Ultrasonography 1-4 PDF
Document Details
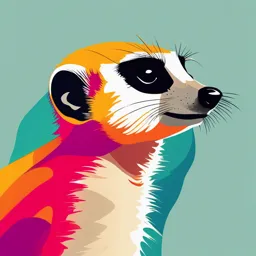
Uploaded by SublimeCharacterization
Tags
Summary
This document provides an overview of ultrasound physics, covering topics like sound wave definitions, categories, and wave characteristics. It also explores the concepts of frequency, wavelength, and wave velocity.
Full Transcript
Ultrasonography I (DRAD322) Lecture 1 Basics of Ultrasound Physics Lecture Objectives: Definition of sound and sound waves Explain the principles of sound waves Learn about categories of sound....
Ultrasonography I (DRAD322) Lecture 1 Basics of Ultrasound Physics Lecture Objectives: Definition of sound and sound waves Explain the principles of sound waves Learn about categories of sound. Teach how to use these principles to help your diagnostic abilities. Basics of Sound & Sound waves Sound: energy transmitted as a mechanical, longitudinal wave that requires a medium through which to travel Sound is Measured in Hertz (Hz). ❖ one hertz =cycle per second ❖ 1 kHz = 1000 Hz ❖ 1 MHz = 1000 kHz, 1000,000 Hz Classification of sound Audible Sound Infrasound. Ultrasound. Audible Sound: Sound frequencies between 20 to 20,000 hertz. Infrasound: Sound frequencies below the range of human hearing are known as infrasound. > 20 hertz Ultrasound: Sound frequencies above the human audible range is known as ultrasound. < 20,000 hertz CATEGORIES OF SOUND Basics Sound requires a medium through which travels The wave travels through a medium by compressing and rarefacting matter. Depending on the matter- the wave will travel at different velocities or directions. Sound waves Sound waves are consisting of mechanical vibrations called condensations (compressions) & rarefactions (decompressions) that are transmitted through a medium. 1 cycle= compressions + rarefaction Terminology Sonogram: the pictorial record of an ultrasound examination (the US image itself) Scattering: this occurs when a sound wave strikes an interface between two small objects that is equal to or smaller than the wavelength Scatterer: an object that causes scattering Acoustic: pertaining to sound waves Amplitude: the height of a wave. The amplitude and intensity of sound represent the energy of sound wave Specular reflectors: the interfaces responsible for the major organ outlines seen in ultrasound imaging, dependent on the incident beam angle and the reflector surface 1. Smooth surface: the interface is large compared with the wavelength size of the sound wave 2. Rough surface: when the undulations are approximately one wavelength or less then sound is reflected in all directions, this is known as diffuse reflection Exposure: the condition of being scanned with an ultrasound transducer. Increasing the power to the transducer produces high intensity ultrasound pulse Exposure time: the total amount of time the transducer assembly is delivering ultrasonic energy to the subject. For a pulsed system (diagnostic ultrasound imaging systems operate in the pulsed mode), this includes the time between pulses (pulse repetition period) Pulse Repetition Frequency: The PRF is set in order to allow time for the most distant echoes to return before sending next pulse Time Gain Compensation: the TGC compensate for this loss of signal strength so that equal amplitudes can be displayed from all depths as the same brightness on the image Gain: the degree of amplification of the returning wee displayed on ultrasound image, usually expressed in decibels (dB). Gain is an input function Artifact: an echo feature, present or absent in a sonogram, which does not correspond to the presence or absence of a real target e.g. Reverberations and shadowing Wave Terminology Frequency(f): the number of cycle per second. Expressed in Hertz(Hz). 1Hz = 1cycle per second Wave length(λ): the distance between two any two consecutive identical points on a wave, which is typically between 0.1-1 mm for medical ultrasound imaging The wavelength of a sound wave depends on both the frequency (f) and the propagation speed (c). λ= c/f Velocity(c): speed of sound with direction specified Wave Terminology The wavelength and frequency of US are inversely related, i.e., ultrasound of high frequency has a short wavelength and vice versa Wave Terminology Period (T): the time taken for a particle in the medium through which the wave is traveling to make one complete cycle. as frequency increases, period decreases if frequency in Hz, period in seconds/cycle if frequency in kHz, period in msec/cycle if frequency in MHz, period in microsec/cycle Period / Frequency If frequency = 2 MHz then sound period is 1/2 = 0.5 msec If frequency = 10 kHz then sound period is 1/10 = 0.1 msec If