Unit 1 Bacterial Strategies for Evasion & Study of Few Diseases PDF
Document Details
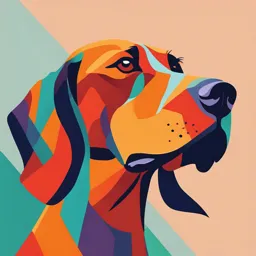
Uploaded by FruitfulHibiscus
Tags
Summary
This document provides an overview of bacterial strategies for evasion and the study of certain diseases. It details pathogenicity islands, bacterial virulence factors (including adherence factors, invasion mechanisms, and toxins), and the infectious process. It also touches upon the role of bacterial enzymes, antiphagocytic factors, and the requirement of iron in bacterial pathogenesis.
Full Transcript
Unit 1. Bacterial Stratergies for Evasion & Study of Few Diseases 1.1.1. Pathogenicity islands. 1.1.2 Bacterial Virulence Factors. 1.1.2.1 Adherence Factors. 1.1.2.2 Invasion of host cells and tissues. 1.1.3 Toxins 1.1.3.1 Exotoxins. 1.1.3.2 Exotoxins associated with diarrhoeal diseases and...
Unit 1. Bacterial Stratergies for Evasion & Study of Few Diseases 1.1.1. Pathogenicity islands. 1.1.2 Bacterial Virulence Factors. 1.1.2.1 Adherence Factors. 1.1.2.2 Invasion of host cells and tissues. 1.1.3 Toxins 1.1.3.1 Exotoxins. 1.1.3.2 Exotoxins associated with diarrhoeal diseases and Food poisoning. 1.1.3.3 LPS of Gram negative Bacteria. 1.1.4 Enzymes. 1.1.4.1 Tissue degrading enzymes. 1.1.4.2 IgA1 proteases. 1.1.5 Antiphagocytic Factors. 1.1.6 Intracellular pathogenicity. 1.1.7 Antigenic Heterogeneity. 1.1.8 The requirement of Iron Analysis of infection and disease through the application of principles such as Koch’s postulates leads to classification of bacteria Pathogens. Opportunistic pathogens. Non-pathogens. Some bacterial species are always considered to be pathogens, and their presence is abnormal; examples include Mycobacterium tuberculosis (tuberculosis) and Yersinia pestis (plague). Other species are commonly part of the normal microbiota of humans (and animals) but also can frequently cause disease. For example, E coli is part of the gastrointestinal microbiota of normal humans but is also a common cause of urinary tract infections, traveler’s diarrhea, and other diseases. Strains of E coli that cause disease are differentiated from non pathogenic by determining (1) Whether they are virulent in animals or in vitro models of infection (2) Whether they have a genetic makeup that is significantly associated with production of disease. The Infectious Process. 1. Entry. 2. Adhering / attaching to the Host cells (usually ephithelial cells.) 3. Bacteria now have established the primary site of infection. 4. Multiplication and spreading. 5. Spreading directly through tissue or via lymphatic system to the bloodstream. 6. Bacterimia : presence of bacteria in the bloodstream. 7. It can be transient or persistent. GENOMICS AND BACTERIAL PATHOGENICITY Bacteria are haploid limit genetic interactions that might change their chromosomes and potentially disrupt their adaptation and survival in specific environmental niches. The Clonal Nature of Bacterial Pathogens Conservation of chromosomal genes in bacteria is that the organisms are clonal Mobile Genetic Elements. Primary mechanisms for exchange of genetic information between bacteria includes: 1. Natural transformation 2. Transmissible mobile genetic elements such as plasmids, transposons, and bacteriophages. Transfer of these mobile genetic elements between members of one species or, less commonly, between species can result in transfer of virulence factors, including antimicrobial resistance genes. 1.1.1. Pathogenicity Islands Large groups of genes that are associated with pathogenicity and are located on the bacterial chromosome are termed pathogenicity islands (PAIs). They are large organized groups of genes, usually 10–200 kb in size. Properties of Pathogenic Islands. (PAIs) 1. They have one or more virulence genes. 2. They are present in the genome of pathogenic members of a species but absent in the nonpathogenic members. 3. They are large organized groups of genes, usually 10-200 Kb in size. 4. They typically have a different guanine plus cytosine (G + C) content than the rest of the bacterial genome. 5. They are commonly associated with tRNA genes. 6. They are often found with parts of the genome associated with mobile genetic elements. 7. They often have genetic instability, and they often represent mosaic structures with components acquired at different times. 