UMST CHO Metabolism Pathways PDF
Document Details
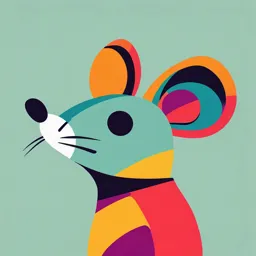
Uploaded by KeenCopernicium
UMST
Tags
Summary
This document details metabolic pathways, specifically focusing on carbohydrates and their digestion. It explains the processes of carbohydrate digestion, glycolysis, and the fates of pyruvate. The document also discusses metabolic pathways and their regulation within the body.
Full Transcript
COLLEGE OF MEDICINE AND HEALTH METABOLIC PATHWAYS IN SCIENCES CARBOHYDRATES SCHOOL OF MEDICINE AND PHARMACY METABOLISM...
COLLEGE OF MEDICINE AND HEALTH METABOLIC PATHWAYS IN SCIENCES CARBOHYDRATES SCHOOL OF MEDICINE AND PHARMACY METABOLISM MODULE CODE: MD1251 MODULE TITLE: BIOCHEMISTRY To be delivered by: 1. Dr. Samson Awotunde 2. Dr. Elizabeth Gori Course Objectives: On completion of this Unit , you should be able to: Understand the process and stages of carbohydrates digestion. Explain the importance and stages of Glycolysis. Explain the various fates of pyruvate. Describe conditions and diseases associated with digestion and glycolysis pathway. Introduction Metabolism is a topic in biochemistry that summarises a series of reactions in the body that sustain life. These reactions release energy required for biological activities such as thermo regulation, reproduction, coordination etc. Metabolism requires biomolecules such as: Proteins Fats Carbohydrates Nucleic acids That play vital roles in maintaining cellular function, structure, and energy balance, thereby sustaining life processes through metabolism Metabolic Pathway A sequence of reactions that has a specific purpose (for instance: degradation of glucose, synthesis of fatty acids) is called metabolic pathway. The pathway may be in different forms: (c) Spiral pathway (a) Linear (b) Cyclic (fatty acid biosynthesis) Introduction Metabolism involves Catabolic reactions that break down large, complex molecules to provide energy & smaller molecules. Anabolic reactions that Metabolic pathways can be grouped into two paths – catabolism and anabolism Catabolic reactions - degrade molecules to create smaller molecules and energy Anabolic reactions - synthesize molecules for cell maintenance, growth and reproduction Catabolism is characterized by oxidation reactions and by release of free energy which is transformed to ATP. Anabolism is characterized by reduction reactions and by utilization of energy accumulated in ATP molecules. Catabolism and anabolism are tightly linked together by their coordinated energy requirements: catabolic processes release the energy from food and collect it in the ATP; anabolic processes use the free energy stored in ATP to perform work. Stages of Metabolism Catabolic reactions: Stage 1: Digestion and hydrolysis break down large molecules to smaller ones that enter the bloodstream. Stage 2: Degradation Further breaking and some oxidation of molecules to 2 & 3-carbon compounds. Stage 3: Oxidation of small molecules to CO2 & H2O in the citric acid cycle and electron transport provides energy for ATP synthesis. Anabolism and catabolism are coupled by energy Metabolism Proceeds by Discrete Steps Multiple-step pathways permit Single-step vs multi-step pathways control of energy input and output Catabolic multi-step pathways provide energy in smaller stepwise amounts Each enzyme in a multi-step pathway usually catalyzes only one single step in the pathway Control points occur in A multistep enzyme pathway releases multistep pathways energy in smaller amounts that can be used by the cell Metabolic Pathways Are Regulated Levels of Metabolism Metabolism is highly regulated to permit Regulation organisms to respond to changing conditions. 1. Cell (membrane) level. Most pathways are irreversible but some are 2. Molecular level reversible. 3. Nervous system. 4. Endocrine system. Flux – is the flow of material through a metabolic pathway which depends upon: 5. Interaction between (1) Supply of substrates organs (2) Removal of products (3) Pathway enzyme activities Digestion of Carbohydrates Digestion is the first stage of metabolism in Carbohydrate is a major which large molecule are hydrolyzed or broken down into small molecules that can source of energy. be absorbed into the blood from the small Digestible carbohydrates; intestine and utilized. Examples of hydrolysis reactions are: - starch, Proteins are hydrolyzed in to amino acids - glycogen, using the proteases - disaccharides. (sucrose, Polysaccharides are hydrolyzed into monosaccharides using the glycosidases lactose and trehalose ) Triglycerides are hydrolyzed into fatty Indigestible carbohydrates: acids and glycerol using lipases - cellulose, Nucleic acids are hydrolysed by nucleases into the nucleotides. - pectins, - gums, etc Carbohydrates digestion Digestion Process Importance of Carbohydrate Digestion carbohydrates are broken down into simple, soluble sugars that can be transported across the intestinal wall into the circulatory system to be transported throughout the body. digestion begins in the mouth with the action of salivary amylase on starches and ends with monosaccharides being absorbed across the epithelium of the small intestine. Once the absorbed monosaccharides are transported to the tissues, the process of cellular respiration begins. This section will focus first on glycolysis, a process where the monosaccharide glucose is oxidized, releasing the energy stored in its bonds to produce ATP. Digestion in Mouth Digestion of carbohydrate starts at the mouth. In mouth, food undergoes mastication. During mastication, food comes in contact with saliva secreted by salivary gland). Saliva contain salivary amylase (ptyalin). Action of salivary amylase- It requires Cl- ion for activation and PH 6.7. The enzyme hydrolyzes a-(1 -4) glycosidic bonds at random deep inside polysaccharide (starch, glycogen). Producing dextrins, maltose, maitotriose, glucose. Digestion in Stomach Digestion of carbohydrate temporarily stops in the stomach. The action of salivary !amylase stops in stomak because of high acidity of stomach. No carbohydrate splitting enzymes available in gastric juice. Digestion in Intestine Further digestion