Trends in Kinase Drug Discovery PDF
Document Details
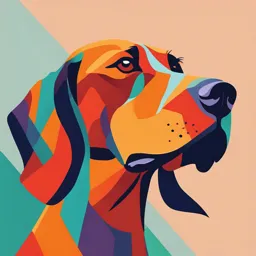
Uploaded by PrudentRainforest
null
Misty M. Attwood, Doriano Fabbro, Aleksandr V. Sokolov, Stefan Knapp, and Helgi B. Schiöth
Tags
Summary
This review article analyzes the landscape of approved and investigational kinase inhibitors. It discusses the trends in kinase inhibitor design, including allosteric and covalent inhibitors, bifunctional inhibitors, and chemical degraders, and the expanding range of indications for kinase inhibitors, including oncology and rheumatoid arthritis.
Full Transcript
Reviews Trends in kinase drug discovery: targets, indications and inhibitor design Misty M. Attwood 1, Doriano Fabbro2, Aleksandr V. Sokolov1, Stefan Knapp and Helgi B. Schiöth1,6 ✉ 3,4,5 Abstract | The FDA approval of imatinib in 2001 was a breakthrough in molecularly targeted cancer therapy and...
Reviews Trends in kinase drug discovery: targets, indications and inhibitor design Misty M. Attwood 1, Doriano Fabbro2, Aleksandr V. Sokolov1, Stefan Knapp and Helgi B. Schiöth1,6 ✉ 3,4,5 Abstract | The FDA approval of imatinib in 2001 was a breakthrough in molecularly targeted cancer therapy and heralded the emergence of kinase inhibitors as a key drug class in the oncology area and beyond. Twenty years on, this article analyses the landscape of approved and investigational therapies that target kinases and trends within it, including the most popular targets of kinase inhibitors and their expanding range of indications. There are currently 71 small-molecule kinase inhibitors (SMKIs) approved by the FDA and an additional 16 SMKIs approved by other regulatory agencies. Although oncology is still the predominant area for their application, there have been important approvals for indications such as rheumatoid arthritis, and one-third of the SMKIs in clinical development address disorders beyond oncology. Information on clinical trials of SMKIs reveals that approximately 110 novel kinases are currently being explored as targets, which together with the approximately 45 targets of approved kinase inhibitors represent only about 30% of the human kinome, indicating that there are still substantial unexplored opportunities for this drug class. We also discuss trends in kinase inhibitor design, including the development of allosteric and covalent inhibitors, bifunctional inhibitors and chemical degraders. Functional Pharmacology, Department of Neuroscience, Uppsala University, Uppsala, Sweden. 1 2 Cellestia Biotech, Basel, Switzerland. 3 Structural Genomics Consortium, Goethe University Frankfurt, Buchmann Institute for Molecular Life Sciences, Frankfurt am Main, Germany. 4 Institute of Pharmaceutical Chemistry, Goethe University Frankfurt, Frankfurt am Main, Germany. 5 German Cancer network DKTK and Frankfurt Cancer Institute (FCI), Goethe University Frankfurt, Frankfurt am Main, Germany. 6 Institute for Translational Medicine and Biotechnology, Sechenov First Moscow State Medical University, Moscow, Russia. ✉e-mail: [email protected] https://doi.org/10.1038/ s41573-021-00252-y Reversible protein phosphorylation mediated by kinases and phosphatases has a key role in regulating cellular functions such as cell proliferation, apoptosis, subcellu lar translocation, inflammation and metabolism1,2. The human kinome is composed of around 560 protein kin ases, including approximately 500 eukaryotic protein kinases (ePKs) that are divided into eight major groups, such as tyrosine kinases, and approximately 60 atypical protein kinases, such as lipid kinases, which have a con served kinase fold like ePKs but differ with regard to other highly conserved sequence motifs of ePKs3,4. Since the 1980s, protein kinases have been recognized as potential drug targets, particularly based on advances in the molecular understanding of cancer, including the discovery of oncogenes such as SRC5. However, the development of protein kinase inhibitors that bind to the ATP site was initially viewed as an unsurmount able challenge because of the high concentration of ATP in the cell, the poor understanding of the regulation of kinase activity and the conserved ATP-binding pocket. Nevertheless, the natural compound staurosporine6 was pursued because it had potent kinase inhibitory activi ties, albeit with poor selectivity. By the end of the 1980s, this paved the way to identify and optimize synthetic small-molecule kinase inhibitors (SMKIs) directed at the ATP-binding site with suitable drug-like properties, selectivity and potency7–9. NATure RevIeWS | Drug DiSCovEry In parallel with such efforts in the 1990s, two kinase inhibitors received regulatory approval. Fasudil, an inhib itor of Rho-associated coiled-coil-containing protein kinases 1 and 2 (ROCK1 and ROCK2) was approved in Japan in 1995 for the treatment of cerebral vasospasm, although the ROCK-inhibitory activity of fasudil