1.1.2. BACTERIAL VIRULENCE FACTORS A. Adherence Factors Adherence, which is only one step in the infectious process, is followed by development of microcolonies and subsequent steps in the pathogenesis of infection. 1. Interaction Between Bacteria and tissue cell surface. 2. Bacterial surface molecules such as Pili and Fimbrae. 3. specific ligand-receptor mechanisms. 1. The interactions between bacteria and tissue cell surfaces Factors playing important roles including surface hydrophobicity and net surface charge, binding molecules on bacteria (ligands), and host cell receptor interactions. Bacteria and host cells commonly have net negative surface charges and therefore repulsive electrostatic forces. These forces are overcome by hydrophobic and other more specific interactions between bacteria and host cells. In general, the more hydrophobic the bacterial cell surface, the greater the adherence to the host cell. Different strains of bacteria within a species may vary widely in their hydrophobic surface properties and ability to adhere to host cells. 2. Bacterial surface molecules such as Pili and Fimbrae Bacteria also have specific surface molecules that interact with host cells. Many bacteria have pili, thick rodlike appendages / fimbriae, shorter “hairlike” structures that extend from the bacterial cell surface and help mediate adherence of the bacteria to host cell surfaces. The E.coli that cause diarrheal diseases have pilus (fimbriae)-mediated adherence to intestinal epithelial cells. The type of pili and specific molecular mechanisms of adherence appear to be different depending on the form of the E coli that induce the diarrhea. E.coli causing urinary tract infections have P-pili, which attach to a portion of the P blood group antigen; the minimal recognition structure is the disaccharide α-d-galactopyranosyl-(1–4) β-d-galactopyranoside (GAL–GAL binding adhesion). 3. Specific ligand-receptor mechanisms Group A streptococci (Streptococcus pyogenes) also have hairlike appendages, termed fimbriae, that extend from the cell surface. Lipoteichoic acid, protein F, and M protein are found on the fimbriae. The lipoteichoic acid and protein F cause adherence of the streptococci to buccal epithelial cells; this adherence is mediated by fibronectin, which acts as the host cell receptor molecule. M protein acts as an antiphagocytic molecule and is a major virulence factor. Adherence factor Role played in Virulence. 1. Capsule Helps in adherence Ex. Streptococcus mutans causative agent of dental Caries produce glucan capsule and adheres to smooth tooth surfaces. It also inhibits phagocytosis. 2. Pili Helps in adherence Ex. Neisseria gonorrhaea adheres to epithelial cells of cervix, urethra and conjuctiva. Ecoli , Causative agent of diarrhae adheres to mucosall lining of small intestine by pili 3. M protein Cell wall protein of Streptococcus pyogenes a causative agent of Sore throat acts as adhesin for attachment to epithelial cells of the throat. 4. Techoic acids and Cell wall component of Gram positive bacteria Helps in Adhesion. Lipotechoic acid 5. Lectin A carbohydrate binding protein present in many Gram negative bacteria helps in adhesion. 6. Ligands A low molecular weight substances occurs in cell wall helps in adhesion 7. Slime layer Slime of Pseudomonas plays important role in adhesion 8. Glycocalysx A layer of exopolysaccharide fibres present on the surface of pathogens. What Next After Adherence ? After adherence occurs, conformational changes in the host cell ensure that can lead to cytoskeletal changes allowing organism uptake by the cell. Sometimes changes in the adhesin molecule after attachment may trigger activation of virulence genes that promote invasion or that result in other pathogenic changes 1.1.2.1 Invasion of Host Cells and Tissues Invasion is the term commonly used to describe the entry of bacteria into host cells, implying an active role for the organisms and a passive role for the host cells. When inside the host cell, bacteria may remain enclosed in a vacuole composed of the host cell membrane, or the vacuole membrane may be dissolved and bacteria may be dispersed in the cytoplasm. Some bacteria (eg, Shigella species) multiply within host cells, but other bacteria do not. In many infections, the bacteria produce virulence factors that influence the host cells, causing them to engulf (ingest) the bacteria. The host cells play a very active role in this process. I. Invasion by Shigella. Shigella