of carbohydrate occurs in small intestine by pancreatic enzymes. Food bolus reaches the small intestine from stomach where it meets the pancreatic juice. Pancreatic juice contain enzyme called pancreatic amylase(amylopsin) similar to 5. amylase, There are two phase of intestinal digestion.... Digestion due to pancreatic amylase Digestion due to intestinal brush border enzyme Action of pancreatic amylase It hydrolyzes the dextrins to mixture of maltose, isomaltose, Ci t dextrin. Action of intestinal brush border enzyme These enzymes are responsible for final digestion of carbohydrate. The enzymes & their reactions are presented on the right Stages of Digestion of Starch The digestion and absorption of starch (glycogen) occur in 3 major stages; A). Intraluminal hydrolysis into oligosaccharides by α-amylase. B). Brush-border surface hydrolysis of disaccharides and oligosaccharides to their monomers. (by specific oligosaccharidases) C). Transport of monosaccharides into enterocytes A. Intraluminal hydrolysis by α-amylase Intraluminal hydrolysis begins in the mouth by salivary α-amylase This is stopped by the acidic environment of the stomach. Starch digestion is resumed by pancreatic α-amylase in the duodenum. Note: Salivary and pancreatic α-amylases exist in several isoenzyme forms Dietary carbohydrates principally consist of: I. Polysaccharides: - Starch and glycogen. II. Disaccharides: - Sucrose (cane sugar), lactose (milk sugar) and maltose. III. Small amounts monosaccharides; -like fructose and pentoses. Liquid food materials like milk, soup, fruit juice escape digestion in the mouth because they are swallowed, but solid foodstuffs are masticated thoroughly before they are swallowed. 1. Digestion in mouth: - It starts at the mouth, where they come in contact with saliva during mastication. Saliva contains a carbohydrate splitting enzyme called salivary amylase (ptyalin). Food remains in the mouth for short time. Action of Ptyalin (Salivary Amylase) It is α-amylase, it requires Cl⁻ ion for activation and optimum pH 6.7 (range 6.6 to 7.0). It hydrolyzes α-1→ 4 glycosidic linkage at random deep inside polysaccharide molecule like starch, glycogen and dextrins,(i.e endoglycosidase). It produces smaller molecules maltose, glucose and trisaccharide maltotriose. Its action stops in stomach when pH falls to 3.0 2. Digestion in Stomach: There is no carbohydrate splitting enzymes available in gastric juice. Some dietary sucrose may be hydrolysed to equimolar amounts of glucose and fructose by HCl. Although the food remains in the mouth for only a short time, the action of ptyalin continues for up to several hours in the stomach— until the food is mixed with the stomach secretions, the high acidity of which inactivates ptyalin. Ptyalin’s digestive action depends upon how much acid is in the stomach, how rapidly the stomach contents empty, and how thoroughly the food has mixed with the acid. Under optimal conditions as much as 30 to 40 percent of ingested starches can be broken down to maltose by ptyalin during digestion in the stomach. 3. Digestion in duodenum: In the small intestine, the remainder of the starch molecules (food bolus) are catalyzed mainly to maltose by pancreatic amylase. This step in starch digestion occurs in the first section of the small intestine (the duodenum), the region into which the pancreatic juices empty. The by-products of amylase hydrolysis are ultimately broken down by other enzymes into molecules of glucose, which are rapidly absorbed through the intestinal wall. a. Pancreatic juice: It contains a carbohydrate splitting enzyme pancreatic amylase ( amylopsin) similar to salivary amylase. It is also an α-amylase, optimum pH 7.1. it also requires Cl⁻ for activity. B. Brush-Border Surface Hydrolysis Brush border cells are found mainly in the small intestine , kidney and large intestine. The small intestine tract: This is where absorption takes place. The brush borders of the intestinal lining are the site of terminal carbohydrate digestions. The microvilli that constitute the brush border have enzymes for this final part of digestion anchored into their apical plasma membrane as integral membrane proteins. These enzymes are found near to the transporters that will then allow absorption of the digested nutrients. Enterocytes (intestinal absorptive cells) They are simple columnar epithelial cells which line the inner surface of the small and large intestines. A glycocalyx (i.e cell coat, is a glycoprotein and glycolipid covering which surrounds the cell membranes of epithelial cells)surface coat contains digestive enzymes. Microvilli on the apical surface increase its surface area. This facilitates transport of numerous small molecules into the enterocyte from the intestinal lumen. These small molecules include digested products of proteins, fats, and sugars, as well as water, electrolytes, vitamins, and bile salts. Enterocytes also have an endocrine role, secreting hormones such as leptin. Brush-Border Surface Hydrolysis Some oligosaccharidases and reactions they catalyse; Enzyme Hydrolysis Product Oligosaccharidases on enterocyte cell Reaction membranes hydrolyze products from sucrase α(1 --> 2) Fructose & stage 1 and digested dissaccarides to linkage of glucose monosaccarides. sucrose Monosaccharides are then transferred α(1 -+ 4) Two across the brush-border bilayer. linkage of glucose maltose molecules Hydrolysis of the oligosaccharides is not the rate limiting stage for lactase β(1 --> 4) Galactose monosaccharide absorption but linkage & glucose of lactose rather their transport system. trehalase trehalose two glucose molecules maltase …………… …………… …………… …………… ………. ………. a. Lactase: It is a β-galactosidase, its pH range is 5.4 to 6.0. Hydrolysed to equimolar amounts of glucose and galactose. Isomaltase: Catalyses hydrolysis of α-1→ 6 glycosidic linkage, thus splitting α- limit dextrin at the branching points and producing maltose and glucose Maltase: Enzyme hydrolyses the α-1→4 glycosidic linkage between glucose units in maltose molecule liberating equimolar quantities of two glucose molecules. Its pH range is 5.8 to 6.2. Sucrase: Has pH range of 5.0 