Unit 1. Origin and Evolution of Cells PDF
Document Details
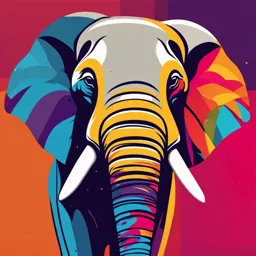
Uploaded by FinestLucchesiite1012
Escuela de Estudios Internacionales UCV
Tags
Summary
This document provides a summary of the origin and evolution of cells, discussing cellular biology and molecular biology. It explores different aspects of cell types, including prokaryotic and eukaryotic cells. It also touches upon the historical and evolutionary context, including important milestones in cell research.
Full Transcript
Unit 1. Overview of the cell and cell research. SECTION I: INTRODUCTION. Cell Biology Biology: science dedicated to the study of the composition, development, functioning, links and distribution of living things. Cell: related to cells (fundamental unit of living beings, which can reproduce ind...
Unit 1. Overview of the cell and cell research. SECTION I: INTRODUCTION. Cell Biology Biology: science dedicated to the study of the composition, development, functioning, links and distribution of living things. Cell: related to cells (fundamental unit of living beings, which can reproduce independently). Cell biology: discipline that specializes in the analysis of cells. It is focused on the structure, function, components, interactions, and properties of these microscopic units. It draws on information related to genetics, biochemistry, and immunology, among other areas of knowledge. UNIT 1 2 Cell biology is responsible for the study of cells with regard to the properties, structure, functions, organelles they contain, their interaction with the environment and their life cycle. Molecular biology aims to study the processes that develop in living beings from a molecular point of view. It tries to explain the phenomena of life from its macromolecular properties. Two macromolecules in particular are his object of study: nucleic acids and proteins. Molecular biology is mainly concerned with the understanding of the interactions of the different systems of the cell, including those of DNA with RNA, protein synthesis, metabolism, and how all these interactions are regulated to achieve correct functioning of the cell. The difference between molecular biology and cell biology lies in the focus of their study. Cell biology focuses on understanding how cellular systems work, how these cells are regulated, and understanding how their structures work. Molecular biology specifically studies the functions of the molecular structure, especially in relation to genetic material (DNA and RNA). UNIT 1 3 INDEX 1.1. Origin and evolution of cells 1.2. Cells as experimental models 1.3. Cell biology instruments UNIT 1 4 1.1. Origin and evolution of cells The cell Cell types Development of multicellular organisms UNIT 1 5 The cell (from Latin cella, meaning "small room") is the basic structural, functional, and biological unit of all known organisms. The cell is the minimum unit of an organism capable of acting autonomously. Hooke's drawing of cells in cork Robert Hooke (1635-1703) (Micrographia, 1665) UNIT 1 6 Antoni van Leeuwenhoek (1632-1723) made very simple but very precise microscopes with which he was able to observe some cells such as protozoa and blood cells, sperm and bacteria, which he called “animalcules”. Drawings of bacteria and protozoa observed by Leeuwenhoek UNIT 1 7 Cell theory Many debates about cells began amongst scientists. Most of these debates involved the nature of cellular regeneration, and the idea of 1- All living organisms are composed cells as a fundamental unit of life. of one or more cells. Cell theory was eventually formulated in 1839. 2- The cell is the basic unit of This is usually credited to Matthias Schleiden and structure and organization in Theodor Schwann. However, many other scientists organisms. like Rudolf Virchow contributed to the theory. 3- Cells arise from pre-existing cells. It was an important step in the movement away 4- Heredity information (DNA) is from spontaneous generation. passed on from cell to cell. UNIT 1 8 There are two main cell types, initially defined according to whether or not they contain a nucleus: Prokaryotic cells. Derived from the Greek "before the nucleus", they comprise bacteria and archaebacteria, they are small cells with a simple structure. In addition to the absence of a nucleus, their genomes are less complex and do not contain cytoplasmic organelles or cytoskeleton. Eukaryotic cells. Derived from the Greek "true nucleus", it is made up of all other living organisms, including protozoa, plants, fungi and animals. They are much bigger. UNIT 1 9 UNIT 1 11 The first cell Life emerged at least 3.8 billion years ago, roughly 750 million years after the earth originated. Biology is a historical science, since the forms and structures of the living world that we know today are the result of billions of years of evolution. Spontaneous formation of organic molecules Stanley Miller (1930-2007), 1953 (Fellow at the University of Chicago) The water vapor was expelled into an atmosphere consisting of H2, 13 CH4, and NH3, in which sparks of electricity were discharged. Analysis of the reaction products revealed the formation of several organic molecules, including the amino acids alanine, aspartic acid, glutamic acid, and glycine. UNIT 1 13 The next step in evolution was the formation of macromolecules. The monomeric building blocks that make up macromolecules have been shown to spontaneously polymerize under plausible prebiotic conditions. Ex: heating dry mixtures of amino acids results in their polymerization to form polypeptides. But the fundamental characteristic of the macromolecule from which life arose must have the ability to replicate itself. Only a macromolecule capable of directing the synthesis of new copies of itself would be able to reproduce and evolve. Only nucleic acids are capable of directing their replication. UNIT 1 14 RNA self-replication The complementary pairs between nucleotides [Adenine (A) with Uracil (U) and Guanine (G) with Cytosine (C)] allow an RNA strand to serve as a template for the synthesis of a new strand with the complementary sequence. RNA is the only one capable of catalyzing its own replication. UNIT 1 15 CENTRAL DOGMA OF MOLECULAR BIOLOGY UNIT 1 16 GENETIC CODE UNIT 1 17 Coating the self-replicating RNA with a phospholipid membrane. The first cell is believed to have arisen from the coating of self-replicating RNA and its associated molecules by a membrane composed of phospholipids. Each phospholipid molecule has two long hydrophobic tails, attached to a hydrophilic group. The hydrophobic tails are embedded in the lipid bilayer; the hydrophilic heads are exposed to water on both sides of the membrane. The envelope of the self-replicating RNA and the molecules associated with a phospholipid membrane have remained as a unit, capable of