species adhere to host cells in vitro. Commonly, HeLa cells are used; these undifferentiated unpolarized cells were derived from a cervical carcinoma. The adherence causes actin polymerization in the nearby portion of the HeLa cell, which induces the formation of pseudopods by the HeLa cells and engulfment of the bacteria. Adherence and invasion are mediated at least in part by products of genes located on a large plasmid common to many shigellae. There are multiple proteins, including the invasion plasmid antigens (IpA-D), that contribute to the process. Inside the HeLa cells, the shigellae either are released or escape from the phagocytic vesicle, where they multiply in the cytoplasm. Actin polymerization propels the shigellae within a HeLa cell and from one cell into another. In vivo the shigellae adhere to integrins on the surface of M cells in Peyer’s patches and not to the polarized absorptive cells of the mucosa. M cells normally sample antigens and present them to macrophages in the submucosa. The shigellae are phagocytosed by the M cells and pass through the M cells into the underlying collection of macrophages. Shigellae inside the M cells and macrophages can cause these cells to die by activating the normal cell death process (apoptosis). The shigellae spread to adjacent mucosal cells in a manner similar to the in vitro model by actin polymerization that propels the bacteria. How Actin helps in taking up and spreading of Bacteria. Actin polymerization propels the shigellae II. Invasion by Yersiniae enterocolitica Yersiniae adhere to the host cell membrane and cause it to extrude protoplasmic projections. The bacteria are then engulfed by the host cell with vacuole formation. Invasion is enhanced when the bacteria are grown at 22°C rather than at 37°C. When yersiniae have entered the cell, the vacuolar membrane dissolves and the bacteria are released into the cytoplasm. In vivo, the Yersiniae are thought to adhere to and invade the M cells of Peyer’s patches rather than the polarized absorptive mucosal cells, much like shigellae. II. Invasion by Listeria monocytogenes. L monocytogenes from the environment is ingested in food. Presumably, the bacteria adhere to and invade the intestinal mucosa, reach the bloodstream, and disseminate. The pathogenesis of this process has been studied in vitro. L monocytogenes adheres to and readily invades macrophages and cultured undifferentiated intestinal cells. The listeriae induce engulfment by the host cells. Proteins, called internalins, have a primary role in this process. The engulfment process, movement within a cell and movement between cells, requires actin polymerization to propel the bacteria, as with shigellae. II. Invasion by Legionella pneumophila Legionella pneumophila infects pulmonary macrophages and causes pneumonia. Adherence of the legionellae to the macrophage induces formation of a long, thin pseudopod that then coils around the bacteria, forming a vesicle ( coiling phagocytosis). The vesicle remains intact, phagolysosome fusion is inhibited, and the bacteria multiply within the vesicle. III. Invasion by N. gonorrhoeae N gonorrhoeae uses pili as primary adhesins and Opacity Associated Proteins (Opa) as secondary adhesins to host cells. Certain Opa proteins mediate adherence to polymorphonuclear cells. Some gonococci survive after phagocytosis by these cells. Pili and Opa together enhance the invasion of cells cultured in vitro. In uterine (fallopian) tube organ cultures, the gonococci adhere to the microvilli of nonciliated cells and appear to induce engulfment by these cells. The gonococci multiply intracellularly and migrate to the subepithelial space by an unknown mechanism. 1.1.3 TOXINS Toxins produced by bacteria are generally classified into two groups: Exotoxins and Endotoxins. Exotoxins are proteins that are most often excreted from the cell. Some exotoxins accumulate inside the cell and are either injected directly into the host or are released by cell lysis. Endotoxins are lipid molecules that are components of the bacterial cell membrane. 