to 7.0. It hydrolyses sucrose molecule to form equimolar quantities of glucose and fructose. Note: Maltase III and maltase IV also have sucrose activity. C. ABSORPTION AND TRANSPORT OF CARBOHYDRATES The digestion of carbohydrate is complete when the food materials reach the small intestine and all complex dietary carbohydrates like starch and glycogen and the disaccharides are ultimately converted to simpler monosaccharides. All monosaccharides, are completely absorbed almost entirely from the small intestine. Glucose and galactose are absorbed very fast, then fructose and mannose intermediate rate and the pentoses are absorbed slowly. Galactose is absorbed more rapidly than glucose. Why cellulose is not digested by humans Cellulose is polysaccharide found in plants. It contain 0- (1-4) glycosidic bond in its structure. Humans cannot synthesize the enzyme which can break 1- 5 glycosidic bond. Hence, cellulose is not digested by humans. Although is not digested, it is one of the important component in the human diet. undigested cellulose provides bulk as fibre in the diet. Fibre helps in intestinal motility & as a stool softer Structure of cellulose Glucose Transporters (GLUT) Glucose transporters are integral membrane proteins that mediate the transport of glucose and structurally related substances across cellular membranes. They have two families of transporters i. Facilitated diffusion glucose transporter family (GLUT family), also known as uniporters. ii. Sodium-dependent glucose transporter family (SGLT family), also known as cotransporters or symporters. i. Facilitated diffusion glucose transporter family (GLUT family), also known as uniporters Uniporters, also known as solute carriers or facilitated transporters, are a type of membrane transport protein that passively transports solutes (small molecules, ions, or other substances) across a cell membrane. It uses facilitated diffusion for the movement of solutes down their concentration gradient from an area of high concentration to an area of low concentration. Unlike active transport, it does not require energy in the form of ATP to function. Uniporters are specialized to carry one specific ion or molecule and can be categorized as either channels or carriers. Facilitated diffusion may occur through three mechanisms: uniport, symport, or antiport. The difference between each mechanism depends ii. Sodium-dependent glucose transporter family (SGLT family), also known as cotransporters or symporters. Sodium-dependent glucose cotransporters (or sodium-glucose linked transporter, SGLT) are a family of glucose transporter found in the intestinal mucosa (enterocytes) of the small intestine (SGLT1) and the proximal tubule of the nephron (SGLT2 in PCT and SGLT1 in PST). They contribute to renal glucose reabsorption. In the kidneys, 100% of the filtered glucose in the glomerulus has to be reabsorbed along the nephron (98% in PCT, via SGLT2). If the plasma glucose concentration is too high (hyperglycemia), glucose passes into the urine (glucosuria) because SGLT are saturated with the filtered glucose. Six glucose transporters have been identified in the cell membranes. They are (GLUT-1 to GLUT-5 and GLUT-7). They are tissue specific. GLUT-I is abundant in erythrocytes whereas GLUT-4 is abundant in skeletal muscle and adipose tissue. Insulin increases the number and promotes the activity of GLUT-4 in skeletal muscle and adipose tissue. In type 2 diabetes mellitus, insulin resistance is observed in these tissues because of the reduction in the quantity of GLUT-4 in insulin deficiency. Glucose Transporters. Several glucose transporter (GLUT-1 to 7) have been identified in various tissues Summary of Transport Transport of Monosaccharides into the Enterocyte By; - 1.Passive diffusion. 2. Active transport system. The Active transport system. It is carrier-mediated. Na+-dependent. Obeys Michaelis-Menten kinetics. Glucose & 2Na+ bind to membrane carrier on the luminal side. The carrier-bound Na+ and glucose are internalized along the electrochemical gradient. both are released from the carrier inside the cell. Passive diffussion Glucose is transported out of the cell into the intercellular space then to portal capillaries by; 1.serosal carrier. 2. Diffusion. 3. or both. Glucose can be converted into lactate, transported via portal blood system to the liver. Lactate in the liver is converted into glucose. Note: This transport does not use ATP directly. Its hence considered secondary active transport. Summary of Digestion GLUT1 deficiency syndrome It is also known as GLUT1-DS, GLUT1 deficiency is characterized by an array De Vivo disease or Glucose of signs and symptoms including mental and transporter type 1 deficiency motor developmental delays, infantile seizures syndrome, refractory to anticonvulsants, ataxia, dystonia, It is an autosomal dominant dysarthria, opsoclonus, spasticity, other genetic metabolic disorder paroxysmal neurologic phenomena and associated with a deficiency of sometimes deceleration of head growth also GLUT1, the protein that known as microcephaly. transports glucose across the blood brain barrier. Glucose Transporter Type 1 Deficiency Syndrome has an estimated birth incidence of 1 in 90,000 to 1 in 24,300. Fanconi–Bickel syndrome It is a form of glycogen storage disease named for Guido Fanconi and Horst Bickel, who first described it in 1949. It is associated with GLUT2, a glucose transport protein which, when functioning normally, allows glucose to exit several tissues, including the liver, nephrons, and enterocytes of the intestines, and enter the blood. The syndrome results in hepatomegaly secondary to glycogen accumulation, glucose and galactose intolerance, fasting hypoglycaemia, a characteristic proximal tubular nephropathy and severe short stature. CASE discussion 1 A 40-year-old obese female presents to the emergency center with complaints of worsening nausea, vomiting, and abdominal pain. Her pain is located in the midepigastric area and right upper quadrant. She reports a subjective fever and denies diarrhea. Her pain is presently constant and sharp in nature but previously was intermittent and