylcholine produce EPSP. Na+ Na+ EPSP Na+ Na+ Unit 3 – Neurophysiology and neural communication 3. NEURAL COMMUNICATION: SYNAPSES 3.3. Outcome of the synaptic transmission. – Inhibitory postsynaptic potential (IPSP): the NT binds a receptor that is either a K+ or a Cl‐ channel and induces hyperpolarization. The main inhibitory NT is GABA. K+ K+ + Cl- Cl- IPSP K K+ Cl- Cl- IPSP The same postsynaptic neuron will receive EPSP and IPSP through its dendrites, as several NT can be released at the same time over it. The soma integrates these unitary changes so that if the intensity of EPSP overcomes IPSPs and is enough to reach the threshold (‐55mV), the postsynaptic neuron will trigger an action potential, becoming a presynaptic neuron for the following synapse. Unit 3 – Neurophysiology and neural communication 3. NEURAL COMMUNICATION: SYNAPSES 3.3. Outcome of the synaptic transmission. It is important to completely remove the NT from the synaptic cleft once the transmission is over (when the action potential at the axon terminal finishes). Three mechanisms: ‐ Diffusion of the NT to adjacent glial cells or blood vessels. ‐ Enzymatic degradation of the NT in the synaptic cleft. ‐ Re‐uptake into the presynaptic terminal (active transport). At least two mechanisms are needed to ensure rapid removal, and normally all three can be found at synapses in the CNS. Unit 3 – Neurophysiology and neural communication 3. NEURAL COMMUNICATION: SYNAPSES Unit 3 – Neurophysiology and neural communication 3. NEURAL COMMUNICATION: SYNAPSES 3.4. Signal integration. Triggering an action potential in the postsynaptic neurons requires the participation of several synapses: Summation. Depending on how each synapse get to the soma: Spatial summation Temporal summation TRIGGER AREA: axon hillock Unit 3 – Neurophysiology and neural communication 3. COMUNICACIÓN NEURONAL: SINAPSIS 3.4. Mecanismos de integración de la señal. Spatial summation When two or more presynaptic afferents fire at the same time, each one produces its EPSP. Each single EPSP does not reach the threshold potential By adding the three EPSPs, threshold is reached and AP is triggered. Tema 3 – Neurofisiología y comunicación neural 3. NEURAL COMMUNICATION: SYNAPSES 3.4. Signal integration. It may also happen that an IPSP from an inhibitory neuron decreases the value of the EPSPs generated by other excitatory neurons No action potential is generated Unit 3 – Neurophysiology and neural communication 3. NEURAL COMMUNICATION: SYNAPSES 3.4. Signal integration. TEMPORAL SUMMATION When the same presynaptic fiber increases its firing frequency several in quick succession, the EPSPs overlap to reach the threshold Two potentials lower than the threshold potential, reach Two potentials lower than the threshold, arriving at the trigger zone very close in time, add up and can the trigger zone separated in time, cannot generate generate an AP an AP Unit 3 – Neurophysiology and neural communication 3. NEURAL COMMUNICATION: SYNAPSES 3.4. Signal integration. Unit 3 – Neurophysiology and neural communication 3. NEURAL COMMUNICATION: SYNAPSES 3.4. Signal integration. Summation mechanisms can modulate and diversify the responses: Presynaptic inhibition Postsynaptic inhibition Unit 3 – Neurophysiology and neural communication 3. NEURAL COMMUNICATION: SYNAPSES 3.4. Signal integration: Neural circuits. Lineal circuits: Chain‐like. A single neuron synapses with another one, and this one with another one. But a presynaptic neuron can contact with several postsynaptic neurons. Converging circuits: Many incoming fibers send impulses to the same neuron (spatial summation). Goal: integration of information from different areas (sensory information coming into the spinal cord). Diverging circuits: One incoming fiber triggers responses in increasing numbers of neurons. Goal: Amplify the signal (motor control / neurons of the motor cortex). Unit 3 – Neurophysiology and neural communication 4. NEUROTRANSMITTERS 4. NEUROTRANSMITTERS Unit 3 – Neurophysiology and neural communication 4. NEUROTRANSMITTERS CLASSICAL NT Ester Amino acids Amines Acetylcholin Glutamate Dopamine (DA) e GABA Noradrenaline/ Glycine norepinephrine Adrenalin/ epinephrine Histamine Serotonin (5-HT) Non-classical Purines Peptides Endocannabinoids NT ATP / UTP Neurohormones Anandamide Adenosine Opiates 2-arachidonoylglycerol Neuropeptides Gases Growth Factors Nitric oxide Neurotrophins (NO) Neurokines Fibroblast GF................ Unit 3 – Neurophysiology and neural communication 4. NEUROTRANSMITTERS CLASSICAL NEUROTRANSMITTERS Acetilcholine (Ach): NT from the neuromuscular junction, synthesized by all the neurons of the spinal cord. Specific roles in both CNS and PNS. Catecholamines (dopamine, adrenaline, noradrenaline): Synthesized in áreas of the NS that participate in movement, mood, atention and visceral function. ‐ They can act as neurohormones and travel in the blood flow. Serotonin: Widely distributed in the CNS, participates in processes such as the sleep‐wake cycle, emotions, satiety, circadian rhytms. Histamine: Produced by the hypothalamus and distributed to all the CNS. Participates in attention, analgesia, satiety and also as neuromudulator. Aminoacids: participate in most of the synapses within the CNS. Glutamate (Glu): excitatory aminoacid (EPPs) GABA and Glycine (Gly): inhibitory aminoacids (IPPs) Unit 3 – Neurophysiology and neural communication