reproducing itself and evolving. Protein synthesis from RNA could evolve. The first cell would consist of a self-replicating RNA and its encoded proteins. UNIT 1 18 Evolution of metabolism Generation of metabolic energy. Glycolysis is the anaerobic breakdown of glucose into lactic acid. Photosynthesis uses the energy from the sun to drive the synthesis of glucose from CO2 and H2O, releasing O2 as a product. This O2 is used by oxidative metabolism. In oxidative metabolism, glucose breaks down into CO2 and H2O, releasing much more energy than that obtained from glycolysis. The development of these metabolic processes, particularly the use of H2O in photosynthesis, changed the Earth's atmosphere, altering the course of evolution. UNIT 1 19 Consequence of the release of O2 in photosynthesis, which Post photosynthesis changed the environment in which cells evolved Development of oxidative metabolism The increase in O2 was an advantage for Prior to photosynthesis organisms capable of using it in energy generation reactions UNIT 1 20 Current prokaryotes Two main types: archaebacteria and bacteria. Shapes: spherical, rod-shaped or spiral. Diameter: 1 - 10 mm. DNA content: 0,6 - 5 million bp. Components: cell wall (polysaccharides and peptides); plasma membrane (phospholipids and proteins); cytoplasm (ribosomes) and nucleoid (DNA, without nuclear membrane). Cyanobacteria E. coli E. coli UNIT 1 21 Eukaryotic cells UNIT 1 22 UNIT 1 23 UNIT 1 24 Evolution of cells (hypothesis) Today's cells evolved from a common prokaryotic ancestor to three long lines of descent, giving rise to archaebacteria and bacteria. Eukaryotic cells may have arisen by the endosymbiotic association of an aerobic eubacterium with an archaea, leading to the development of mitochondria in addition to the formation of the eukaryotic genome with genes derived from both eubacteria and archaebacteria. Chloroplasts subsequently evolved as a result of the endosymbiotic association of a cyanobacterium with the ancestor of plants. UNIT 1 25 Development of multicellular organisms Saccharomyces cerevisiae Yeasts are the simplest eukaryotes UNIT 1 26 Other single-celled eukaryotes are much more complex cells. Paramecium Chlamydomonas Ciliated protozoa. Large and complex cells (up to Green algae. They contain chloroplasts and are 350 µm). Specialized in the movement and capable of photosynthesis. ingestion of bacteria and yeasts. They have almost twice as many genes as human cells (39500). UNIT 1 27 The evolution of multicellular organisms from single-celled eukaryotes is believed to have taken place 1000-2000 million years ago. Algae, for example, consist of unicellular and multicellular species. Multicellular green algae (Volvox) Volvox algae is made up of cells of 2 types: about 16 large germ cells and 2000 somatic cells immersed in a gelatinous matrix. Increased cell specialization and division of labor in the cells of simple multicellular organisms later led to the diversity and complexity of the multiple cell types that make up today's plants and animals. UNIT 1 28 Animal cells Animal cells are considerably more diverse than those of plants. Epithelial tissue Connective tissue Blood Nervous tissue Muscle tissue UNIT 1 29 Epithelial cells form sheets that cover the surface of the body and delimit the internal organs. There are many types of epithelial cells, each specialized for a specific function, including protection (the skin), absorption (cells of the small intestine), and secretion (cells of the salivary gland). UNIT 1 30 Connective tissue includes bone, cartilage, and adipose tissue, each of which is made up of different types of cells (osteoblasts, chondrocytes, and adipocytes, respectively). The loose connective tissue that delimits with the epithelial layers and fills the spaces between the organs and tissues of the body is formed by another type of cells, fibroblasts. osteoblasts chondrocytes adipocytes fibroblasts UNIT 1 31 The blood contains different types of cells, which function in the transport of oxygen (red blood cells or erythrocytes), inflammatory reactions (granulocytes, monocytes and macrophages) and immune responses (lymphocytes). UNIT 1 32 Nervous tissue is made up of nerve cells, or neurons, that are highly specialized in transmitting signals throughout the body. Various types of sensory cells, such as those in the eye or the ear, are even more specialized in receiving signals from the environment. UNIT 1 33 Muscle cells are responsible for the production of force and movement. Smooth muscle Skeletal muscle Cardiac muscle It is found in the walls of the hollow Composed of long, cylindrical They are part of the wall of the heart. viscera and in most of the blood multinucleated cells that contract to They are elongated and branched cells, vessels. Its cells are spindle-shaped facilitate movement of the body and its with a central nucleus. Most of the and have no striations or tubule parts. They have a large number of cytoplasm is occupied by longitudinally system. mitochondria. Contractile proteins are arranged myofibrils with the same regularly arranged in dark (mainly striated pattern as skeletal muscle. myosin) or light (actin) bands. UNIT 1 34 INDEX 1.1. Origin and evolution of cells 1.2. Cells as experimental models 1.3. Cell biology instruments 35 1.2. Cells as experimental models Unicellular models Multicellular models Escherichia coli Arabidopsis thaliana Yeast Caenorhabditis elegans Drosophila melanogaster Danio rerio Mus musculus 36 Model organisms: species used in research as models of other living beings All cells descend from a common ancestor, whose fundamental properties have been conserved throughout evolution. The knowledge derived from the study of one organism contributes to the study of others, including the human being. Different types of cells and organisms as experimental models to study various aspects of molecular and cellular biology. Not all organisms are as easy to study in the laboratory. Some have certain properties that facilitate this: they grow easily in simple media, they divide quickly, some multicellular organisms are transparent, the complete genomic sequence is available, etc. 37 THE CHOICE OF MODEL ORGANISMS Although, in principle, any living being can be a model organism, in practice, creating a new laboratory model is quite a difficult task. You have to find the right organism to study a scientific problem, then see if it is suitable for the laboratory (culture media) and develop the appropriate techniques for its study. Finally, the consolidation of a new model organism also has to do with the creation of a community of researchers that shares the knowledge generated and works cooperatively. 