cramping only after eating “greasy” foods. On examination she has a temperature of 37.8°C (100°F) with otherwise normal vital signs. She appears ill and in moderate distress. She has significant midepigastric and right upper-quadrant tenderness. Some guarding is present but no rebound. Her abdomen is otherwise soft with no distention and active bowel sounds. Laboratory values were normal except for increased liver function tests, white blood cell count, and serum amylase. Ultrasound of the gallbladder revealed numerous gallstones and a thickening of the gallbladder wall. A surgery consult was immediately sought. ◆ What is the most likely diagnosis? ◆ What is the role of amylase in digestion? Diagnosis: Gallstone pancreatitis. Role of amylase: Enzyme for carbohydrate metabolism, used to digest glycogen and starch. Obstruction of the main pancreatic duct as a result of a gallstone lodged in or near to the hepatopancreatic ampulla can result in acute pancreatitis. One theory is that obstruction increases the pressure in the main pancreatic duct. The increase in pressure causes interstitial edema, which impairs the blood flow to the acinus. The lack of blood flow leads to ischemic injury of the acinar cell, resulting in release of the digestive enzymes into the interstitial space. CLINICAL CORRELATION Acute pancreatitis is an inflammatory process in which pancreatic enzymes are activated and cause autodigestion of the gland. Alcohol use is the most common cause, and episodes are often precipitated by binge drinking. The next most common cause is biliary tract disease, usually passage of a gallstone into the common bile duct. Hypertriglyceridemia is also a common cause, and that occurs when serum triglyceride levels are greater than 1000 mg/dL, as is seen in patients with familial dyslipidemias or diabetes. When patients appear to have “idiopathic” pancreatitis, that is, no gallstones are seen on ultrasound, and no other predisposing factor can be found, biliary tract disease is still the most likely cause: either biliary sludge (microlithiasis), or sphincter of Oddi dysfunction. Abdominal pain is the cardinal symptom of pancreatitis, and it is often severe, typically in the upper abdomen with radiation to the back. The pain is often relieved by sitting up and bending forward and is exacerbated by food. Patients also commonly have nausea and vomiting, also precipitated by oral intake. The treatment includes nothing by mouth, intravenous hydration, pain control, and monitoring for complications METABOLIC PATHWAYS IN CARBOHYDRATES Overview of Carbohydrate Metabolism Glycolysis Two greek words ie glykys- “sweet” lysis- “splitting” It occurs in the cytosol & is common to most organisms. Glycolysis involves 10 reactions that result in the breakdown of glucose (hexose) into two pyruvate molecules (triose) with conservation of chemical potential energy in the form of ATP and NADH. Note: all the 10 reactions are anaerobic. Embden–Meyerhof–Parnas Pathway (Glycolysis) The pathway was given the name to commemorate the work of Gustav Embden, Otto Meyerhof, and Jacob Parnas in its elucidation. The major pathways of carbohydrate metabolism either begin or end with glucose Glucose is a major source of metabolic energy in many cells is the major form in which carbohydrate absorbed from the intestinal tract is presented to cells of the body. Glucose is the only fuel used to any significant extent by a few specialized cells and the major fuel used by the brain. Glucose is so important to these specialized cells and the brain that several of the major tissues of the body work together to ensure a continuous supply of this essential substrate. Glucose metabolism is defective in two very common metabolic diseases, obesity and diabetes, contribute in development of a number of major medical problems, including Embden–Meyerhof–Parnas Pathway The major pathways of carbohydrate metabolism either begin or end with glucose Glucose is a major source of metabolic energy in many cells is the major form in which carbohydrate absorbed from the intestinal tract is presented to cells of the body. Glucose is the only fuel used to any significant extent by a few specialized cells and the major fuel used by the brain. Glucose is so important to these specialized cells and the brain that several of the major tissues of the body work together to ensure a continuous supply of this essential substrate. Glucose metabolism is defective in two very common metabolic diseases, obesity and diabetes, contribute in development of a number of major medical problems, including Embden–Meyerhof–Parnas Pathway It plays a key role in energy metabolism by: providing a significant portion of the free energy used by most organisms and preparing glucose and other compounds for further oxidative degradation Glycolysis involves the breakdown of glucose to pyruvate while using the free energy released in the process to synthesize ATP from ADP and Pi. The 10-reaction sequence of glycolysis is divided into two stages: i. Energy investment and ii. Energy recovery. Embden–Meyerhof–Parnas pathway Embden–Meyerhof–Parnas pathway Reactions of glycolysis The pathway can also be divided into three distinct phases. 1. Energy investment phase or priming stage. 2. Splitting phase. 3. Energy generation phase. 1. Energy investment phase. Glucose is phosphorylated to glucose-6-phosphate by hexokinase (HK) or glucokinase enzyme, which splits the ATP into ADP, and the Pi is added on to the glucose. The energy released by the hydrolysis of ATP is utilized for the forward reaction. The enzyme catalyses a regulatory step that is irreversible. The enzyme is almost in all tissues, it catalyses the phosphorylation of various hexoses. Inhibited by glucose 6-phosphate. Glucokinase enzyme, which splits the ATP into ADP, and the Pi is added on to the glucose. The energy released by the hydrolysis of ATP is utilized for the forward reaction. The enzyme catalyses a regulatory step that is irreversible. The enzyme is almost in all tissues, it catalyses the phosphorylation of various hexoses. Inhibited by glucose 6-phosphate. Embden–Meyerhof–Parnas pathway Glucokinase is present in the liver. Phosphorylates only glucose. It is not inhibited by glucose 6-phosphate. It is under the influence of insulin. 