1.1.3.1 Exotoxins Many gram-positive and gram-negative bacteria produce exotoxins of considerable medical importance. Vaccines have been developed for some of the exotoxin-mediated diseases and continue to be important in the prevention of disease. These vaccines—called toxoids—are made from exotoxins, which are modified so that they are no longer toxic. Toxins have two Subunit A and B B subunits helps in adherence and A subunit have toxicity effect. I. C. diphtheriae exotoxin C. diphtheriae is a gram-positive rod that can grow on the mucous membranes of the upper respiratory tract or in minor skin wounds. Strains of C diphtheriae that carry a lysogenic, temperate corynebacteriophage (β-phage or ω-phage) with the structural gene for the toxin are toxigenic and produce diphtheria toxin and cause diphtheria. Many factors regulate toxin production; when the availability of inorganic iron is the factor limiting the growth rate, then maximal toxin production occurs. The toxin molecule is secreted as a single polypeptide molecule (molecular weight [MW], 62,000). This native toxin is enzymatically degraded into two fragments, A and B, linked together by a disulfide bond. Fragment B (MW, 40,700) binds to specific host cell receptors and facilitates the entry of fragment A (MW, 21,150) into the cytoplasm. Fragment A inhibits peptide chain elongation factor EF-2 by catalyzing a reaction that attaches an adenosine diphosphate–ribosyl group to EF-2, yielding an inactive adenosine diphosphate–ribose–EF-2 complex. Arrest of protein synthesis disrupts normal cellular physiologic functions. Diphtheria toxin is very potent II. C. tetani exotoxin C tetani is an anaerobic gram-positive rod that causes tetanus. C tetani from the environment contaminates wounds, and the spores germinate in the anaerobic environment of the devitalized tissue. Infection often is minor and not clinically apparent. The vegetative forms of C tetani produce the toxin tetanospasmin (MW, 150,000) that is cleaved by a bacterial protease into two peptides (MW, 50,000 and 100,000) linked by a disulfide bond. The toxin initially binds to receptors on the presynaptic membranes of motor neurons. It then migrates by the retrograde axonal transport system to the cell bodies of these neurons to the spinal cord and brainstem. The toxin diffuses to terminals of inhibitory cells, including both glycinergic interneurons and γ-aminobutyric acid (GABA)–secreting neurons from the brainstem. The toxin degrades synaptobrevin, a protein required for docking of neurotransmitter vesicles on the presynaptic membrane. Release of the inhibitory glycine and GABA is blocked, and the motor neurons are not inhibited. Spastic paralysis results. Extremely small amounts of toxin can be lethal for humans. Tetanus is totally preventable in immunologically normal people by immunization with tetanus toxoid. III. C. botulinum exotoxin. C botulinum causes botulism. This anaerobic, gram positive spore-forming organism is found in soil or water and may grow in foods (eg, canned, vacuum packed) if the environment is appropriately anaerobic. An exceedingly potent toxin (the most potent toxin known) is produced. Lethal dose 1.3-2.1 ng/kg It is heat labile and is destroyed by sufficient heating. There are seven distinct serologic types of toxin. Types A, B, E, and F are most commonly associated with human disease. The toxin is very similar to tetanus toxin, with a 150,000 MW protein that is cleaved into 100,000-MW and 50,000-MW proteins linked by a disulfide bond. Botulinum toxin is absorbed from the gut and binds to receptors of presynaptic membranes of motor neurons of the peripheral nervous system and cranial nerves. Proteolysis, by the light chain of botulinum toxin, of target proteins in the neurons inhibits the release of acetylcholine at the synapse, resulting in lack of muscle contraction and flaccid paralysis. IV. C perfringens exotoxin. Spores of C perfringens are introduced into wounds by contamination with soil or feces. In the presence of necrotic tissue (an anaerobic environment), spores germinate, and vegetative cells can produce several different toxins. Many of these are necrotizing and hemolytic and—together with distention of tissue by gas formed from carbohydrates and with blood supply—favor the spread of gas gangrene. The alpha toxin of C perfringens is a lecithinase that damages cell membranes by splitting lecithin to phosphorylcholine and diglyceride. Theta toxin also has a necrotizing effect. Collagenases and DNAses are produced by clostridiae as well. V. S aureus exotoxin. Some S aureus strains growing on mucous membranes (eg, the vagina in association with menstruation) or in wounds, elaborate toxic shock syndrome toxin-1 (TSST-1), which