38 A rod-shaped bacterium that usually inhabits the intestines of humans and other vertebrates. Escherichia coli Particularly useful due to its simplicity and ease of cultivation in the laboratory on simple nutrient media. Its genetic information is contained in a circular double-stranded DNA molecule of approximately 4.6 million base pairs and synthesizes 4,300 different types of proteins. The small size of its genome is an advantage for genetic studies, including genetic manipulation. Most of our knowledge regarding the fundamental mechanisms of life (DNA replication, the genetic code, gene expression, and protein synthesis) comes from studies in E. coli. 39 The small size of the E. coli genome provides clear advantages for genetic analysis. 40 Scanning electron micrograph of E. coli, grown in culture and adhered to a slide. Under optimal culture conditions, E. coli divides every 20 min. Rapid growth medium: glucose, salts, and various organic compounds, such as amino acids, vitamins, and nucleic acid precursors. Slow growing medium: salts, a nitrogen source (such as ammonia), and a carbon and energy E. coli colonies growing on the source (such as glucose). surface of an agar medium. 41 E. coli is a mesophilic bacterium, its optimum development is around the body temperature of warm-blooded animals (35-43 ºC). The growth limit temperature is around 7 ºC. E. coli is sensitive to temperatures above 70 ºC (role of pasteurization). E. coli can live without oxygen (although it can sometimes use it) and gets energy from the fermentation of sugars. Bacteria that can do without oxygen or use it depending on environmental conditions are said to have facultative anaerobic metabolism. PH can influence the growth of E. coli. The optimal development conditions for this parameter is 7.2 (scale 0-14). The development of E. coli stops at extreme pHs (below 3.8, or above 9.5) 42 They are the simplest eukaryotes. They divide every 2 h and can easily grow into colonies Yeast from a single cell. They can be used in a variety of genetic manipulations. Crucial model for the study of many of the fundamental aspects of eukaryotic cell biology (DNA replication, transcription, RNA processing, protein assembly, regulation of cell division...). Saccharomyces cerevisiae Used for making bread and beer. Saccharomyces cerevisiae and Schizosaccharomyces pombe. They can be divided by budding and fission. Between 2001 and 2013, four Nobel Prizes have been awarded for discoveries in yeast research. Saccharomyces pombe 43 Saccharomyces cerevisiae First eukaryotic organism to be sequenced, it is currently the best known eukaryotic genome. Complete sequencing of the S. cerevisiae genome was completed in 1996, after four years of a project led by the European Union and the participation of more than one hundred laboratories from around the world. 12 million bp of DNA, about 6000 genes. 16 linear chromosomes. Its cytoplasm contains a cytoskeleton and subcellular organelles that include mitochondria but not chloroplasts. In 2002, the genome of another yeast, Schizosaccharomyces pombe, also widely used, was sequenced. 44 Fission. Symmetric form of asexual reproduction: It consists of the duplication of DNA, followed by the division of the cytoplasm (cytokinesis), giving rise to two daughter cells. Budding. Asymmetric form of asexual reproduction: From a cell a protrusion is created that grows and ends up giving rise to another cell, smaller (at first) than the initial cell. 45 46 Humanized yeast A fundamental aspect of using yeast as a model is the possibility of "humanizing" it. Human genes are introduced into yeast or its own genes are replaced by their human orthologs, in order to study their functioning. In the pharmaceutical industry, use is made of yeast strains that express human genes for the design of new drugs that interact with the product of said genes (an inhibitor of an enzyme or a ligand for a receptor). Being able to analyze the physiological effects on a microorganism such as yeast greatly facilitates the early stages of the drug development process. 47 The Nobel Prize in Physiology or Medicine 2001 was awarded jointly to Leland H. Hartwell, Tim Hunt and Sir Paul M. Nurse "for their discoveries of key regulators of the cell cycle." 48 Vesicles trafficking "for their discoveries of machinery regulating vesicle traffic, a major transport system in our cells.“ 49 Caenorhabditis elegans Nematodes, commonly known as round worms or cylindrical worms, have constituted, due to their characteristics, a good model for the study of multicellular organisms. They are essentially aquatic organisms, although they also proliferate in terrestrial environments. The main nematode model is Caenorhabditis elegans. Worm just over a millimeter in length. It has been used to study the genetics of animal development and Caenorhabditis elegans cell differentiation and the nervous system. Lately, he is also making contributions to the knowledge of the causes of aging, cell death and the structure of the genome. 50 It is a multicellular organism in the shape of an elongated tube that thins at the ends. Simple multicellular organism: 959 somatic cells, and 1000 to 2000 germ cells. It can be easily reproduced and subjected to genetic manipulation in the laboratory. The whole body is covered by a fine outer cuticle. Cells are organized into fairly simple organs and systems. It has a digestive system made up of a stoma (or mouth), pharynx, and intestine. It also has sexual organs (gonads) and a rudimentary nervous system. Caenorhabditis elegans 51 Adults can present two slightly different sexual forms: hermaphrodism, in which individuals present the sexual organs of both sexes, and the male one. In the first case, which are the majority, they have oviducts, ovaries and a cavity to store sperm. A small percentage of individuals become males, which have a copulatory tail. Reproduction between males and hermaphrodites favors greater genetic variability in the population. In the absence of males, hermaphrodites self-fertilize. Very fast life cycle. From when the egg is produced until it reaches sexual maturity, between three and five days pass; the adult usually lives between two and three weeks and lays 200-300 eggs. Caenorhabditis elegans 52 First multicellular organism to have its genome sequenced (1998). The C. elegans genome is approximately 100 million (108) base pairs long and consists of six chromosomes and one mitochondrial genome. The genome contains an estimated 19,000 protein-coding genes. 36% of the genes of C. elegans have human homologues. 53 He proposed the use of C. elegans as a model organism for research primarily on neural development in animals. The transparency of C. elegans facilitates the study of cell differentiation and other developmental processes in the intact organism. It could be easily grown on Petri dishes on agar, was small enough in size to allow up to a hundred worms per dish and could be observed under an electron microscope. Their unique breeding system was ideal for easily controllable genetic studies that could isolate hermaphrodites to make pure crosses or create new genetic material by crossing with males. 