was not described until 1997. Sirolimus, a natural product that had a key role in the discovery of mechanistic target of rapamycin (mTOR)10–13, became the first kinase inhibitor to reach the market in the United States in 1999, when it was approved by the FDA for the prevention of organ rejection14, and, in retrospect, was the first allosteric kinase inhibitor. Given the history of these two drugs, imatinib (CGP 57148, STI571) — which was discovered in the labs of Ciba-Geigy in 1992 (ref.15) and approved by the FDA in 2001 — is perceived as a pivotal land mark in the development of kinase inhibitors. Imatinib, which potently inhibits several tyrosine kinases including BCR–ABL and the platelet-derived growth factor recep tor (PDGFR), was initially approved for the treatment of BCR–ABL-driven chronic myelogenous leukaemia (CML) and subsequently for other indications such as the treatment of KIT-driven gastrointestinal tumours (GISTs) (reviewed in ref.16). Its remarkable clinical impact was a catalyst in the surge of activity in the field of molecularly targeted therapy in oncology, in which kinase inhibitors have continued to be prominent in the past two decades. volume 20 | November 2021 | 839 0123456789();: Reviews Box 1 | Data collection and curation The agents that were identified as approved for marketing by the FDA were verified in the US registry of approved drugs at Drugs@FDA: FDA-Approved Drugs. Drugs that were approved by other regulating bodies were identified from the literature and press releases. The dataset of approved drugs that target kinases is provided in Supplementary Table 1. The US NIH database ClinicalTrials.gov is the primary resource for clinical trial registration and contains more than 360,000 research studies within the United States and 219 countries worldwide. ClinicalTrials.gov is an agent-centric resource and hence a priori knowledge of the agents to be investigated and obtain information about is required. We used previously published drug–target interaction datasets181,236–238 and literature reviews21,25,239,240 as well as cross-referencing target-centric resources such as the IUPHAR Guide to Pharmacology and the OpenTargets Platform241 and also personal communications (D. Fabbro) to build the dataset of kinase inhibitors in clinical trials. For these already-identified investigative kinase inhibitors, the mechanism of action — that is, the targeted kinases — had already been determined. To obtain information on new kinase inhibitors entering clinical trials, the commercial resource CenterWatch was used as it posts publicly accessible weekly updates on drugs entering clinical trials as well as developments in industry pipelines. However, the molecular targets of the new agents entering clinical trials generally have to be manually verified and this information can be collated from different resources, preferably primary sources including the literature and patent information, and also other resources such as DrugBank242, Chembl, and industry press releases and literature. Information on the clinical trials associated with each of the identified kinase inhibitors in development was obtained through ClinicalTrials.gov. Only agents with trials registered in ClinicalTrials.gov were included in the analysis and figures, although we provide information on additional agents that were found cited in the literature as currently in trials in the Supplementary information. An advantage of our methods is that we have tried to create a unique and extensive dataset that collates agents registered in clinical trials from different sources to obtain as exhaustive a list as possible. However, owing to the dynamic nature of agents in clinical trials, as well as the time-consuming work of manually validating the mechanism of action for agents entering clinical trials, it can be difficult to maintain current drug– target resources for analysis. Hence, although kinase research is developing with many excellently curated resources, there is still difficulty in maintaining a consistent and comprehensive list of agents in clinical trials, and our curated dataset (provided in Supplementary Table 2) is likely to contain some omissions. Therefore, we consider our dataset to be a comprehensive cross-section of kinase inhibitors that have reached clinical trials. Eukaryotic protein kinases (ePKs). There are eight main groups of eukaryotic protein kinases: AGC (protein kinase A, G and C); CaMK (calcium/ calmodulin-dependent kinases); CMGC (cyclin-dependent kinases, MAP kinases, glycogen synthase kinases, CDC-like kinases); TK (tyrosine kinases); STE (homologues of sterile 7); CK1 (casein kinases); TKL (tyrosine kinase like); and the RGC (receptor guanylate cyclase) groups. Recent reviews on kinase inhibitors have described important aspects of kinase drug discovery, including the targeted pathways and protein families3,17,18, FDA-only