causes toxic shock syndrome The illness is characterized by shock, high fever, and a diffuse red rash that later desquamates; multiple other organ systems are involved as well. TSST-1 is a super antigen and stimulates T-cells to produce large amounts of interleukin-2 (IL-2) and tumor necrosis factor (TNF). The major clinical manifestations of the disease appear to be secondary to the effects of the cytokines. Many of the systemic effects of TSST-1 are similar to those of toxicity caused by lipopolysaccharide. Some strains of group A β-hemolytic streptococci produce pyrogenic exotoxin A that is similar to or the same as streptococcal erythrogenic toxin, which results in scarlet fever. Rapidly progressive soft tissue infection by streptococci that produce the pyrogenic exotoxin A has many clinical manifestations similar to those of staphylococcal toxic shock syndrome. The pyrogenic exotoxin A also is a super antigen that acts in a manner similar to TSST-1. Super Antigen 1.1.3.2 Exotoxins associated with diarrhoeal diseases and Food poisoning. Exotoxins associated with diarrheal diseases are frequently called enterotoxins. We will study two Exotoxins V cholerae & S.aureus Enterotoxin. I. V.cholerae Enterotoxin V cholerae enter the host via contaminated food or drink, and penetrates the intestinal mucosa and attaches to microvilli of the brush border of gut epithelial cells. V cholerae, usually of the serotype O1 (and O139), can produce an enterotoxin with a MW of 84,000. The toxin consists of two subunits—A, which is split into two peptides, A1 and A2 , linked by a disulfide bond, and B. Subunit B has five identical peptides and rapidly binds the toxin to cell membrane ganglioside molecules. Subunit A enters the cell membrane and causes a large increase in adenylate cyclase activity and in the concentration of cAMP. (cyclic Adenosine Mono phosphate). The net effect is rapid secretion of electrolytes into the small bowel lumen, with impairment of sodium and chloride absorption and loss of bicarbonate. Life-threatening massive diarrhea (eg, 20–30 L/day) can occur, and acidosis develops. The deleterious effects of cholera are due to fluid loss and acid–base imbalance; treatment, therefore, is by electrolyte and fluid replacement. II. S.aureus Enterotoxin Some strains of S aureus produce enterotoxins while growing in meat, dairy products, or other foods cases, the food has been recently prepared but not properly refrigerated. There are at least seven distinct types of the staphylococcal enterotoxin. After the preformed toxin is ingested, it is absorbed in the gut, where it stimulates vagus nerve receptors. The stimulus is transmitted to the vomiting center in the central nervous system. Vomiting, often projectile, results within hours. Diarrhea is less frequent. Staphylococcal food poisoning is the most common form of food poisoning. S aureus enterotoxins are super antigens. 1.1.3.2 Endotoxin Lipopolysaccharides The LPS (endotoxin) of gram-negative bacteria are bacterial cell wall components that are often liberated when the bacteria lyse. The substances are heat-stable, have MWs between 3000 and 5000 (lipooligosaccharides, LOS) and several million (lipopolysaccharides) and can be extracted (eg, with phenol-water). Pathophysiological Effect of LPS. 1. LPS in the bloodstream is initially bound to circulating proteins, which then interact with receptors on macrophages neutrophils and other cells of the reticuloendothelial system. 2. Proinflammatory cytokines such as IL-1, IL-6, IL-8, TNF-α, and other cytokines are released, and the complement and coagulation cascades are activated. 3. The following can be observed clinically or experimentally: 4. fever, due to Interleukin-1 5. leukopenia, Decrease in WBC count. 6. hypoglycemia; Rapid Glycolysis leads to decrease in blood glucose level. 7. hypotension Low Blood pressure causes low oxygen delivery to various organs. 