1. The phosphorylation of glucose traps it within the cells. Once phosphorylated, glucose-6-phosphate is trapped within the cell and has to be metabolized. 2. Glucose-6-phosphate is isomerised to fructose-6-phosphate by phosphohexose isomerase and mg²⁺. This step is reversible. Then fructose-6-phosphate is further phosphorylated to fructose1,6-bisphosphate by the enzyme phosphofructokinase. PFK is an allosteric, inducible, regulatory enzyme. It is an important key enzyme of this pathway. This is again an activation process, the energy being derived by hydrolysis of yet another molecule of ATP. This irreversible step is the rate limiting reaction in glycolysis. However, during gluconeogenesis, this step is circumvented by fructose-1,6-bisphosphatase. Embden–Meyerhof–Parnas pathway 2. Splitting phase. 4. The 6 carbon fructose-1,6-bisphosphate is cleaved into two 3 carbon units. One is glyceraldehyde-3- phosphate and another molecule of dihydroxyacetone phosphate (DHAP). Since the backward reaction is an aldol condensation, the enzyme is called aldolase. This reaction is reversible. 5. Dihydroxy acetone phosphate is isomerised to glyceraldehyde-3-phosphate by the enzyme phosphotriose isomerase. Glucose is now cleaved into 2 molecules of glyceraldehyde-3-phosphate. The steps are called the splitting phase. The enzyme is inhibited by bromohydroxyacetone phosphate. 3. Energy generation phase. 6. Glyceraldehyde-3-phosphate is dehydrogenated and simultaneously phosphorylated to 1,3-bisphospho glycerate (1,3-BPG) with the help of NAD⁺. The enzyme is glyceraldehyde-3phosphate dehydrogenase. The product contains a high energy bond. This is a reversible reaction. This enzyme brings about 2 reactions i.e. i). Oxidation, where the hydrogens are transferred to NAD⁺ and ii). Phosphorylation of the substrate adding one phosphate moiety. Embden–Meyerhof–Parnas pathway This step is important because it is involved in the formation of NADH + H⁺ and a high energy compound 1,3-bisphospoglycerate. The enzyme phosphoglycerate kinase acts on 1,3-bisphosphoglycerate resulting in the synthesis of ATP and formation of 3- phosphoglycerate. The step is an example of substrate level phosphorylation, since ATP is synthesized from substrate without ETC involvement. Phosphoglycerate kinase is reversible. 3-phospho glycerate is isomerized to 2-phospho glycerate by shifting the phosphate group from 3rd to 2nd carbon atom. The enzyme involved is phosphoglucomutase. This is a readily reversible reaction. 2-phosphoglycerate is converted to phosphoenol pyruvate by the enzyme enolase. One water molecule is removed. Embden–Meyerhof–Parnas pathway Enolase requires Mg2⁺, and by removing magnesium ions, fluoride will irreversibly inhibit this enzyme. Thus, fluoride will stop the whole glycolysis. So when taking blood for sugar estimation, fluoride is added to blood. If not, glucose is metabolized by the blood cells, so that lower blood glucose values are obtained. Phosphoenol pyruvate (PEP) is dephosphorylated to pyruvate, by pyruvate kinase. First PEP is made into a transient intermediary of enol pyruvate, which is spontaneously isomerized into keto pyruvate, the stable form of pyruvate. One mole of ATP is generated during this reaction. This is again an example of substrate level phosphorylation. The pyruvate kinase is a key glycolytic enzyme. This step is irreversible. The reversal, however, can be brought about in the body with the help of two enzymes (pyruvate kinase and phosphoenol pyruvate carboxy kinase) and hydrolysis of 2 ATP molecules. In anaerobic condition, pyruvate is reduced to lactate by lactate dehydrogenase (LDH). In aerobic conditions, the pyruvate enters the citric acid cycle for complete oxidation. The end product of anaerobic glycolysis is lactate which enters the Cori's cycle. Steps of glycolysis Glycolysis Step -1 hexokinase phosphorylates glucose in the cell's cytoplasm. a phosphate group from ATP is transferred to glucose producing glycose 6-phosphate. Glucose-e +ATP —ADP+ glucose 6phosphate step2 phosphoglucoisomerase converts glucose 6-phosphate into its isomer fructose6- phosphate. Glucose6-phosphate +phosphoglucoisomerase fructose 6- phosphate step3 phosphofructokinase uses another ATP molecule to transfer a phosphate group to fructose 1,6-bisphosphate. Fructose 6 phosphate + phosphofructokinase +ATP ADP +fructose — bisphosphate. Step 4 Aldolase splits fructose 1,6 bisphosphate into 2 sugar isomers of each other. viz dihydroxycetone phosphate and glyceraldehyde phosphate Fructose 1,6- bisphosphate + aldolase dihydroxyacetone phosphate + glyceraldehyde phosphate step5 triose phosphate isomerase rapidly inter-converts the molecules dihydroxyacetone phosphate and glyceraldehyde phosphate. Glyceraldehyde phosphate is removed as soon as it is formed to be used in the next step of glycolysis. Dihydroxyacetone phosphate glyceraldehyde phosphate step6 triose phosphate dehydrogenase serves 2 functions in this step 1. First the enzyme transfers a H from glyceraldehyde phosphate to the oxidizing agent NAD to form NADH. 2.Next it adds a phosphate from the cytosol to the oxidized glyceraldehyde phosphate to form 1,3bisphosphosphoglycerate. This occurs for both molecules of glyceraldehyde phosphate produced in step -5 A. triose phosphate dehydrogenase+ 21-1 +2NAD 2NADH +21-1 B. Triose phosphate dehydrogenase +2 P +2 glyceraldehyde phosphate 2 molecules of 1,3- bisphosphoglycerate step-7 phosphoglycerokinase transfers a P from — bisphosphoglycerate to a molecule of ADP to form ATP. This happens for each molecule of 1,3biphosphoglycerate. The process yields two 3- phosphoglycerate molucules and two ATP molecules. 2 molecules of 1,3- bisphoshoglycerate + phosphoglycerokinase + 2 ADP 2 molecules of 3- phosphoglycerate + 2ATP step-8 phosphoglyceromutase relocates the P from 3- phosphoglycerate from C3 to C2 to form 2 phosphoglycerate. 2molecules of 3-phosphoglycerate + phosphoglyceromutase 2 molecules of 2- phosphoglycerate step -9 enolase removes a molecule of water from 2- phosphoglycerate to form phosphoenolpyruvic acid This happens for each molecule of 2phosphoglycerate. 