approved SMKIs19,20, associated disease indications21–23 or structural aspects24,25. However, to our knowledge there is not a recently published comprehensive analysis of kinase inhibitors and kinase targets in clinical trials, which we present here (see Box 1 for details on the origin of the dataset and analysis, and Supplementary Tables 1 and 2 for a curated dataset). We cover trends in both FDA-approved kinase inhibitors and agents in clinical trials, which could substantially expand the proportion of the kinome that is therapeutically targeted. With such opportunities in mind, we also discuss trends and strategies in kinase inhibitor design. Trends for approved kinase inhibitors Since the approval of fasudil in 1995, the number of approved kinase inhibitors worldwide has increased to 98 drugs, 71 of which are SMKIs that have been approved by the FDA (as of May 2021). Remarkably, the number of approved SMKIs has more than doubled in the past 5 years with 37 FDA approvals, and SMKIs con stitute approximately 15% of all novel drug approvals by 840 | November 2021 | volume 20 the FDA from 2016 to 2021. Ten monoclonal antibod ies (mAbs) that target receptor tyrosine kinases (RTKs) have also been approved by the FDA. Sixteen addi tional SMKIs and one mAb have been granted approval by other regulatory agencies (see details in Fig. 1 and Supplementary Table 1). Kinase families and targets for approved drugs. The 71 FDA-approved SMKIs target 21 kinase families that constitute approximately 20% of the kinome, which corresponds with previous estimates of the coverage of protein kinase targets24,26–28. Kinases targeted by a drug that has been FDA-approved (which are typically considered clinically validated by industry standards) include members of families in five of the eight major ePK groups (TK, TKL, STE, CMGC and AGC), one fam ily in the atypical protein kinase group (PIKK) and one family in the lipid kinases (PI3K; phosphatidylinositol 4,5-bisphosphate 3-kinase). Specifically, these 71 SMKIs primarily inhibit approximately 42 proteins within these groups and most of them — 49 SMKIs — predominantly target 30 proteins that are members of 15 families in the TK group (Fig. 2). Although the TK group is the most exploited kinase group, only approximately 30% of it is targeted, indicating that there is still significant room for further exploration. Members of the HER family (HER1–4) of RTKs are the most targeted, with eight FDA-approved SMKIs and eight FDA-approved mAbs that target these pro teins. The vascular endothelial growth factor receptor (VEGFR) family of RTKs has also been extensively tar geted, with seven FDA-approved SMKIs and one mAb, although all of the SMKIs are non-selective inhibitors that also interact with other RTKs. Inhibition of Janus kinases (JAKs) has been another popular therapeutic strategy, and so far five SMKIs that target this family have been approved by the FDA. Approved kinase inhibitors in oncology. There is a large body of clinical evidence that supports the driver role of kinases in cancer owing to their aberrant activation by either translocations or activating mutations29–32. Chromosomal translocations produce fusion proteins with abnormal localization that can be potentially oncogenic33. Identification and characterization of these disease drivers has facilitated the design and approval of molecularly guided cancer therapies, beginning with the pioneering example of imatinib to treat CML driven by the BCR–ABL translocation, which results in a protein with elevated tyrosine kinase activity34. The majority of FDA-approved SMKIs (61; 89%) and all the FDA-approved mAbs that target kinases (ten mAbs) have oncology indications. Following on from imatinib, four further SMKIs that target ABL have been approved so far: nilotinib, dasatinib, bosutinib and ponatinib35,36 (see Box 2 for details on the development of ABL inhibitors). It is noteworthy that treatment of CML with SMKIs has been shown to be long-lasting and that a substantial pro portion of patients with chronic phase CML (up to 40%) did not relapse after cessation of therapy37,38. More than 20% (19 SMKIs and two mAbs) of the approved agents in our dataset have been approved for www.nature.com/nrd 0123456789();: Reviews treatment of non-small-cell lung cancer (NSCLC), which is significant given that lung cancer is the leading cause of cancer death among both men and women, and 80–85% of lung cancers are NSCLC39,40. Approvals for NSCLC illustrate an important trend in kinase inhibitor devel opment in oncology in general, in which identification of the molecular characteristics of tumours enables the development and application of first-generation SMKIs, as well as guiding further drug development to overcome the emergence of resistance41. In fact, next-generation inhibitors that address acquired resistance comprise up to 70% of the drugs that