ATAR Physics Unit 4 Topic 3: The Standard Model PDF
Document Details
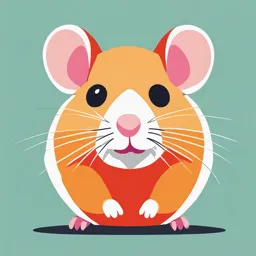
Uploaded by FoolproofLute
Tags
Summary
This document provides teacher notes on the Standard Model in ATAR Physics, Unit 4, Topic 3. It covers fundamental concepts such as elementary particles, quarks, leptons, and particle interactions, providing definitions and explanations.
Full Transcript
ATAR Physics Unit 4: Topic 3 The Standard Model 4.3.1 The Standard Model 4.3.1.1 define the concept of an elementary particle and antiparticle 4.3.1.2 recall the six types of quarks 4.3.1.3 define the terms baryon and...
ATAR Physics Unit 4: Topic 3 The Standard Model 4.3.1 The Standard Model 4.3.1.1 define the concept of an elementary particle and antiparticle 4.3.1.2 recall the six types of quarks 4.3.1.3 define the terms baryon and meson 4.3.1.4 recall the six types of leptons 4.3.1.5 recall the four gauge bosons 4.3.1.6 describe the strong nuclear, weak nuclear and electromagnetic forces in terms of the gauge bosons 4.3.1.7 contrast the fundamental forces experienced by quarks and leptons 4.3.2 Particle interactions 4.3.2.1 define the concept of lepton number and baryon number 4.3.2.2 recall the conservation of lepton number and baryon number in particle interaction 4.3.2.3 explain the following interactions of particles using Feynman diagrams - electron and electron - electron and positron - a neutron decaying into a proton 4.3.2.4 describe the significance of symmetry in particle interactions. 1 4.3.1 The Standard Model 4.3.1.1 define the concept of an elementary particle and antiparticle 4.3.1.2 recall the six types of quarks 4.3.1.3 define the terms baryon and meson 4.3.1.4 recall the six types of leptons 4.3.1.5 recall the four gauge bosons 4.3.1.6 describe the strong nuclear, weak nuclear and electromagnetic forces in terms of the gauge bosons 4.3.1.7 contrast the fundamental forces experienced by quarks and leptons Elementary particles Elementary particles are the smallest known building blocks of the universe. They are subatomic and thought to have no internal structure, meaning that researchers think about them as zero-dimensional points that take up no space. Particles currently thought to be elementary include the fundamental fermions that make up all matter and anti- matter in the universe as well as bosons that mediate the fundamental force interactions among fermions. A particle containing two or more elementary particles is a composite particle. Matter and anti-matter: All matter particles have a corresponding anti-matter particle typically written with a line above the symbol. They share the same mass but have opposite electric charge and quantum numbers. When matter and its corresponding anti-matter particles collide, they mutually annihilate each other and release other subatomic particles in the process. 2 The Standard Model The Mathematical Model we use to describe all elementary particles and their interactions is known as the Standard Model. When studying these elementary particles and their interactions, we use their Quantum Numbers to guide us: 1. Charge (relative to 𝟏𝒆 = 𝟏. 𝟔 × 𝟏𝟎 𝟏𝟗 𝑪) 2. Spin 3. Baryon Number 4. Colour Charge For example, a proton has a Charge of +𝟏𝒆 and based on this we can see that two Up Quarks and one Down Quark can combine to give us this particle: 𝟐 𝟐 𝟏 𝟏𝒆 = 𝒆+ 𝒆− 𝒆 𝟑 𝟑 𝟑 We will look at this further in the next section. 3 Fermions The 12 Fermions of the Standard Model make up all matter in the universe. They are split up into Quarks (pronounced “quorks”) and Leptons. The generation refers to their relative size and mass compared to the first generation. Quarks: Quarks are the subatomic particles that combine to create larger particles that interact via the Strong Nuclear Force (SNF). There are six types of Quarks, known as the six flavours: 1. Up 2. Down 3. Charm 4. Strange 5. Top 6. Bottom 𝟐 𝟏 The charge of each Quark is either + 𝟑 𝒆 or − 𝟑 𝒆 and these are used to help us determine the possible combinations that exist. These combinations are