2 molecules of 2— phosphoglycerate + enolase 2 molecules of phosphoenolpyruvic acid (PEP) Step-10 The enzyme pyruvate kinase transfers a P from PEP to ADP to form pyruvic acid and ATP This happens for each molecule of PEP This reaction produces 2 molecules of pyruvic acid and 2 ATP molecules. Enzymes of Glycolysis There are ten different enzymes involved in glycolysis. These are listed below with their roles in each step: 1. Hexokinase: It activates glycolysis by phosphorylating glucose. This is the rate-limiting step in glucose metabolism. 2. Phosphohexose isomerase: It changes glucose-6-phosphate to its isomer fructose-6-phosphate. 3. Phosphofructokinase: It adds another phosphate group to fructose-6-phosphate with the help of ATP (Adenosine Triphosphate). 4. Aldolase: Breaks down the six-carbon fructose-6-phosphate to 3 carbon dihydroxyacetone phosphate (DHAP) and glyceraldehyde-3-phosphate (GAP). 5. Isomerase: This is another enzyme that converts dihydroxyacetone phosphate to its isomer glyceraldehyde-3-phosphate. 6. Glyceraldehyde-3-phosphate dehydrogenase: It has two uses; it dehydrogenates GAP and adds phosphate group into GAP and converts it into 1,3- biphosphoglycerate. 7. Phosphoglycerate kinase: Helps transfer one phosphate molecule into ADP (adenosine diphosphate). 8. Phosphoglycerate mutase: Helps change phosphate’s position from third to second; that is, 3-phosphoglycerate is transformed into 2-phosphoglycerate. 9. Enolase: Helps remove a molecule of water from 2-phosphoglycerate to form phosphoenolpyruvate (PEP). 10. Pyruvate kinase: It works in transferring phosphate of phosphoenolpyruvate to form pyruvate. Glycolysis is only the first step in the degradation of glucose. Divided into two phases; 1. preparatory phase. 2. payoff phase. Preparatory phase Step 1: Phosphorylation of glucose. Glucose is activated by phosphorylation at C6 catalyzed by hexokinase (glucokinase in the liver) The enzyme is present in virtually all extrahepatic cells & has high affinity (low Km) for glucose Step 2: Reversible isomerization of glucose 6- phosphate to fructose 6-phosphate Catalysed by phosphohexose isomerase the reaction requires Mg2+ ions Step 3: Phosphorylation of fructose 6-phosphate to fructose 1, 6-bisphosphate This irreversible reaction is catalysed by Phosphofructokinase-1 in the presence of ATP. PFK-1 has a regulatory role in glycolysis. Step 4: Cleavage of fructose 1, 6-bisphosphate Catalysed by aldolase results into formation of two triose phosphates, glyceraldehyde 3-phosphate and dihydroxyacetone phosphate. This reaction is reversible Step 5: Interconversion of the triose phosphates In the presence of triose phosphate isomerase, Dihydroxyacetone phosphate is rapidly and reversibly converted to glyceraldehyde 3-phosphate. This reaction completes the preparatory phase of glycolysis forming two molecules of GAP. The payoff phase. In this phase, - the 2 molecules of glyceraldehyde 3-phosphate are converted into 2 molecules of pyruvate. - 2 molecules of ATP + NADH are produced. Step 6: oxidation of glyceraldehyde 3-phosphate to 1, 3- bisphosphoglycerate Catalysed by GAP dehydrogenase results into dehydrogenation of the aldehyde group to an acyl phosphate. GAP dehydrogenase is inhibited by iodoacetetate Step 7: Phosphoryl transfer from 1, 3-bisphospho- glycerate to ADP The enzyme phosphoglycerate kinase transfers the high-energy Phosphoryl group from the carboxyl group to ADP, forming ATP and 3-phosphoglycerate. Steps 6 and 7 represent an energy-coupling process in which 1, 3-phosphoglycerate is the common intermediate. GAP+ ADP + Pi +NAD+ PG + ATP + NADH + H+ Step 8: Conversion of 3-phosphoglycerate to 2- phosphoglycerate. Phosphoglycerate mutase catalyzes this reversible reaction that requires Mg2+ Step 9: Dehydration of 2-phosphoglycerate to phosphoenolpyruvate Enolase promotes reversible removal of a molecule of water from 2-phosphoglycerate to yield phosphoenolpyruvate The loss of the water molecule causes a redistribution of energy within the molecule, generating a super high-energy phosphate compound Step 10: Transfer of the phosphoryl group from phosphoenolpyruvate to ADP This is the last step in glycolysis, catalyzed by pyruvate kinase, which requires K+ and Mg 2+ or Mn 2+ The product pyruvate undergoes tautomerization from its enol to keto form which is more stable at pH 7 Overall balance sheet - net gain of ATP Glucose + 2 ATP+ 2 NAD+ + 4 ADP + 2 Pi 2 Pyruvate + 2 ADP + 2 NADH + 2 H+ + 4 ATP + 2 H2O Or Glucose + 2 NAD+ + 2 ADP + 2 Pi 2 Pyruvate + 2 NADH + 2 H+ + 2 ATP + 2 H2O Under aerobic conditions, the two molecules of NADH are re-oxidized to NAD+ by transfer of their electrons to the respiratory chain in the mitochondrion 2 NADH + 2 H+ + O2 2 NAD+ + 2 H2O During glycolysis: *Carbon pathway - Glucose 2x pyruvate *Phosphate pathway - 2 ADP + 2 Pi 2 ATP *Electron pathway - Four electrons (two hydride ions) are transferred from 2 molecules of glyceraldehyde-3-phosphate to two of NAD+ These are all exergonic and physiologically Glycolysis is regulated at 3 irreversible steps involving non These enzymes function as “valves”, regulating equilibrium reactions the flow of carbon through glycolysis. The Step 1: hexokinase rates of these steps are limited not by the substrate but by the activity of the enzymes. glucose glucose 6-phosphate Enzymes that catalyze these exergonic, rate- Step 3: phosphofructokinase limiting steps are commonly the targets of fructose 6-phosphate fructose metabolic regulation. 1, 6-bisphosphate Examples of regulation: Step 10: pyruvate kinase Phosphofructokinase-1 - inhibited by high phosphoenolpyruvate pyruvate levels of ATP. ATP binds to an allosteric site and lowers affinity for fructose 6-phosphate Hexokinase - allosterically inhibited by its product. Pyruvate kinase - inhibited by ATP Embden–Meyerhof–Parnas Pathway 1. Glucokinase/Hexokinase Phosphorylation of glucose by these enzymes is a reaction which is regulated by feed back inhibition (hexokinase by glucose-6-phosphate) and activated by insulin (glucokinase is induced by insulin). Glucokinase that is active mainly in the liver has a high Km for glucose and low affinity. Therefore, glucokinase can act only when there is adequate glucose supply so that excess can be stored. Hexokinase with low km and high affinity can phosphorylate glucose even at lower concentrations so that glucose is made available to the brain, cardiac and skeletal muscle. Glucokinase can act only when there is plenty of glucose. When the supply of glucose is limited, glucose is made available to brain and muscles. 