frequency = 50 Hz then sound period is 1/50 = 0.02 sec If sound period = 0.2 msec then frequency = 1/0.2 = 5 MHz If sound period = 0.4 msec then frequency = 1/0.4 = 2.5 kHz If sound period = 0.1 sec then frequency = 1/0.1 = 10 Hz SPEED OF SOUND Propagation velocity: It depends on tissue density and is lowest in air, higher in soft tissue, and highest in solids The denser the medium, the greater the acoustic impedance, and the faster the sound will travel Propagation Speed of Sound (velocity) - For body tissues in the diagnostic ultrasound range, the speed of sound is (approximately) independent of frequency and depends primarily on the physical makeup of the material. - physical makeup of the material determines tissue’s resistance to compression (density or stiffness). Propagation Speed of Sound (velocity) Increased by increasing stiffness Reduced by increasing density Each medium has a constant velocity of sound (c).. e.g: ❖ Bone: 4,080 m/sec ❖ Air: 330 m/sec ❖ Soft Tissue Average: 1,540 m/sec NOTE all ultrasound scanners use this value for the speed of sound to compute tissue depth. Propagation Speeds in Soft Tissues and Other Materials: Amplitude The degree of variance from the normal. The maximum variation in an acoustic variable. Pulse Repetition Frequency (PRF) # of sound pulses per unit time. Is independent of sound frequency. Is determined by source. Unit: hertz. clinical range (typical values): - 1 - 10 KHz Pulse Repetition Period (PRP) Time from beginning of one pulse until beginning of next. Time between corresponding points of adjacent pulses. Pulse Repetition Period Pulse repetition period is reciprocal of pulse repetition frequency. as pulse repetition frequency increases, pulse repetition period decreases. Units: any unit of time. pulse repetition period & frequency determined by source. Pulse Duration Time it takes for one pulse to occur. Units: time per pulse (time/pulse). Equation: Period X # cycles per pulse pulse duration = ------------------------------------------------------------ (time/pulse) (time/cycle) (cycles/pulse) Medical Diagnostic Ultrasound >1MHz Lecture 2 Physical Principles of Sound Wave Lecture Objectives: I. Review basic physics vocabulary II. Learn about basic principles of sound waves III. Teach how to use these principles to help your diagnostic abilities INTENSITY The intensity of an ultrasonic beam at a point is the rate of flow of energy through unit area perpendicular to the beam at that point. Determines the sensitivity of the instrument, i.e. the number and sizes of echoes recorded. Intensity is highest in the focal zone of the beam. Intensity decreases towards the edge of the beam. Acoustic Impedance (Z) Acoustic impedance is the tissue’s resistance to sound. Is independent of frequency Acoustic impedance of most tissues is constant. Determines the amount of sound waves reflected at an interface. Different tissues creates Differences in acoustic impedance called Impedance mismatch = ΔZ ΔZ create reflective interfaces that reflects the u/s waves. Homogeneous mediums (no ΔZ) reflect no sound cont Acoustic Impedance (Z) The acoustic impedance unit is called the Rayl (kg/m2/s) Acoustic impedance (Z) of a material is given by: (Z) (Rayl) = speed of sound in material c (m/s) X density of material D (kg/m3) impedance density cont impedance speed of sound Acoustic Impedance MISMATCH Z1=Z2 : Substances with same acoustic impedance there is total transmission of energy (100%) and therefore no reflection Z1Z2: small difference in (Z) results in a large amount of energy transmitted (95-99%) and small amount of energy reflected e.g. fat/muscle Z1Z2: if there is a large difference in (Z), there is a large amount of reflected energy e.g. bone/muscle or air/soft tissue Acoustic Impedance (Z) acoustic interfaces create visual boundaries between different tissues. Bone/tissue or air/tissue interfaces with large Δz values reflect almost all the sound Muscle/fat interfaces with smaller Δz values reflect only part of the energy Reflection The production of echoes at reflecting interfaces between tissues of differing physical properties. Reflection Specular Reflection Large smooth interfaces (e.g. diaphragm, bladder wall) reflect sound like a mirror Specular Reflection Only the echoes returning to the machine are displayed Specular reflectors will return echoes to the machine only if the sound beam is perpendicular to the interface Diffuse Reflector Interfaces may be smaller than the wavelength of the sound Most echoes that arised from small interfaces within solid organs The echoes produced scatter in all directions These echoes form the characteristic pattern of solid organs and other tissues Refraction