called Hadrons of which there are two types: 1. Baryons (3 Quarks) 2. Mesons (2 Quarks) Colour Charge: When determining whether a possible combination of Quarks can exist, we use a rule known as Colour Charge. We assign the colours red, green and blue for quarks, and anti-red, anti-green and anti-blue for anti-quarks. To be a possible Hadron, the colour charge must combine to create white. Baryons: Baryons are composite particles made up of a combination of 3 Quarks. The most common Baryons are Protons and Neutrons, formed by the combination of Up and Down Quarks. Baryon Number: 𝟏 Each Quark has their own Baryon Number of + 𝟑 which help us determine whether the possible combination can exist because they must add up to 1. Anti-quarks have a Baryon 𝟏 Number of − such that Anti-baryons must add up to -1. 𝟑 4 Mesons: Mesons are made up of two Quarks and are very unstable and short-lived. This is because to satisfy colour charge, the only combinations can be quarks and anti-quarks. Since matter and anti-matter annihilate, this accounts for the short-lived life of Mesons. i) Pion-plus 𝝅 particle ii) Pion-minus 𝝅 particle iii) Kaon-plus 𝒌 particle iv) Cannot exist as this pair will annihilate v) Green and yellow do not make white – colour charge is violated vi) Blue and light blue do not make white – colour is violated Leptons: The second type of Fermion are the Leptons which unlike Quarks exist on their own and interact via the Weak Nuclear Force (WNF). The most familiar Lepton is the electron, but it has two heavier “cousins” called the muon and tau particles. Each of these three have a corresponding neutrino that work in tandem with their Lepton in various physical interactions. For example, beta-minus radioactive decay (Unit 1) is the loss of an electron and anti-neutrino. 5 Worked Example 13.2.1: Electromagnetic and Colour Charge in Baryons Exercises Pearson: Chapter Review 13.1 & 13.2 6 Gauge Bosons There are four fundamental forces of the universe that describe how all matter interact with each other. When physicists discovered the Photon, the electromagnetic particle, scientists realised these forces interact via the exchange of an elementary particle. These are the four Gauge Bosons that exist on the Standard Model. For example, we know electrons repel each other due to the electromagnetic force, but if we could zoom in on this interaction, we would see that this occurs because both electrons release a photon towards the other such that it collides and repels the opposing electron. Due to this, every interaction of the fundamental forces (bar gravity) can be described by the exchange of a Gauge Boson. 1. Gluon (g) The gluon carries the Strong Nuclear Force which acts between Quarks to hold nucleons together. 2. Photon (𝜸) The photon carries the Electromagnetic Force which acts between any fermion with charge. 3. Z & W Bosons These set of Gauge Bosons carry the Weak Nuclear Force, responsible for the radioactive decay of quarks and hadrons. There is No Quantum explanation for Gravity, but scientists have given it a theoretical Gauge Boson called the Graviton. The unification of Quantum Physics and General Relativity remains the biggest problem in Physics and until a Unified Theory is developed, will remain so. Exercises Pearson: Chapter Review 13.3 7 4.3.2 Particle Interactions 4.3.2.1 define the concept of lepton number and baryon number 4.3.2.2 recall the conservation of lepton number and baryon number in particle interaction 4.3.2.3 explain the following interactions of particles using Feynman diagrams - electron and electron - electron and positron - a neutron decaying into a proton 4.3.2.4 describe the significance of symmetry in particle interactions. Particle interactions The interactions of the elementary particles of the Standard Model have produced the universe as we know it. Physicists study the interactions of these particles and their behaviour by analysing their Quantum Numbers with a set of rules and tools. Conservation Laws Just like chemical and nuclear reactions, Quantum