8. shock resulting in impaired perfusion of essential organs (eg, brain, heart, kidney); 9. intravascular coagulation; 10. death from massive organ dysfunction. Disseminated intravascular coagulation is a frequent complication of gram-negative bacteremia and can also occur in other infections. LPS activates factor XII (Hageman factor)—the first step of the intrinsic clotting system—and sets into motion the coagulation cascade, which culminates in the conversion of fibrinogen to fibrin. At the same time, plasminogen can be activated by LPS to plasmin (a proteolytic enzyme), which can attack fibrin with the formation of fibrin split products. Reduction in platelet and fibrinogen levels and detection of fibrin split products are evidence of Disseminated intravascular coagulation (DIC). Heparin can sometimes prevent the lesions associated with DIC. This results in Less platelet counts and fibrinogen detection of fibrin split products are evidence of DIC. Disseminated intravascular coagulation 1.1.4. Enzymes. Many species of bacteria produce enzymes that are not intrinsically toxic but do play important roles in the infectious process. A. Tissue-Degrading Enzymes Lecithinase, collagenase, coagulase, Hyaluronidases, streptokinase (fibrinolysin) The lecithinase enzyme contributes to pathogenesis by destroying red blood cells. Lecithinase is an enzyme which catalyzes the breakdown of phospholipids. This enzyme can breakdown the phospholipids in the membranes of red blood cells. C perfringens produces the proteolytic enzyme collagenase, which degrades collagen, the major protein of fibrous connective tissue, and promotes spread of infection in tissue. S aureus produces coagulase, which works in conjunction with blood factors to coagulate plasma. Coagulase contributes to the formation of fibrin walls around staphylococcal lesions, which helps them persist in tissues. Coagulase also causes deposition of fibrin on the surfaces of individual staphylococci, which may help protect them from phagocytosis or from destruction within phagocytic cells. Hyaluronidases are enzymes that hydrolyze hyaluronic acid, a constituent of the ground substance of connective tissue. They are produced by many bacteria (eg, staphylococci, streptococci, and anaerobes) and aid in their spread through tissues. Hyaluronidases are enzymes that hydrolyze hyaluronic acid, a constituent of the ground substance of connective tissue. They are produced by many bacteria (eg, staphylococci, streptococci, and anaerobes) and aid in their spread through tissues. Many hemolytic streptococci produce streptokinase (fibrinolysin), a substance that activates a proteolytic enzyme of plasma. This enzyme is then able to dissolve coagulated plasma and probably aids in the rapid spread of streptococci through tissues. Streptokinase has been used in treatment of acute myocardial infarction to dissolve fibrin clots. Many bacteria produce substances that are cytolysins— that is, they dissolve red blood cells (hemolysins) or kill tissue cells or leukocytes (leukocidins). Streptolysin O, for example, is produced by group A streptococci and is lethal for mice and hemolytic for red blood cells from many animals. Streptolysin O is oxygen labile and can therefore be oxidized and inactivated, but it is reactivated by reducing agents. It is antigenic. The same streptococci also produce oxygen-stable, serum-inducible streptolysin S, which is not antigenic. Clostridia produce various hemolysins, including the lecithinase described earlier. Hemolysins are produced by most strains of S aureus; staphylococci also produce leukocidins. Most gram-negative rods isolated from sites of disease produce hemolysins. For example, whereas E coli strains that cause urinary tract infections typically produce hemolysins, strains that are part of the normal gastrointestinal flora may or may not produce hemolysins B. IgA1 Proteases Immunoglobulin A is the secretory antibody on mucosal surfaces. It has two primary forms, IgA1 and IgA2, that differ near the center, or hinge region of the heavy chains of the molecules. IgA1 has a series of amino acids in the hinge region that are not present in IgA2. Some bacteria that cause disease produce enzymes, IgA1 proteases, that split IgA1 at specific proline–threonine or proline–serine bonds in the hinge region and inactivate its antibody activity. IgA1 protease is an important virulence factor of the pathogens N gonorrhoeae, N meningitidis, H influenzae, and S pneumoniae. Production of IgA1 protease allows pathogens to inactivate the primary antibody found on mucosal surfaces and thereby eliminate protection of the host by the antibody. 