target seven major kinase Alpelisib Entrectinib Erdafitinib Alectinib Fedratinib Cobimetinib Pexidartinib Lenvatinib Necitumumab Fasudil* Sirolimus ROCK Gefitinib Sorafenib mTOR Crizotinib Osimertinib Ruxolitinib Palbociclib Upadacitinib Abemaciclib Zanubrutinib Acalabrutinib Baricitinib Trastuzumab– deruxtecan Vandetanib Afatinib Brigatinib Peficitinib* Vemurafenib Dabrafenib Copanlisib Flumatinib* Icotinib* Ibrutinib Midostaurin Tepotinib Lapatinib Trametinib Neratinib Tivozanib Nilotinib Trastuzumab– emtansine Netarsudil Trilaciclib Temsirolimus RAF • JAK • ALK • BRAF-V600E • MEK • BTK Ribociclib • EGFR-T790M • CDK4/6 Umbralisib FLT3 • CSF1R • FGFR 1995 1998 1999 2001 2003 2004 2005 2006 2007 2009 2011 2012 2013 2014 2015 2016 2017 2018 2019 2020 2021 EGFR/HER BCR–ABL Trastuzumab Imatinib VEGFR PI3K PDGFRA • TRK • SYK • MET mutants • PDGFRA-D842V • RET mutants • KIT-D816V Cetuximab Everolimus Ceritinib Binimetinib Avapritinib Erlotinib Pazopanib Idelalisib Dacomitinib Capmatinib Axitinib Nintedanib Duvelisib Pemigatinib Panitumumab Bosutinib Ramucirumab Encorafenib Pralsetinib Sunitinib Cabozantinib Apatinib* Fostamatinib Ripretinib Pertuzumab Nimotuzumab* Gilteritinib Selpercatinib Ponatinib Ripasudil* Larotrectinib Selumetinib Regorafenib Lorlatinib Tucatinib Tofacitinib Catequentinib* Tirbanibulin Radotinib* Fruquintinib* Margetuximab Pyrotinib* Almonertinib* Dasatinib Kinase nomenclature Target family Suffix TK -inib mTOR -limus RAF -rafenib VEGFR -anib MEK -metinib ROCK -dil PI3K -lisib CDK -clib antibody -mab RET Olaratumab Olmutinib* Tirabrutinib* Delgocitinib* Approved for a non-oncology indication Filgotinib* Antibody Orelabrutinib* Fig. 1 | Timeline of approved kinase inhibitors. Beginning with the approval of fasudil in 1995, the timeline indicates the year a novel kinase family became validated (that is, the first year an agent targeting that kinase family was approved) and each of the small-molecule kinase inhibitors (SMKIs) that have been approved. Seventy-one SMKIs have been approved by the FDA; eight further SMKIs have been approved by the National Medical Products Administration (NMPA) in China; five by the Pharmaceuticals and Medical Devices Agency (PMDA) in Japan; one approved solely by the EMA, and two approved by the Ministry of Food and Drug Safety (MFDS) in South Korea (all non-FDA approvals are indicated by NATure RevIeWS | Drug DiSCovEry an asterisk). Eleven monoclonal antibodies have also been approved (indicated by a red square): ten by the FDA and one by the NMPA. Many of the kinase inhibitors have also been approved by the EMA; this timeline prioritizes approval dates from the FDA first. Several of the kinase inhibitors not approved by the FDA have been approved by more than one other regu latory agency, and the first date of approval with the corresponding regulatory agency is emphasized. Drugs that have been approved for non- oncology indications are denoted with a blue square. The suffix nomenclature for kinase inhibitors and monoclonal antibodies is provided in the key. TK, tyrosine kinase. volume 20 | November 2021 | 841 0123456789();: Reviews Tyrosine kinase group JAK * Ruxolitinib * Tofacitinib * Baricitinib * Fedratinib * Upadacitinib RET * Cabozantinib * Pralsetinib * Selpercatinib FLT3 * Gilteritinib * Midostaurin EGFR/HER * Gefitinib * Erlotinib * Tucatinib + Lapatinib ♦ Afatinib ♦ Osimertinib ♦ Neratinib ♦ Dacomitinib VEGFR * Axitinib * Lenvatinib * Pazopanib * Sunitinib * Vandetanib * Nintedanib # Tivozanib ABL * Bosutinib * Dasatinib * Ponatinib # Nilotinib # Imatinib KIT * Avapritinib # Ripretinib BTK ♦ Ibrutinib ♦ Acalabrutinib ♦ Zanubrutinib TRK * Larotrectinib * Entrectinib SYK * Fostamatinib MET * Capmatinib * Tepotinib SRC Tirbanibulin FGFR * Erdafitinib * Pemigatinib Lipid kinases PI3K * Alpelisib * Idelalisib * Copanlisib * Duvelisib * Umbralisib ALK * Crizotinib * Ceritinib * Alectinib * Brigatinib * Lorlatinib CSF1R # Pexidartinib TKL BRAF + Vemurafenib + Dabrafenib + Encorafenib # Regorafenib # Sorafenib Atypical kinases mTOR § Sirolimus § Temsirolimus § Everolimus CK1 PIKK PI3K CMGC CDK4/6 * Palbociclib * Abemaciclib * Ribociclib * Trilaciclib STE MEK1/2 § Trametinib § Cobimetinib § Binimetinib § Selumetinib AGC ROCK1/2 * Netarsudil CAMK Type of inhibitor * + # § ♦ 1 1.5 2 3 Covalent Fig. 2 | FDA-approved kinase inhibitors mapped onto the human kinome. The kinase targets of the 71 FDA-approved small-molecule kinase inhibitors (SMKIs) are mapped onto a phylogenetic representation of the human kinome. The primary kinase targets are identified, although many SMKIs cross-react with other kinases and in reality bind in varying degrees to other kinases. The type of kinase inhibitor is also indicated. Tirbanibulin targets the peptide substrate site of SRC. gene families: NTRK, ABL, ALK, BTK, FLT3, KIT and MET. Next-generation sequencing has become the gold standard in sequencing