54 In its short life as a model organism, C. elegans has participated in the obtaining of 3 Nobel prizes: Sydney Brenner, John Sulston and Robert Horvitz, who studied apoptosis. Additionally, some work with the worm has pioneered the development of now universal genetic tools: o The RNAi (RNA interference) technique was first described in worm. Mechanism of regulation of genes "for their discoveries concerning genetic regulation of that used as a technique for the functional analysis of organ development and programmed cell death" the genome allows to introduce well-defined sequences of double-stranded RNA into the worm to silence virtually any gene. o The technique based on the Green Fluorescent Protein (GFP). The transparency of C. elegans allowed its use for the in vivo monitoring of certain biological processes. 55 Drosophila melanogaster It can be found practically anywhere on the planet. It feeds on the yeast colonies that grow on top of apples, grapes, bananas, and other sweet fruits. Its biological cycle includes a complete metamorphosis. Development takes place inside the egg, once fertilized, and gives rise to a larva that goes through three successive larval stages and two molts until it reaches its final size. Then the pupal stage occurs, during which metamorphosis takes place: most of the larval cells are destroyed and adult tissues are formed. After emerging, females can lay eggs from the second day; they Drosophila melanogaster can deposit half a thousand eggs in the next ten days. (Fruit fly) 56 1901. William E. Castle (1867-1962), introduces it to Harvard University (USA). A few years later, Thomas H. Morgan (1866-1945) created the fly room at Columbia University in New York and it was then that this species became a model organism for experimentation. Starting in 1910, Morgan's group found the first mutants of clearly defined and heritable characters (eye color, wing shape, etc.), such as the famous white-eyed “white mutants”, and their suitability for being used in genetic studies. Its short life cycle (10 to 15 days) with the generation of numerous families of individuals made it possible to study the appearance and transmission of mutations in successive generations subjected to different environmental conditions (temperature, humidity). By the 1930s, Morgan's team had elucidated the basic principles of modern genetics. From controlled crosses between more than one hundred types of mutant flies, they discovered that the characters are found on chromosomes, are inherited from generation to generation and located their position on the chromosomes. 57 "for his discoveries concerning the role played by the chromosome in heredity." By conducting statistical studies of the way genetic traits are passed on in fruit flies, Thomas Hunt Morgan confirmed that genes are stored in chromosomes inside cell nuclei. He came to understand that genes are organized in a long row inside chromosomes and how traits related to each other correspond to genes that lie close to one another on the chromosomes. He also discovered the "crossover" phenomenon, in which parts of different chromosomes can trade places with one another. 58 The genome of the fruit fly was completely sequenced in March 2000. It contains 180 million base pairs that house around 14,000 genes. Its sequence is publicly accessible, and researchers can look for matches between fly genes and genes involved in certain human diseases. Extensive genetic analysis in Drosophila has uncovered many of the genes that control development and differentiation in animals. The era of genomics, therefore, has opened a new context for research on the fly that links it to biomedical research. 59 Arabidopsis thaliana Model for the study of Molecular Biology in plants. It grows indoors in large numbers. It has no commercial interest, considered a ‘weed’. Produces thousands of shoots per plant in 8-10 weeks. The research covers a wide range of biological processes such as germination, flowering or the response to different types of stress. Similarities and differences in animal and plant development. Arabidopsis thaliana 60 It has a genome of about 125 million base pairs; and its complete sequence is known (year 2000). 25,498 genes have been identified, which are duplicated (only 15,000 genes are different). Complexity similar to C. elegans and D. melanogaster. About 30% of their genes are similar to human genes. Easy to grow in the laboratory. Availability of methods for genetic manipulation. Applications: in agriculture (improvement of species, creation of variants resistant to pests); in medicine (understanding the keys to aging; and understanding / deciphering the causes of difficult-to-understand disorders. 61 Danio rerio The zebrafish (Danio rerio) is a small and active fish and very common in aquariums. Its natural habitat is more or less calm waters, sometimes stagnant, of central Asia, particularly of the region of the Ganges in India. Danio rerio Adult individuals are usually between 3 and 5 cm long and 1 cm (Zebrafisk) wide depending on environmental conditions. It has an elongated shape with a dorsal fin. On the sides it has between 5 and 9 bluish bands that overlap the background color, which is golden in males and silver in females. This striped look has earned it the popular name of zebrafish. The ventral area is whitish and pink. 62 Easy to maintain, resistant to variations in environmental conditions and can coexist with many species. Omnivorous animal that feeds on mosquito larvae and other species of insects (zooplankton) and also on microscopic algae (phytoplankton). Oviparous species. The females can lay between 200 and 300 eggs. The embryonic development of all organs and tissues occurs in just 24 hours. Embryos develop outside of the mother and are transparent, so it is possible to observe all the phases that occur during embryonic development. Generation time of 3-4 months. 63 George Streinsinger (1927-1984). In the 1970s, from the University of Oregon, he proposed the use of this fish to study certain complex processes in biology such as development and the nervous system. George Streinsinger Lateral view of an embryo of a transgenic zebrafish line with GFP expression (green) in the brain and lateral line, and RFP expression (red) in the muscles. 