A change in direction of the sound wave as it passes from one tissue to a tissue of higher or lower sound velocity Refraction U/S scanners assume that an echo returns along a straight path Distorts depth reading by the probe Minimize refraction by scanning perpendicular to the interface that is causing the refraction ATTENUATION Attenuation is the reduction or decrease in the intensity and amplitude of sound waves as they travel through a medium. The unit of attenuation is the bel, or the more commonly used decibel (dB). Decibel is actually one- tenth of a bel Attenuation is caused by the following processes: Absorption Reflection Scattering Beam divergence Absorption This is the main factor causing attenuation of the ultrasound beam Increases linearly with ultrasound frequency Reflection The amount of energy that is reflected depends on the size of the acoustic impedance mismatch The greater the acoustic impedance mismatch, the greater the reflected component that occurs Scattering When an interface is equivalent to one wavelength in size, the reflected echoes are scattered in all directions More scattering will be noted at higher frequencies Commonly be seen in organ parenchyma e.g. liver Beam Divergence When the ultrasound beam diverges, the intensity of the beam will be reduced Divergence will be most notable in the far field of a non-focused transducer Lecture 3 Generation of Ultrasound Lecture Objectives I. Demonstrate the anatomy of ultrasound scanner II. Identify the components and characteristics of ultrasound Transducer III. Explain Piezoelectric and reverse or Converse Piezoelectric Effect IV. Display different modes of ultrasound machine ULTRASOUND : How is it produced ? Produced by passing an electrical current through a piezoelectrical material in the transducer. Ultrasound Generation : Piezoelectrical Effect: This is the ability of a material to generate an electrical charge in response to applied pressure Materials that expand and contract with current Ultrasound Generation These materials convert electrical energy into a mechanical ultrasound wave Reflected echoes return to the scan head which converts the ultrasound wave into an electrical signal Principles of Image Information : Pulse-Echo in tissue: Ultrasound pulse is launched into the first tissue At tissue interface a portion of ultrasound signal is transmitted into the second tissue and a portion is reflected within the first tissue (termed an echo) Echo signal is detected by the transducer Piezoelectric material A material that converts electrical energy into mechanical energy (Reverse Piezoelectric Effect) and vice versa (Piezoelectric Effect). Types of piezoelectric materials. Naturally occurring - quartz. Artificial - Lead zirconate titanate PZT materials can be degraded by – Mechanical damage, such a dropping – Heating – Exceeding the electrical limits set by the manufacture Piezoelectric Effect ❖ Is the formation of an electrical charge on the surfaces of the crystal when (mechanical energy) is applied. Reverse Piezoelectric Effect ❖ Is the production of ultrasound (mechanical energy) when an electrical signal is applied to the crystal. Ultrasound Production : Transducer contains piezoelectric elements/crystals which produce the ultrasound pulses by converting electrical energy into a mechanical ultrasound wave. Microscopic view of transducer The Returning Echo : Reflected ultrasound energy (echoes) return to the transducer where the piezoelectric elements convert the ultrasound wave back into an electrical signal. The electrical signal is then processed by the ultrasound system Piezoelectric Crystals : ❖ The thickness of the crystal determines the frequency of the transducer. Frequency Vs. Resolution : The frequency affects the QUALITY of the ultrasound image The HIGHER the frequency, the BETTER the resolution The LOWER the frequency, the LESS the resolution. Frequency Vs. Penetration : The HIGHER the frequency, the LESS the penetration The LOWER the frequency, the DEEPER the penetration CW : A transducer working or operated in CW mode must have two crystal groups: one crystal group is used for transmitting sound waves and the other for receiving echoes returning to the transducer. Continuous-wave output : Pulsed Echo : Pulsed transducers generate multiple, sequential, short pulses of ultrasound waves. Between successive pulses, the transducer is in reception mode and listening for echoes returning to the transducer. A machine operated in pulsed mode uses the same crystal or crystal group for sound transmission and echo reception. Pulsed wave output : Ultrasound Scanner : Ultrasound Image Formation : ❖ Transducer has dual function: (1%) of time