tumours in NSCLC to detect driver mutations and facilitate comprehensive testing of multiple gene targets in a single assay. This has helped to characterize driver alterations in genes such as EGFR, ALK, ROS1, NTRK and MET, which are now targeted by FDA-approved drugs42,43. The first SMKIs to be approved for NSCLC in the early 2000s, gefitinib and erlotinib, targeted epidermal growth factor receptor (EGFR) based on its important role in tumour growth and progression44. However, mutation-induced drug resistance commonly occurs with SMKIs that target EGFR. In particular, T790M gate keeper mutations arise in up to 50% of patients treated with EGFR inhibitors45. The single-point substitution mutation (L858R) in exon 21 and exon 19 deletions, which are often referred to as ‘classical’ EGFR activat ing mutations as they account for 85–90% of EGFR kinase domain mutations46, are identified as sensitizing mutations associated with different clinico-pathological features47,48. Exon 20 frame insertions are also activating mutations; however, they are not inherently sensitive to 842 | November 2021 | volume 20 EGFR inhibitors and have been associated with poor patient prognosis49. Next-generation covalent SMKIs including afatinib and dacomitinib that are active against mutations such as T790M have been approved by the FDA, and the third-generation inhibitor osimertinib, which selectively targets activating mutations as well as the T790M resistance mutation, has also been approved50. Rearrangements in ALK, which produce an abnor mal ALK protein that promotes cellular proliferation and migration, are identified in approximately 5% of cases of NSCLC, and the first ALK inhibitor, crizotinib, was approved in 2011 (ref.51). ROS1, which is closely related to ALK, is also targeted by ALK inhibitors, and ROS1 rear rangements have an incidence of approximately 1–2% in NSCLC52. Furthermore, acquired ROS1 resistance mutations occur in up to 60% of crizotinib-refractory patients53. Second- and third-generation compounds have been developed to improve on crizotinib’s thera peutic characteristics and combat resistance mutations. Four additional SMKIs that target ALK and/or ROS1 translocations have since been approved by the FDA: ceritinib, alectinib, brigatinib and lorlatinib54. Recently, four further SMKIs that target disease-related mutations www.nature.com/nrd 0123456789();: Reviews that affect specific kinases have been approved for NSCLC: selpercatinib and pralsetinib for the treatment of RET-fusion-positive NSCLC; and capmatinib and Box 2 | Developing multiple generations of ABL kinase inhibitors Imatinib revolutionized the treatment of chronic myelogenous leukaemia (CML) and provided proof for the concept that small molecules that specifically target molecular drivers of cancer, such as the mutant BCR–ABL kinase in CML, can provide effective therapy. Various efforts have been made to develop second- and third-generation ABL inhibitors that could overcome the intrinsic clinical resistance of CML to imatinib due to mutations in the kinase domain of ABL (including the gatekeeper mutation T315I)109,243,244. One approach has been structure-based drug design, enabled by the elucidation of the imatinib–ABL co-crystal structure, to improve the molecular recognition of inhibitors in the ATP-binding site, which resulted in the approval of nilotinib and ponatinib245–249. A second approach in which compounds were screened against both wild-type and mutant BCR–ABL led to the approval of dasatinib and bosutinib as broad-spectrum BCR–ABL inhibitors. However, except for ponatinib, none of these second-generation ATP-binding-site-directed BCR–ABL inhibitors was able to inhibit the gatekeeper T315I mutant. A phenotypic screen using BCR–ABL-transformed cell lines resulted in the discovery of GNF-2, which seemed not to be directed to the ATP-binding site of ABL, as it was unable to inhibit enzymatic ABL kinase activity when the kinase domain only of ABL was used250. The structure of ABL with its regulatory SH2 and SH3 domains lacking the last exon showed that the N terminus of ABL was myristoylated and that myristate binding into a cylindrical pocket located at the C terminus of the kinase domain (the myr pocket)244,245 led to an auto-inhibited conformation136,137. However, when BCR is fused to the N terminus of ABL, the N-terminal myristoyl is lost, which results in the constitutive ABL kinase activity of BCR–ABL136,137. The binding of GNF-2 into the myr pocket was demonstrated by X-ray crystallography and NMR136. In addition, binding of GNF-2 to the myr pocket was disrupted by point mutations that also conferred resistance to the ability of GNF-2 to inhibit cellular BCR–ABL activity251,252. Further structural and experimental analyses indicated that GNF-2 could allosterically inhibit BCR–ABL kinase activity, even with the recalcitrant T315I gatekeeper mutation