64 Unlike mammals, zebrafish have the ability to regenerate some tissues, such as nervous tissue, heart tissue, the retina or the ear. Investigating the mechanisms that act in the regenerative process unfolds new opportunities for the repair of injured tissues in humans. The zebrafish is excellent for the development of genomic studies of relevance to human health: studying the function of genes involved in processes that take place during the early development of all vertebrates, including humans; as well as studies of gene regulation "in vivo". In 2001, the zebrafish genome began to be sequenced. In 2002 the first draft was made public and since then they have been updated several times until today 85-90% of the genome is complete. It is estimated that it contains 1.7 billion base pairs that correspond to around 14,000 genes, of which it shares about 80% with humans. The genomic information of this species is gathered on different platforms, such as the Zebra Fish Information Network (ZFIN) database. 65 Mus musculus The domestic mouse, known by the scientific name Mus musculus, is a small rodent mammal present in almost all countries. It usually haunts urban environments, although it can also be found inhabiting meadows and forests. The mouse has become the quintessential laboratory animal in life science research. Small in size, it does not usually exceed 35 grams in weight. The tail is usually between 7 and 10 centimeters, about the length of the head and body together. The females may be slightly larger than the males. Gestation usually lasts about 20 days and the average number of offspring is seven embryos per female. 66 It is the laboratory organism par excellence. It has been said of him that he is a pocket human being. A great variety of human diseases reproduce in this rodent, toxicity tests are carried out and therapies are tried. This vast field of research was possible especially from the 1980s with the construction of the first transgenic mice, which incorporate a gene that is not specific to the species, and of knock-out mice in which different genes are deactivated. In 2002 the complete sequence of the mouse genome was published. It measures 2.6 billion base pairs (Mb). It contains about 30,000 genes, and 99% of these genes have their human counterpart. 67 Most of the research done on the mouse in the fields of neuroscience, pharmacology, and physiology, which is then translated into results for humans, is done only with males. One of the reasons males are used is so that the effects of the sex hormones (they reach puberty in 5 or 6 weeks) do not interfere with the results. However, there are many aspects that, such as anxiety or pain, present great differences between the sexes. For this reason, lately the need to include both sexes in research and pay attention to differences has been claimed. In this way, the results will be more realistic and treatments and drugs directed at humans can be fine-tuned. 68 To know more: http://seresmodelicos.csic.es/ https://www.ciudadciencia.es/exposiciones/exposicion_seresmodelicos/ 69 70 Kim J, Koo BK, Knoblich JA. Nat Rev Mol Cell Biol. 2020;21(10):571-584. 71 72 Heydari Z, Moeinvaziri F, Agarwal T, et al. Organoids: a novel modality in disease modeling [published online ahead of print, 2021 Aug 9]. Biodes Manuf. 2021;1-28. 73 Summary of generated human organoid disease models Summary Applications of model organisms Unicellular E. coli: basic molecular processes; genetic manipulation Due to their genetic simplicity and ease of study, bacteria such as E. coli are particularly useful for investigating fundamentals of biochemistry and molecular biology. Yeast: cell cycle and intracellular trafficking As the simplest eukaryotic cells, yeasts are an important model for the study of various aspects of eukaryotic cell biology. 75 Multicellular A. thaliana: in agriculture (improvement of species, creation of variants resistant to pests), studies of the molecular biology of plants and their development. C. elegans: developmental genetics and the animal nervous system; causes of aging, of cell death. D. melanogaster: animal tissue development and differentiation, cancer model. D. rerio: development and the nervous system in vertebrates, identification of new drugs, tissue repair. M. musculus: neuroscience, pharmacology and physiology; transgenic, knock-outs, xenografts. Genetic analysis in mammals. 76 INDEX 1.1. Origin and evolution of cells 1.2. Cells as experimental models 1.3. Cell biology instruments 77 1.3. Cell Biology Instruments (a) Optical microscopy Electron microscopy Super-Resolution Microscopy 78 Light microscope Transmission electron microscope 79 Magnification: the factor by which an image appears to be enlarged. o Light microscope: 1000 X o Electron microscope: 150.000-200.000 X Resolving power: ability of the objective lens to distinguish as separate and different two points very close to each other. o Human eye: 0,1 mm. o Light microscope: 0,2 mm. o Transmission electron microscope: 1-2 nm (10-20 ángstrom (Å)) 80 82 Frog egg (naked eye) 83 Epithelial tissue (Optical microscopy) 84 Ribosome Transcription machinery (electron microscopy) Polypeptide chain mRNA molecule 85 Conventional light microscopy The conventional optical microscope is made up of three systems: Mechanical: foot (base), column (arm), stage (sample holder) and tube (head). Optical: Objectives and eyepieces Lighting: spotlight, condenser and diaphragm. Magnification and resolution Magnification: About 1000 times Most cells can be seen Resolution: 0.2 mm It does not allow to observe small details of the cellular structure = wavelength Resolution = NA NA = Numerical Aperture visible light = 0,5 mm NA = n * sen a n = refractive index of the medium (1,0 for air. Max. 1,4 for immersion oil) a = the maximal half-angle of the cone of light that can enter Resolution the lens limit = = 0,22 mm amax = 90 ͦ 1,4 sen amax = 1 Types of optical microscopy Light field microscope Phase contrast microscope Differential interference-contrast microscope Fluorescence microscope Confocal microscope Multiphoton microscope Light field microscope The light passes directly through the cell. Ability to distinguish different cell parts depends on the contrast obtained from the absorption of visible light by cellular components. Fixation: Stabilize and preserve cell structures (alcohol, acetic acid, formaldehyde...) Staining: Highlight Contrast Light field micrograph of stained tissue. Unsuitable techniques for observing Section of a benign kidney tumor. living cells. Phase contrast microscope Allows us to view live samples without the need for staining techniques. It manipulates the light in such a way that it is possible to increase the contrast of the observed sample, allowing to observe structures that are invisible through a conventional microscope. The phase contrast microscope works by increasing the contrast of the phases of the waves reaching the objective. Small phase differences turn into intensity changes. Phase: measurement of the wave's position relative to a reference point. Yeast observed in the phase