Interactions observe a set of Conservation Laws too. 1. Mass – energy and momentum conservation 2. Charge conservation 3. Baryon and Lepton number conservations Mass – energy and momentum conservation: Just like any other interaction in physics, Mass – energy and momentum must be conserved to satisfy the Laws of Conservation of Energy and Momentum. On the fundamental scale, when Matter and Anti-matter collide, we know they annihilate each other and release a burst of energy according to 𝑬 = 𝒎𝒄𝟐. For example, when an electron and positron collide, they release energy in the form of a photon. One of the quirks of quantum mechanics is that the reverse process can also take place, known as Pair- production, whereby a photon can spontaneously create an electron – position pair. This happens because of the conservation of energy which says it cannot be created or destroyed. To account for the annihilation of electrons from the universe, photons must be able to replace them through this process. Charge conservation: Just like nuclear reactions, charge must also be conserved in particle interactions. If we consider the decay of a proton into a neutron, the reason a beta- positive particle is released is to ensure charge is conserved. 8 Baryon and Lepton number conservation: The third important conservation law has to do with Baryon and Lepton number, which must be conserved during particle interactions. In other words, you cannot have a discrepancy of Baryons and Leptons before and after a particle interaction. Neutron Decay: As an example, let’s have a look at these conservation laws in action when a Neutron decays into a Proton: Mass-energy Conservation: 𝟗𝟑𝟗. 𝟓𝟕 → 𝟗𝟑𝟖. 𝟐𝟕 + 𝟎. 𝟓𝟏𝟏 + 𝟎 + 𝟎. 𝟕𝟗 Charge Conservation: 𝟎𝒆 → 𝟏𝒆 + −𝟏𝒆 + 𝟎 + 𝟎 Baryon number Conservation: 𝟏 → 𝟏+𝟎+𝟎+𝟎 Lepton number Conservation: 𝟎 → 𝟎 + 𝟏 + −𝟏 + 𝟎 Worked Example 13.4.2: Conservation in Particle Interactions 9 Feynman Diagrams A useful visualisation of particle interactions are the graphs called Feynman Diagrams. Conventions: 1. Represent space on the vertical axis and time on the horizontal axis (you may see variations of this with the axes flipped around). 2. Represent particles as straight-line arrows in the direction of time, with a letter indicating the type of particle represented. 3. Represent antiparticle arrows pointing in the opposite direction to time. 4. Represent bosons as wiggly lines, with a letter indicating the type of boson represented. There are three Feynman diagrams you need to know: 1. Electron and electron interaction 10 2. Electron and positron interaction Bhabha Scattering 3. Neutron decaying into a proton 11 Symmetry Symmetry is a very important principle in Physics because it allows us to predict what is possible to occur and predict the existence of new particles. The three main Symmetries in Physics are: 1. Charge Reversal: All particles in an interaction can be converted into their antiparticle pairs and the interaction remains valid. 2. Parity Symmetry: Movements and signs of particles can be made negative and the laws of physics still hold true. In other words, a mirror image behaves the same way as a real object. 3. Time Reversal: Particle interactions that occur backwards in time are as equally true as those that occur in forward time. The implication of CPT symmetry is that a "mirror-image" of our universe — with all objects having their positions reflected through an arbitrary point (parity symmetry), all momenta reversed (time reversal) and with all matter replaced by antimatter (charge reversal) — would evolve under exactly the same physical laws that allowed our universe to develop. The CPT transformation turns our universe into its "mirror image" and vice versa. Supersymmetry: Supersymmetry is an extension of the Standard Model that aims to fill some of the gaps. It predicts a partner particle for each particle in the Standard Model. These new particles would solve a major problem with the Standard Model – fixing the mass of the Higgs boson. Exercises Pearson: Chapter Review 13.4 12 13