1.1.5 Antiphagocytic Factors Many bacterial pathogens are rapidly killed after they are ingested by polymorphonuclear cells or macrophages. Some pathogens evade phagocytosis or leukocyte microbicidal mechanisms by adsorbing normal host components to their surfaces. For example, S aureus has surface protein A, which binds to the Fc portion of IgG. Other pathogens have surface factors that impede phagocytosis (eg, S pneumoniae, N meningitidis) many other bacteria have polysaccharide capsules. S pyogenes (group A streptococci) has M protein. N gonorrhoeae (gonococci) has pili. Most of these antiphagocytic surface structures show much antigenic heterogeneity. For example, there are more than 90 pneumococcal capsular polysaccharide types and more than 150 M protein types of group A streptococci. Antibodies against one type of the antiphagocytic factor (eg, capsular polysaccharide, M protein) protect the host from disease caused by bacteria of that type but not from those with other antigenic types of the same factor. A few bacteria (eg, Capnocytophaga and Bordetella species) produce soluble factors or toxins that inhibit chemotaxis by leukocytes and thus evade phagocytosis by a different mechanism. 1.1.6 Intracellular Pathogenicity Some bacteria (eg, M tuberculosis, Listeria monocytogenes, Brucella species, and Legionella species) live and grow in the hostile environment within polymorphonuclear cells, macrophages, or monocytes. The bacteria accomplish this feat by several mechanisms: They may avoid entry into phagolysosomes and live within the cytosol of the phagocyte; They may prevent phagosome–lysosome fusion and live within the phagosome; They may be resistant to lysosomal enzymes and survive within the phagolysosome. Many bacteria can live within nonphagocytic cells 1.1.7 Antigenic Heterogeneity Antigenic heterogeneity or antigenic alteration refers to the mechanism by which an infectious agent such as a protozoan, bacterium or virus alters the proteins or carbohydrates on its surface and thus avoids a host immune response, making it one of the mechanisms of antigenic escape. V cholerae O antigen type 1 and O antigen type 139 typically produce cholera toxin, but very few of the many other O types produce the toxin. N meningitidis capsular polysaccharide types A and C are associated with epidemic meningitis. These surface antigens are used in serologic classification. Some bacteria and other microorganisms have the ability to make frequent shifts in the antigenic form of their surface structures in vitro and presumably in vivo. N gonorrhoeae ,the gonococcus has three surface-exposed antigens that switch forms at very high rates of about one in every 1000: lipooligosaccharide, 6–8 types; pili, innumerable types; and Opa, 10–12 types for each strain. The number of antigenic forms is so large that each strain of N gonorrhoeae appears to be antigenically distinct from every other strain. Switching of forms for each of the three antigens appears to be under the control of different genetic mechanisms. It is presumed that frequent switching of antigenic forms allows gonococci to evade the host’s immune system; gonococci that are not attacked by the immune system survive and cause disease. The Requirement for Iron Iron is an essential nutrient for the growth and metabolism of nearly all microorganisms and is an essential cofactor of numerous metabolic and enzymatic processes. The availability of iron in humans for microbial assimilation is limited because the iron is sequestered by the high-affinity iron-binding proteins transferrin in serum and lactoferrin on mucosal surfaces. Iron availability affects the virulence of many pathogens. Iron is an essential virulence factor in P aeruginosa In Listeria monocytogenes , increase in iron, results in enhanced susceptibility to infection Decreased iron availability can also be important in pathogenesis. In the presence of low iron availability, there is increased production of diphtheria toxin and potentially more severe disease. The virulence of N meningitidis for mice is increased 1000-fold or more when the bacteria are grown under iron-limited conditions. Human iron deficiency also plays a role in the infectious process. Iron deficiency affects hundreds of millions of people worldwide. Iron deficiency can affect multiple organ systems, including the immune system, and can result in impaired cell-mediated immunity and decreased polymorphonuclear cell function. Providing iron therapy during an active infection probably should be delayed because many pathogenic microorganisms can use the small amounts of supplemental iron, resulting in an increase in virulence.