contrast microscope Differential Interference Contrast (DIC) Nomarski interference contrast (NIC) or Nomarski microscopy Similar to phase contrast, DIC microscopy is a contrast- enhancing technique. DIC uses polarized light and prisms to convert phase delays into intensity changes (contrast). It is useful for rendering contrast in transparent samples and gives brilliant pseudo-3D relief shading images. DIC is used to image live, unstained biological samples. High sensitivity to thin cellular material. Although DIC images look very appealing, the pseudo-3D effect might be misleading in some cases, making it seem that the cells have structures that they do not have. As an example, areas inside a living cell with a different refractive index, like vacuoles and chromatin, appear as DIC microscopy of Sacharomyces bumps, which is actually an optical impression. cerevisiae. Light field microscope (A) Phase contrast microscope (B) Differential interference- contrast microscope (C) Microscopic observation of living cells. Photomicrographs of human buccal cells. Fluorescence Microscopy Fluorescence microscopy is based on the property of fluorescent substances to absorb light from a certain , and emit light from another . To see structures that have been selectively labeled with fluorescent substances (fluorochromes) and for the study of the intracellular distribution of molecules in living or fixed cells. Almost any celular component can be “stained” and thereby specifically imaged. - Nucleic acids. Specific genes or RNA transcripts can be detected by hybridization with complementary probes labeled with fluorescent markers. - Proteins. They can be detected using labeled antibodies that specifically recognize those proteins that we want to study, or by fusing with fluorescent proteins (such as GFP). Widefield Fluorescence Microscopy The light passes through the excitation filter to select the light from a (eg. blue) that excites the dye. Then a dichroic mirror deflects the excited light towards the sample. The light emitted by the sample (e.g green) passes through the mirror and a barrier filter that selects the emitted by the fluorescent dye. Fluorescent micrograph of a newt lung cell in which the DNA is stained blue and the microtubules in the cytoplasm green. Fluorescent dyes can be used to stain specific cell components, such as acridine orange, which selectively stains the nucleus. https://www.thermofisher.com/es/es/home/life-science/cell-analysis/cell-structure.html A culture of BPAE (Bovine Pulmonary Artery Endothelial Cells) cells was stained with MitoTracker Red CMXRos, vividly labeling the intracellular mitochondrial network. The specimen was also labeled for filamentous actin and DNA with Alexa Fluor 488 (green emission) conjugated to phalloidin and DAPI (blue emission), respectively. Green fluorescent protein (GFP) It was isolated from the Aequorea victoria jellyfish. Revolutionary breakthrough in fluorescence microscopy. GFP can be fused to any protein of interest by recombinant DNA technology. To visualize proteins inside living cells. It does not require fixation or staining, unlike the use of antibodies. Cover of the February 11, 1994 issue of the journal Science (from Chalfie M, et al., Green fluorescent protein as a marker for gene expression, Science, 1994. 263. p. 802). It was the beginning of the GFP revolution. The cover image showed green-glowing sensory neurons in C. elegans. FRAP: fluorescence recovery after photobleaching Method to follow the movement of GFP-labeled proteins in living cells. A region of interest in a cell that expresses a GFP- tagged protein is bleached by exposure to high intensity light. Fluorescence recovers over time due to the movement of unbleached GFP-labeled molecules into the bleached region. The rate of fluorescence recovery therefore provides a measure of the rate of protein movement within the cell. FRET: fluorescence resonance energy transfer The two proteins bind to different fluorescent markers, such as two GFP variants (A, B) that absorb and emit light at different , so that the light emitted by the first excites the second. The interaction between two proteins can be detected by illuminating the cell with a light of that excites the first GFP variant and analyzing the of the emitted light. If there is interaction, the light emitted by A will excite B by spatial closeness, resulting in the emission of light at a typical of B. Confocal Microscopy It enables the acquisition of images of increased detail by analyzing the fluorescence of a single plane of the sample. Structures within thicker objects can be conveniently visualized. Instead of illuminating the whole sample at once, laser light is focused onto a defined spot at a specific depth within the sample. This leads to the emission of fluorescent light at exactly this point. A pinhole inside the optical pathway cuts off signals that are out of focus, thus allowing only the fluorescence signals from the illuminated spot to enter the light detector. By scanning the specimen in a raster pattern, images of one single optical plane are created. 3D objects can be visualized by scanning several optical planes and stacking them using a suitable microscopy deconvolution software (z-stack). It is also possible to analyze multicolor immunofluorescence stainings using state-of-the-art confocal microscopes that include several lasers and emission/excitation filters. Multiphoton Microscopy The sample is illuminated with a light of one such that the excitation of the fluorescent dye requires the simultaneous absorption of two or more photons, which only happens at the point of the sample where the laser is focused. Thus, fluorescence is only emitted from the focus plane of the light. Electron microscopy Due to the limited resolution of the light microscope, the analysis of the details of the cell structure has necessitated this much more powerful technique. Developed in 1930. First applied to biological samples in 1940-1950 by Albert Claude, Keith Porter, and George Palade. The electron microscope uses an accelerated electron beam as a 'illumination' source. The of electrons (up to 0.004 nm) is much lower than that of visible light, which allows greater resolution, allowing the observation of cellular organelles. The theoretical maximum resolution is 0.002 nm, but it has not been achieved, as it also depends on the numerical aperture of the objective lens, whose aperture angle is limited to 0.5 degrees (AN = 0.01). Resolution limit = 0.2 nm. In biological samples, the lack of contrast limits the resolution to 1-2 nm. Transmission electron microscopy (TEM) It consists of a cathode ray tube (electron streams in vacuum tubes), approx. 