2. Phosphofructokinase (PFK). It is the most important rate-limiting enzyme for glycolysis pathway. ATP and citrate are the most important allosteric inhibitors. AMP acts as an allosteric activator. 3. Pyruvate Kinase catalyses an irreversible step and is a regulatory enzyme of glycolysis. When energy is plenty in the cell, glycolysis is inhibited. Insulin increases its activity whereas glucagon inhibits. Pyruvate kinase is inactive in the phosphorylated state. METABOLIC FATE OF PYRUVATE Under aerobic conditions, pyruvate is converted to acetyl CoA which enters the TCA cycle to be oxidized to CO₂. ATP is generated. Glycolysis takes place in the cytoplasm. Hence, pyruvate is generated in cytoplasm. It is then transported into the mitochondria by a pyruvate transporter. Pyruvate Dehydrogenase Complex Inside the mitochondria, pyruvate is oxidatively decarboxylated to acetyl CoA by pyruvate dehydrogenase (PDH). PDH is a multi-enzyme complex with 5 co-enzymes and 3 apo-enzymes. The coenzymes needed are: 1. Thiamine pyrophosphate (TPP) 2. Co-enzyme A (CoA) 3. FAD 4. NAD⁺ 5. Lipoamide. The lipoic acid, otherwise called thioctic acid has two sulphur atoms and 8 carbon atoms. It can accept or donate hydrogen atoms. Pyruvate Dehydrogenase Complex 6. The enzyme part of the PDH complex is made up of three component enzymes. A. Pyruvate Dehydrogenase (Enzyme 1): It catalyses oxidative decarboxylation. TPP is required in this step. So, Thiamine, a B-complex group vitamin is essential for utilization of pyruvate. The two carbon unit remains attached to the enzyme, as hydroxyethyl thiamine pyrophosphate. B. Dihydro Lipoyl Trans Acetylase (Enzyme 2): Then, hydroxyethyl group is oxidized to form an acetyl group and then transferred from TPP to lipoamide to form acetyl lipoamide. C. Dihydro Lipoyl Dehydrogenase (Enzyme 3): The last step is the oxidation of lipoamide. At the end of the reaction the cofactors, namely TPP, Lipoamide and FAD are regenerated Pyruvate Dehydrogenase Complex Regulation PDH is subject to regulation by allosteric mechanisms and covalent modification. Allosteric inhibitors are the products acetyl CoA and NADH. PDH or E1 is covalently modified by phosphorylation by PDH kinase and dephosphorylated by PDH phosphatase. The dephosphorylated form of the enzyme is active. Hence activators of PDH kinase like ATP, NADH and acetyl CoA inhibit PDH reaction. PDH phosphatase is activated by Ca⁺⁺, mg⁺⁺and AMP, this will increase the rate of PDH reaction where as PDH kinase is inhibited by Ca⁺⁺. When there is adequate ATP and acetyl CoA, the enzyme is inhibited. Importance of Pyruvate Dehydrogenase 1. Completely irreversible Process. There is no pathway available in the body to circumvent this step. Glucose through this step is converted to acetyl CoA from which fatty acids can be synthesized. 2. The backward reaction is not possible, and so there is no net synthesis of glucose from fat. 3. Pyruvate may be channeled back to glucose through gluconeogenesis. But when pyruvate becomes acetyl CoA, it cannot go back. Thus, pyruvate dehydrogenase step is the committed step towards oxidation of glucose. 4. Energetics: The NADH generated in this reaction, enters the electron transport chain to produce 2.5 ATP molecules. 5. Pyruvate dehydrogenase is regulated by end product inhibition as well as by covalent modification. Phosphorylation of the enzyme by a kinase decreases the activity of the enzyme. Dephosphorylation activates the enzyme. OR Oxidative decarboxylation of pyruvate to acetyl-CoA by the PDH complex. In step 1 pyruvate reacts with the bound thiamine pyrophosphate (TPP) of pyruvate dehydrogenase (E1), undergoing decarboxylation to the hydroxyethyl derivative Pyruvate Dehydrogenase Complex CASE STUDY A 59-year-old male is brought to the emergency department by the EMS after a family member found him extremely confused and disoriented, with an unsteady gait and strange irregular eye movements. The patient has been known in the past to be a heavy drinker. He has no known medical problems and denies any other drug usage. On examination, he is afebrile with a pulse of 110 beats per minute and a normal blood pressure. He is extremely disoriented and agitated. Horizontal rapid eye movement on lateral gaze is noted Diagnosis: Wernicke-Korsakoff syndrome (thiamine deficiency) often associated with chronic alcoholics. CLINICAL CORRELATION Thiamine, also known as vitamin B1, is fairly Importance of thiamine: ubiquitous. Thiamine deficiency is uncommon except in alcoholics as a result of nutritional deficiencies and An important water-soluble vitamin malabsorption. The classic clinical triad of dementia, used as ataxia (difficulty with walking), and eye findings may a cofactor in enzymatic reactions be seen, but more commonly, only forgetfulness is noted. Sometimes, thiamine deficiency can lead to involving the transfer of an vague symptoms such as leg numbness or tingling. aldehyde Because thiamine is water soluble, it can be added to group. Without thiamine, intravenous fluids and administered in that way. Other individuals can develop dementia, manifestations include beri beri, which is cardiac macrocytic involvement leading to a high cardiac output, and vasodilation. Affected patients often feel warm and anemia (folate deficiency), gastritis, flushed, and they can have heart failure. peptic ulcer disease, liver disease, depression, nutritional deficiencies, cardiomyopathy, and pancreatitis. Fate of Pyruvate Metabolic fate of pyruvate 1. Under aerobic conditions, complete oxidation of the pyruvate carbon atoms to CO2 is mediated by the citric acid cycle The energy released in that process drives the synthesis of much more ATP than is generated by the limited oxidation of glucose by the glycolytic pathway alone. In anaerobic metabolism, pyruvate is metabolized to a lesser extent to regenerate NAD+ Other fates of Pyruvic acid can be: Pyruvic acid can be aminated to form the amino acid alanine Pyruvic acid can be converted to form glucose during gluconeogenesis Pyruvic acid can be converted to malic acid, the reaction which is vital for gluconeogenesis. Pyruvic acid can be converted directly to oxaloacetic acid in the body by CO2- fixation (CO2-assimilation) reaction. 