in ABL245. This led to a search for second-generation myr-pocket binders (third-generation ABL inhibitors), resulting in the discovery of ABL001 (asciminib; also known as STAMP (specifically targeting the ABL myristoyl pocket)), which can induce an auto-inhibited conformation of even the ABL-T315I mutant245,251. The improved potency of asciminib against wild-type ABL and ABL-T315I translated into a high degree of synergistic activity with ATP-site-directed inhibitors against cells transformed with BCR–ABL-T315I136. This inhibitor is among the most potent and specific ABL kinase inhibitors known to date. Asciminib has been tested in clinical trials involving heavily pretreated patients with CML who had resistance to or unacceptable side effects from other kinase inhibitors, including patients in whom ponatinib had failed and those with a T315I mutation. A phase III trial met its primary end point, showing that treatment with asciminib resulted in a higher rate of major molecular responses than bosutinib253, and a regulatory submission for asciminib is expected in 2021. The development of BCR–ABL kinase inhibitors illustrates some general lessons for kinase drug discovery. First, allosteric kinase inhibitors can be very selective; asciminib is one of the most selective kinase inhibitors among the synthetic SMKIs. Second, biochemical assays to search for kinase inhibitors are best done with native and full-length proteins if possible to have access to diverse regulatory interactions. Third, understanding the mechanism of action of kinase inhibitors requires time and labour-intensive commitment to medicinal chemistry, pharmacology, biochemistry, biophysics, and cell and structural biology. It took 13 years from the approval of imatinib to the phase I studies with asciminib. Finally, it is important to understand the disease that is being treated by kinase inhibitor drugs. Imatinib and the other inhibitors that target ABL for the treatment of CML still stand out in terms of their unprecedented efficacy compared with many kinase inhibitors developed for other cancers in the past two decades. This may reflect that imatinib and the other BCR–ABL inhibitors are conceptually closer to preventing cancer, namely the acute phase of CML (blast crisis) rather than treating a cancer, which may often be at an advanced stage. Indeed, the chronic phase of CML, in particular the early phase, could be viewed as a myeloproliferative disorder rather than a cancer. Many myeloproliferative disorders are driven by tyrosine kinases, for example, polycythemia vera (JAK2), chronic myelomonocytic leukaemia (PDGFR) and hypereosinophilic syndrome (PDGFR), although they rarely turn into cancer, unlike CML. NATure RevIeWS | Drug DiSCovEry tepotinib for the treatment of MET-mutation-positive NSCLC55–58. Targeting tumour angiogenesis is an important anti cancer strategy, and many approved kinase inhibitors have successfully targeted the angiogenic pathways driven by VEGFR, PDGFR, KIT, fibroblast growth factor receptors (FGFRs) and MET for different indi cations. For example, there are five FDA-approved SMKIs approved for renal cell carcinoma that inhibit VEGFR as well as other RTKs, and also two approved rapalogue inhibitors, temsirolimus and everolimus, that target mTOR59, which is downstream in the VEGFR signalling pathway 60. Metastatic colorectal cancer (CRC) has also been treated via VEGFR inhibition with the FDA-approved mAb ramucirumab and the SMKI fruquintinib, which is approved in China. Medullary thyroid carcinoma has been addressed by the VEGFR inhibitors vandetanib, cabozantinib and lenvantinib, as well as the previously mentioned RET inhibitors pralsetinib and selpercatinib. PDGFR and KIT are inhibited by imatinib, and this activity led to its FDA approval for GISTs in 2002 (ref.61). As with other cancers, further SMKIs have been devel oped to improve activity and/or address resistance to first-generation drugs, with two such agents, avapritinib and ripretinib, approved in 2020 that target particular PDGFR and KIT activation loop mutants, which are responsible for relapse of up to 85–90% of patients with GISTs62. Inhibition of the angiogenic FGFR pathway has more recently been validated as a therapeutic strategy, with FDA approval of erdafitinib in 2019 for the treat ment of FGFR-altered advanced urothelial carcinoma and FDA approval of pemigatinib in 2020, which is the first targeted treatment for cholangiocarcinoma, an aggressive tumour characterized by FGFR driver mutations63,64. Other RTK protein families have been targeted by approved kinase inhibitors for various cancer indica tions. Among the most prominent are drugs that target HER2 to treat breast cancer, which include nine of the approved kinase-targeted agents in the dataset. These include the HER2-targeted mAb trastuzumab, which was approved in 1998 and provides a pioneering exam ple of