1 m long. At the top is the cathode, which emits a beam of electrons, through a potential difference, towards a perforated anode that is lower. The beam passes through the anode and is directed to the basal part of the column. On its way, the beam is first modified by an electromagnetic coil that acts as a condenser, focusing the electrons towards the sample. The beam then passes through the sample and is then modified by a coil that acts as an objective and another that serves as a projection system. In order to conduct the electrons, a system of This image is obtained on a fluorescent screen. pumps that generate vacuum is necessary, so it is not possible to carry out in vivo studies. In order for the electron beam to pass through the sample, it must be very thin (10-100 nm). The contrast in TEM is achieved by differential absorption of heavy metals in the form of salts, which bind to biological structures. Typical contrast substances: lead citrate, osmium tetroxide, and uranium acetate. Structures that have a higher affinity for these substances partially impede the passage of electrons (the electrons collide with the heavy metal ions and are reflected, so they do not contribute to the final image), so it is seen on the screen as a dark area (electrodense areas). The areas that allow a greater passage of electrons are seen as light areas (electrolucent areas). Scanning electron microscopy (SEM) It is used to view tissue and cell surfaces in 3D at high magnification and resolution. The samples are placed at the base of the microscope. They have been covered with a heavy metal, so the electrons do not pass through them, but rather they will be swept by the electrons in the three directions of space, giving a three-dimensional image. Electrons emitted from the sample surface are collected by a sensor and projected onto a television monitor. The display aspect is metallic and three- dimensional. Pollen grains Blood cells More information: https://ibidi.com/content/cat egory/127-microscopy- techniques 1.3. Cell Biology Instruments (b) Specimen preparation Flow citometry Subcellular separation Growth of animal cells in culture Virus BLOQUE 1: INTRODUCCIÓN. UNIDAD DIDÁCTICA 1 111 Specimen preparation Histological processing consists of four steps: a. Fixation b. Tissue embedding c. Sectioning d. Staining FIXATION To stop the degradation processes that occur after cell death (autolysis). It preserves the structures and chemical components of the cells and gives the sample mechanical consistency (hardness), allowing subsequent manipulations. a) Physical: cryofixation. The tissue is exposed to temperatures below -70ºC, decreasing enzymatic lysis activity and immobilizing cell structures. It is a reversible method, which stops working when the temperature increases. it can cause the formation of ice crystals that damage cell structure. Cryoprotective substances, such as sucrose or glycerol, prevent the formation of these crystals. Very fast fixation method, very useful in the processing of urgent biopsies. b) Chemicals: uses chemical substances that interact with cellular macromolecules, stabilizing them. TISSUE EMBEDDING Objective: to remove the water from the tissues and replace it with a medium that solidifies, allowing subsequent cutting. It provides structural support to the piece, which makes it possible to obtain fine cuts to allow the passage of light or electrons.. dehydration: using increasing concentrations of ethanol solidification: paraffin is used for optical microscopy and resins for EM. Paraffin: mixture of waxes Resins: they are harder than composed of long-chain paraffin, so they allow finer hydrocarbons with a melting cuts. They are liquid point of 50ºC. The sample is substances in monomeric immersed in liquid paraffin form, and polymerize after (contained in a mold) that treatment with heat or UV infiltrates the intra- and light, giving rise to solid extracellular spaces of the polymers of great hardness. tissue. SECTIONING Histological sections are made in a microtome (between 3 and 5 mm, OM) or ultramicrotome (between 10-100 mm, EM). Paraffin sections are collected on glass slides and TEM sections on copper or nickel grids. The sections of the freeze-fixed samples are thicker (6-8 mm minimum), and are made in a cryostat, which maintains the samples at a temperature of -20 ° C during the section. These samples do not require the dehydration step. There are samples that do not require fixing or sectioning steps, but the visualization is done directly. For example, cells in liquid medium (blood smear) or samples of various biological fluids, such as sputum or bronchioalveolar lavage. Extensions are made directly on the slide. STAINING In OM, dyes with different affinity for cell organelles are used, depending on their chemical composition. Examples of basic dyes: are thionin, safranin, toluidine blue, methylene blue, hematoxylin. They have a craving for acidic substances in tissue such as DNA, thus revealing the cell nucleus. Examples of acid dyes are acid fuchsin, quick green, orange G, or eosin. They have a craving for basic substances, especially protein structures located in the cell cytoplasm and also for the collagen of the extracellular matrix. One of the most commonly used stains in histology is hematoxylin-eosin. A basic dye (hematoxylin) and another acid (eosin) are used to stain the acidic and basic structures of the cell differently. In EM, the contrast is performed by means of high molecular weight molecules that enhance the dispersion capacity of the sample (uranium acetate and lead citrate). Sample preparation for light microscopy: https://www.youtube.com/watch?v=4DJm4NLECQs Sample preparation for electron microscopy: https://www.youtube.com/watch?v=Ad5VGbA-_vk IMMUNOHISTOCHEMICAL TECHNIQUES Purpose: to identify the presence of a specific protein in a tissue section by using specific antibodies. Immunohistochemistry is essential in pathological anatomy laboratories, due to its ability to help in the diagnosis of diseases. Example: identification of viral proteins, overexpression of certain oncogenes, etc. Immunohistochemical techniques can be divided into two large groups: Direct: The antibody that recognizes the antigen of interest (primary antibody) is conjugated to a substance that may be detectable under a microscope (an enzyme, a fluorochrome, or a gold particle). Indirect: the primary antibody is not conjugated, but a secondary antibody is used, which recognizes the primary one, and which in turn is conjugated with a substance visible under the microscope. Therefore, the signal will come from the secondary antibody. Indirect methods are the most widely used, since the antibodies used can recognize the Fc fraction of a given species, and use the same antibody for many primary antibodies, regardless of the antigen they recognize. Flow cytometry Flow cytometry is a technique for analyzing the number, size and complexity