2. Homolactic Fermentation Converts Pyruvate to Lactate In muscle, during vigorous activity, when the demand for ATP is high and oxygen is in short supply, ATP is synthesized largely via anaerobic glycolysis, which rapidly generates ATP, rather than through the slower process of oxidative phosphorylation. Under these conditions, lactate dehydrogenase (LDH) catalyzes the oxidation of NADH by pyruvate to yield NAD+ and lactate The lactate dehydrogenase reaction is freely reversible, so pyruvate and lactate concentrations are readily equilibrated. In pyruvate reduction by LDH, a hydride ion is stereospecifically transferred from C4 of NADH to C2 of pyruvate The overall process of anaerobic glycolysis in muscle can be represented as Glucose + 2 ADP + 2 Pi → 2 lactate + 2 ATP + 2 H2O + 2 H Lactate represents a sort of dead end for anaerobic glucose metabolism. The lactate can be either exported from the cell or converted back to pyruvate. Much of the lactate produced in skeletal muscle cells is carried by the blood to the liver, where it is used to synthesize glucose It is not lactate buildup in the muscle that causes muscle fatigue and soreness, but the accumulation of glycolytically generated acid Muscles can maintain their workload in the presence of high lactate concentrations if the pH is kept constant 3. Alcoholic Fermentation Converts Pyruvate to Ethanol and CO2 Under anaerobic conditions in yeast, NAD+ for glycolysis is regenerated in a process that has been valued for thousands of years: the conversion of pyruvate to ethanol and CO2 Yeast produces ethanol and CO2 via two consecutive reactions 1. The decarboxylation of pyruvate to form acetaldehyde and CO2 as catalyzed by pyruvate decarboxylase (an enzyme not present in animals). 2. The reduction of acetaldehyde to ethanol by NADH as catalyzed by alcohol dehydrogenase, thereby regenerating NAD+ for use in the GAPDH reaction of glycolysis. The two reactions of alcoholic fermentation CORI'S CYCLE OR LACTIC ACID CYCLE Definition: It is a process in which glucose is converted to lactate in the muscle. In the liver this lactate is re-converted into glucose. In an actively contracting muscle, pyruvate is reduced to lactic acid which may tend to accumulate in the muscle. The muscle cramps, often associated with strenuous muscular exercise, are thought to be due to lactate accumulation. To prevent the lactate accumulation, the body utilises Cori's cycle. This lactic acid from muscle diffuses into the blood. Lactate then reaches the liver, where it is oxidized to pyruvate. It is then channelled to gluconeogenesis. Regenerated glucose can enter into blood and then to muscle. This cycle is called Cori's cycle. Significance of the Cori's cycle The lactate produced in the muscle is efficiently reutilized by the body. Embden–Meyerhof–Parnas Pathway In this series of five reactions, a hexose is phosphorylated, isomerized, phosphorylated again, and then cleaved to two interconvertible triose phosphates. Two ATP are consumed in the process This is an energy-consuming process. During exercise, lactate production is high, which is utilized by the liver to produce glucose. This process needs ATP in significant quantities, which is provided by increased metabolism. This leads to increased oxygen consumption. This is the explanation for the oxygen debt after vigorous exercise. Schematic diagram of the first stage of glycolysis. Significance of the Glycolysis Pathway 1. It is the only pathway that is taking place in all the cells of the body. Its enzymes are present in the cytosomal fraction 2. Glycolysis is the only source of energy in erythrocytes. 3. Occurs in the absence of oxygen (anaerobic) or presence of oxygen (aerobic). Lactate is the end product under anaerobic condition while Pyruvate is the end product of aerobic condition, which is then oxidized to CO₂ and H₂O. 4. The pathway is an emergency energy-yielding pathway for cells in the absence of oxygen. 5. Its occurrence is a prerequisite for the aerobic oxidation of carbohydrates, which takes place in the cells possessing mitochondria. 6. It is a major pathway for ATP synthesis in tissues lacking mitochondria, e.g. erythrocytes, cornea, lens etc. 7. The pathway may be considered as the preliminary step before complete oxidation. 8. In some tissues which have relatively few mitochondria e.g. testes, leucocytes and kidney medulla, glycolysis is significant for ATP production. 9. The pathway provides carbon skeletons for synthesis of non-essential amino acids as well as glycerol part of fat. 10. Most of the reactions of the pathway are reversible, which are also used for gluconeogenesis. Biomedical Importance Of crucial biomedical significance is the ability of glycolysis to provide ATP in the absence of oxygen. This allows skeletal muscle to perform at high levels when aerobic oxidation becomes insufficient, and allows cells to survive anoxic episodes. Diseases associated with impaired glycolysis: Hemolytic anemia: - of the defects in glycolysis that cause hemolytic anemia, pyruvate kinase deficiency (genetic mutations) is the most common. mature erythrocytes contain no mitochondria, totally dependent upon glycolysis for ATP. - ATP is required for Na/K-ATPase-ion transport system which maintain the proper shape of the erythrocyte Recommended Core Reference 1. Donald Voet, Judith G Voet, Charlotte W. Pratt. (2016). Fundamentals of Biochemistry: Live at Molecular Level, 5th Edn. Wiley, USA. 2. Harper’s Biochemistry. 3. Lehninger Principles of Biochemistry 4. Bhagavan Medical Biochemistry