the use of an RTK-targeted agent being guided by companion diagnostics, and the very recently approved HER2-targeted mAb margetuximab, as well as four SMKIs, lapatinib, neratinib, tucatinib and pyrotinib (approved in China) that are also used to treat patients with HER2+ breast cancer65. Two antibody–drug con jugates that target HER2, trastuzumab–emtansine and trastuzumab–deruxtecan, are also included in our data set. Although the cytotoxic payload is a key driver of the anticancer activity of these antibody–drug conjugates rather than inhibition of the activity of HER2 alone, we include them for completeness. Various intracellular kinases have also been targeted by approved SMKIs. Targeting CDK4 and CDK6, which have important roles in cell division, has been so suc cessful that three SMKIs, palbociclib, ribociclib and abemaciclib, are now the standard of care in particular breast cancer indications66. The most recent CDK4/ CDK6 inhibitor, trilaciclib, was approved in 2021 for small cell lung cancer myelopreservation therapy67. volume 20 | November 2021 | 843 0123456789();: Reviews Metastatic melanoma is a challenging disease to treat, and the commonly observed BRAFV600E mutation makes this cancer particularly aggressive. Six SMKIs have been approved for this indication: three agents are BRAF inhibitors (vemurafenib, dabrafenib and encorafenib) and three are MEK inhibitors (trametinib, cobimeti nib and binimetinib)68. Furthermore, the MEK and BRAF combination regimen of dabrafenib plus trametinib was also approved by the FDA in 2018 (ref.69). Additionally, evidence indicates that combinations of targeted ther apy with SMKIs, and particularly MEK and BRAF inhibitors, plus immunotherapy can achieve durable responses with better therapeutic effectiveness70,71. The PI3K–AKT–mTOR pathway is involved in cell survival, metabolism and regulation of cell growth. The dysregulation of this pathway in many cancers23 has led to extensive efforts to target its components. Such efforts have led to the only approved kinase inhibitors that target lipid kinases, beginning with FDA approval of idelalisib, a first-in-class SMKI that targets PI3Kδ, for the treatment of various lymphomas in 2014 (refs72,73). Since then, the dual PI3Kα/β inhibitor copanlisib was approved in 2017 for follicular lymphoma74, the dual PI3Kδ/γ inhibitor duvelisib was approved in 2018 for several lymphomas75 and the PI3Kα-selective inhib itor alpelisib was approved in 2019 for the treatment of breast cancer76. Three SMKIs have been approved that target mTOR (see above), including sirolimus — one of the earliest kinase inhibitors — which has been approved for a variety of indications, both oncological and non-oncological. Substantial progress has been achieved by target ing kinases for the treatment of other haematologi cal malignancies. Notably, mantle cell lymphoma and chronic lymphocytic leukaemia are being addressed by three FDA-approved Bruton tyrosine kinase (BTK) inhibitors25,77: ibrutinib, acalabrutinib and zanubruti nib. Significantly, all three drugs act covalently, and the success of ibrutinib had an important role in catalysing a re-evaluation of covalent inhibition as a drug design strategy (see below), together with afatinib (see above). Finally, kinase inhibitors are also at the forefront of pioneering efforts to develop anticancer drugs based on the molecular characteristics of the tumour, instead of the tissue of origin78. Three decades after the discovery of NTRK1 as a component of an oncogenic fusion gene product in CRC79 and the subsequent discovery of NTRK2 and NTRK3, the pioneering pan-TRK inhibitor laro trectinib became only the second tumour-agnostic ther apy to enter the market (after pembrolizumab) following its FDA approval in 2018 for the treatment of patients with solid tumours that have an NTRK gene fusion80. Since then, it has been joined by the multikinase inhibitor entrectinib, which is also approved for patients with solid tumours that have an NTRK gene fusion81. Other kinase inhibitors are also being investigated in tumour-agnostic development programmes, including the approved RET inhibitors pralsetinib and selpercatinib78. Approved kinase inhibitors beyond oncology. Many kinases are involved in the regulation of immune responses. For instance, JAKs are the key components 844 | November 2021 | volume 20 for T helper cell (TH1, TH2 and TH17) immune responses as they are secondary messengers for many cytokines, including interferons and interleukins 82,83. Eleven SMKIs — more than 13% of the approved SMKIs — have been approved for immune system-related indications, mostly autoimmune and inflammatory disorders. The first kinase inhibitor to be approved outside oncology was ruxolitinib, an inhibitor of JAK1 and JAK2 that was approved by the FDA for the treatment of patients with intermediate or high-risk myelofibro sis in 2011, following the