Transmit( sending US waves) (99%) time Receives reflected echoes ❖ Main principle of US image is the reflection of US waves from surfaces in the path of the beam. ❖ These reflected echoes return to the transducer where the piezoelectric elements convert the ultrasound wave back into an electrical signal. Ultrasound Image Formation : ❖ Electrical signal produces ‘dots’ on the screen. ❖ Brightness of the dots is proportional to the strength of the returning echoes ❖ Location of the dots is determined by travel time. Distance = Velocity Time The Ultrasound Machine : A basic ultrasound machine has the following parts: Transducer probe Central processing unit (CPU) Transducer pulse controls Display Keyboard/cursor Disk storage device Printer Central processing unit (CPU) : The CPU is the brain of the ultrasound machine, it’s basically a computer that contains microprocessor, memory, amplifiers and power supplies for the microprocessor and transducer probe. The CPU sends electrical currents to the transducer probe to emit sound waves, and also receives the electrical pulses from the probes that were created from the returning echoes. The CPU does all of the calculations involved in processing the data. Once the raw data are processed, the CPU forms the image on the monitor. The CPU can also store the processed data and/or image on disk. Transducer pulse controls : The transducer pulse controls allow the operator to set and change the frequency and duration of the ultrasound pulses from the transducer probe, as well as the scan mode of the machine. The commands from the operator are translated into changing electric currents that are applied to the piezoelectric crystals in the transducer probe. The Ultrasound Machine : Display: Displays the image from the ultrasound data processed by the CPU data processed may be displayed in one of several forms. The primary display forms are A- mode, B-mode, and M-mode. Keyboard : Inputs data (eg. Patient data) and takes measurements from the display Disk storage device: (hard, floppy, CD) - stores the acquired images Printer - prints a hard copy of the image from the displayed data Lecture 4 Ultrasound machine Ultrasound transducers Lecture Objectives I. Identify the main types of ultrasound Transducer & Transducer frequency II. Identify the components and characteristics of ultrasound Transducer III. Explain Probe orientation principles IV. Teach how to understand US image reading principles Ultrasound transducers : It is a device that used to convert one form of energy to another, in this case electrical energy into mechanical energy and vice versa Basic Components of the transducer: 1. Physical Housing: made of plastic material and provides the necessary structural support and acts as an electrical and acoustic insulator Its shape depends on transducer type 2. Electrical Connections: Two electrical connections are formed on the front and back face of the crystal by plating a thin film of gold or silver on these surfaces. These electrodes are connected to the ultrasound machine which generates the short burst of electrical pulses to excite the crystal and through the piezoelectric effect generates a pulse of ultrasound energy 3. Piezoelectric crystal: For maximum efficiency the crystal should be operating at its natural or resonant frequency. This occurs when the thickness of the crystal is half a wavelength (λ/2) 4. Backing (Damping) Material: The backing material is located behind the piezoelectric element to prevent excessive vibration Reducing excessive vibration will cause the element to generate ultrasonic waves with a shorter SPL, improving axial resolution in images. However, this reduces the sensitivity of the transducer to detect weak echoes 5. Matching layers: These are interfaced between the crystals and the patient to reduce the acoustical impedance mismatch between the patient and the transducer A high acoustical impedance mismatch would create a strong reverberation artifact 6. Insulation ring (side wall insulator): It is made of the same damping material. Its function is to absorb energy generated from the sides of the crystal 7. Tuning coil: It is a capacitive device. Its function is to offset the capacitive effect of the crystal so as to improve transmitting and receiving function 8. Electrical shield: Its function is to pick up the unwanted signals after the conversion of ultrasound echoes Types of ultrasound probes : Which probe to pick? ✓ FOOTPRINT : the portion of the transducer that contacts the patient Surface area of skin. ✓ Frequency of emitted sound wave Types of transducers : 1- Linear array 2- Curved array 3- Sector/phased array Types of Electronic Array Transducer : 1. Linear Array Transducers: Elements linearly arranged, and sequentially activated to produce an image Rectangular FOV Used to image superficial structures and vessels This probe has higher frequencies (5–20 MHz) Major applications: Small part , musculoskeletal—tendons, muscles ,testicular,breast and appendicitis in thin patients Linear Array Transducers : Types of Electronic Array Transducer : 2. Curvilinear or (sector) Transducers: Similar to a linear array but the transducer face is convex in shape Trapezoidal FOV Used to image deep lying structures, their main applications are in abdominal and obstetric scanning Typically operate at frequency around 3.5 MHz ž Major applications: ž Aorta, gyn, card, renal Curvilinear or (sector) Transducers : Types of Electronic Array Transducer : 3. Phased Array Transducers: Sector or pie-shaped FOV Smaller than their counterparts and provide a wide FOV to be easily fit between the ribs to obtain an image of the heart Frequency: 1-5 MHz Unlike others, all elements are activated nearly simultaneously to produce a single ultrasound beam Major applications: echocardiography (small to fit between ribs) Phased Array Transducers : Endocavitary probes : The endocavitary transducers include endovaginal, endorectal, and endocavity transducers. These probes provide the opportunity to perform internal examinations of the patient. Designed to fit in specific body orifices. They have small footprints and the frequency varies in the range of 3.5Mhz – 11.5Mhz. Endocavitary probes : Some applications for Endocavitary probes are: The endovaginal or transvaginal probe. Major applications: pelvic ultrasound to examine female reproductive organs. This includes the uterus, fallopian tubes, ovaries, cervix, and vagina. The transesophageal (TEE) probe: Major applications: In cardiology to obtain a better image of the heart through the oesophagus. Endocavitary probes : Probe orientation : One of the first principles to remember is that every probe has a raised marker or indentation on it that correlates to the side of the screen with a dot. Objects located near the probe marker on the transducer will appear near the screen marker on the screen. Objects opposite the probe marker will appear on the other side from the screen marker. Probe positioning when scanning: When obtaining a longitudinal or sagittal view, the transducer is oriented along the long axis of the patient’s body (i.e., the probe marker is pointed toward the patient’s head). This means that you will see the cephalad structures on the side of the screen with the marker (here, on the left side). Probe positioning when scanning : The transverse or axial view is obtained by orienting the transducer 90 degrees from the long axis of the patient’s body, producing a cross-sectional display. For the vast majority of indications, the probe marker should be oriented toward the patient’s right. Again, if the marker is pointed to the right, the structures on the right side of the body will appear on the side of the screen with the marker. Probe positioning when scanning : The coronal view is obtained by positioning the transducer laterally. The probe marker is still pointed to the patient’s head so the cephalad structures are on the left side of the screen (marker side). The structures closest to the probe are shown at the top of the screen, and as the beam penetrates, the tissues furthest from the probe are at the bottom of the screen. Types of Ultrasound Transducers: Scanning Skills : Select the right transducer Ensure correct orientation Try different windows A lot of gel Transducer Manipulation (movements) – Rotate ,sliding, tilting, rocking, and compression Ultrasound Sectional Views – Longitudinal view – Transverse view – Coronal view Transducer Manipulations : The basic TAS transducer manipulations are sliding, rocking, Sliding - is done to move the TAS probe to find the best window or to move to a different area of the pelvis or abdomen Rocking - the probe in a given plane of section allows centering of an organ, structure, or area of interest. Tilting – or angling the transducer from side-to-side allows oblique planes in the same axis to come into view without sliding the transducer over the area of Interest Transducer Manipulations : Rotating - the transducer between 12 and 9 o’clock (90o clockwise turn) Compression - For normal scanning, light transducer pressure should be: applied to minimize patient discomfort associated with a full bladder. Reasonable transducer compression may be used to assess tissue response, separate structures, push gas out of the field-of-view, or compress adipose tissue. Transducer Movements : Ultrasound Sectional Views :