of a cell suspension. It is also used to quantify the number of cells in certain cell populations specifically labeled with fluorescent substances and to isolate cell populations. Especially useful for the study of normal and pathological populations of blood cells and bone marrow. The cytometer has the following components: ✓ Fluidic sample transport system. ✓ Optical laser illumination system. ✓ Electronic detector, which converts light into digital signal. The cells pass in a very fine capillary so that they pass one by one. As the cells pass, the beam hits them and a beam distortion is produced, which is what is registered by the detector. This system quantifies the number of events (cells) that are impacted by the laser, but it is also capable of analyzing the shape and internal complexity of the cell.. Visible light scatter is measured in two different directions, the forward direction (Forward Scatter or FSC) which can indicate the relative size of the cell and at 90° (Side Scatter or SSC) which indicates the internal complexity or granularity of the cell. COMPLEXITY SIZE neutrophil neutrophils monocytes lymphocyte monocyte lymphocytes Size 125 Subcellular separation To study the chemical composition and function of certain organelles and other subcellular particles, it is convenient to separate them from the rest of the cytoplasmic and nuclear components. The first thing to do is to break the plasma membrane, which will make it possible to obtain a suspension with all the subcellular components. There are various methods of breaking down cells. Most used physical methods: osmotic shock, ultrasound, mechanical grinding. These procedures break down most cell membranes (plasma membrane and endoplasmic reticulum membranes) Enzymatic methods, usually lysozyme is used. When cells have a wall (plant or bacterial cells). DIFFERENTIAL CENTRIFUGATION A typical homogenate or lysate is made up of a suspension of organelles, cellular components and macromolecules that can be separated by centrifugation. An ultracentrifuge processes the samples at high speed (100,000 rpm) to produce forces around 500,000 times greater than gravity. Cellular components settle to the bottom of the centrifuge tube forming a precipitate (sedimentation) to a degree that depends on size and density: larger and heavier structures settle more quickly. Centrifuges are equipment that generate this type of force and have a rotor (where the samples are placed) that rotates at very high speeds. DENSITY GRADIENT CENTRIFUGATION It allows to obtain a higher degree of purification. The organelles are separated by sedimentation as a function of the gradient of a dense substance, such as sucrose or cesium chloride. Speed separations: Particles of different sizes settle down the gradient at different scales, moving as bands. The separated particles can be obtained in individual fractions Equilibrium separations: The gradient is very concentrated. Subcellular components separate regardless of their size and shape, according to their density. The particle does not move when its density is equal to that of the medium (equilibrium position). Centrifugation also allows cells to be separated, for which gradients such as Ficoll, Percol or Nicodenz are used. The separation of Peripheral Blood Mononuclear Cells (PBMC) may be required for certain applications including flow cytometry. Cell cultures The study of cells in a living organism is very difficult. An alternative is to isolate and maintain the cells in the laboratory under conditions that allow their survival and growth, a procedure that is known by the term cell culture. Although the process is technically much more difficult than the culture of bacteria and yeast, a wide variety of animal and plant cells can be cultured and manipulated in vitro. Cultured cells have several advantages over intact organisms for research: the experimental conditions can be better controlled, in many cases a single cell can easily grow into a colony of many identical cells. The resulting strain of cells, which is genetically homogeneous, is called a clone. ANIMAL CULTURES They are started by dispersing a part of tissue in a suspension of its cellular components. The cells are added to a culture dish containing a nutrient medium. Primary cultures: initial cultures of cells established from a culture. The cells grow to cover the surface of the culture dish. They can then be removed from the plate and replenished at low density to form secondary cultures. This process can be repeated many times, although most normal cells cannot grow indefinitely in culture.E. g.: human fibroblasts tolerate 50-100 passages. In contrast, cells that are derived from tumors often proliferate indefinitely in culture and are called immortal cells. Continuous and uniform source of cells that can be manipulated and cultivated indefinitely. In humans it was obtained for the first time in 1951: HeLa cells, obtained from a biopsy of Henrietta Lacks' cervical tumor. Embryonic stem cells. They are established from early embryos. Ability to differentiate into all cell types of the adult organism. Developmental gene function studies. HeLa cells Dr. George Gey, in his cancer research, spent 30 years trying to grow cancer cells in the laboratory. On February 1, 1951, Henrietta Lacks was taken to John Hopkins Hospital. She had a cervical tumor. Cells from part of her tumor were retained in the hospital's cancer unit as Gey had discovered that they could be grown in the laboratory indefinitely. It was what he had sought for so many years. For the first time anything could be tested in living human cells. One of the first uses was the development of the vaccine against the polio virus. At present they continue to be widely used in human cell and molecular biology. One of the most used tools in the field of cell biology. Cells grown in vitro come from a normal or tumor organ or tissue. These cells are kept in culture media of known composition and under controlled oxygen and temperature conditions (usually 37°C, 5% CO2 and 95% O2). The necessary culture medium is complex (salts, glucose, aa and vitamins). It usually also includes serum, a source of polypeptide growth factors necessary for cell division. Identification of specific growth factors for specific cell types eliminates serum necessity. VIRUSES Viruses need a host in which to carry out their life cycle. To grow viruses in the laboratory, it is essential to grow an animal, plant, fungal or bacterial cell culture, depending on what infects the virus. The host cells will enable the virus to increase its numbers. They provide simple systems for studying the functions of cells. Studies with viruses have revealed fundamental aspects of cell biology: molecular genetic mechanisms, RNA genetic potential, discovery of oncogenes...