recognition that activating mutations in JAK2 caused a group of myeloprolifera tive neoplasms84. A JAK2 inhibitor, fedratinib, was also approved for such diseases in 2019. Rheumatoid arthritis is the autoimmune disease with the most approved SMKIs (five overall, and three approved by the FDA). All five SMKIs selectively tar get isoforms of JAK, and include the first SMKI to be approved for rheumatoid arthritis — tofacitinib in 2012 — followed by baricitinib, upadacitinib, peficitinib and filgotinib. Peficitinib predominantly inhibits JAK3 and was approved by the Pharmaceuticals and Medical Devices Agency (PMDA) in Japan in 2019. Filgotinib is a selective JAK1 inhibitor that has received approval by the EMA and PMDA; however, the FDA has postponed a decision on the new drug application for it for safety reasons (see Related links). JAK kinase inhibitors are also being investigated for other immune disorders, and delgocitinib, a pan-JAK inhibitor that was approved in 2020 by the PMDA, is the first kinase inhibitor approved for the skin disorder atopic dermatitis. The kinase SYK, which is involved in innate and adaptive immune responses, is implicated in the devel opment of allergic and autoimmune diseases, as well as in haematological malignancies85–87. Fostamitinib, a first-in-class SYK inhibitor, was approved by the FDA in 2018 for chronic immune thrombocytopenia owing to its ability to reduce antibody-mediated destruc tion of platelets88. Rejection prophylaxis for organ transplantation and graft-versus-host disease are also immune-related issues that are amenable to kinase inhi bition. Two agents have been approved for each of these disorders: ibrutinib and ruxolitinib. Finally, several SMKIs have been approved for dis eases beyond oncology and immunology. These include the ROCK1 and ROCK2 inhibitor fasudil for cerebral vasospasm (mentioned above), the mTOR inhibitor everolimus for tuberous sclerosis complex-associated partial-onset seizures89, the VEGFR inhibitor nintedanib for idiopathic pulmonary fibrosis90, the FLT3 inhibi tor midostaurin for advanced systemic mastocytosis91 and another ROCK1/ROCK2 inhibitor, netarsudil, for glaucoma and ocular hypertension92. Classes of agents. Nearly all of the FDA-approved SMKIs are directed towards the kinase ATP-binding site (63 SMKIs), with the exception of three rapalogues, four MEK inhibitors and the dual SRC and tubulin polymer ization inhibitor tirbanibulin, which targets the peptide substrate site93 (see below for further discussion of bind ing modes). The conservation of the ATP-binding site in the human kinome means that ‘ATP mimetics’ often www.nature.com/nrd 0123456789();: Reviews cross-react with many other different kinases, resulting in compounds with promiscuous profiles. Promiscuous compounds such as dasatinib94 or sunitinib95,96 have been termed multikinase inhibitors and can have some toxi cological liabilities97. However, this cross-reactivity can also be potentially advantageous. For instance, the activ ity of imatinib against multiple kinases has enabled its application in multiple indications, such as CML driven by the BCR–ABL translocation, GISTs driven by KIT and hypereosinophilic syndrome driven by PDGFRα deregu lation. Inhibiting multiple nodes in cellular networks by blocking two or more kinases can also have synergistic effects that might be clinically useful; for example, tar geting the BRAF and MEK pathways with the previously mentioned combination of dabrafenib plus trametinib98. The cell surface location of RTKs has been success fully exploited by ten FDA-approved mAbs for the treat ment of breast cancer, CRC, NSCLC, soft tissue sarcoma and hepatocellular cancer. The mechanism of action of such agents is more complex than inhibition of kinase activity by SMKIs, and could include blockade of ligand binding (thereby preventing kinase activation), promot ing receptor internalization and antibody-dependent cellular cytotoxicity99. Trends in kinase inhibitor design The identification of SMKIs has been advanced sub stantially by understanding of the structures of their binding sites. More than 5,500 catalytic domain struc tures have been published so far, covering approximately 300 kinases from 104 families. In this section, we over view the key features relevant to structure-based drug design and trends in the development of SMKIs of different types. Structural features of the kinase catalytic domain. The architecture of the kinase fold was first described in 1991 by Knighton et al.100,101, who reported the structure of protein kinase A (PKA) in complex with ATP–Mg2+ and an inhibitory peptide. This structure revealed a bilobal arrangement including an N-terminal smaller lobe con taining a five-stranded antiparallel β-sheet (β1–β5), the conserved regulatory αC helix, two additional helical segments, as well as a larger C-terminal lobe of mostly α-helices (Fig. 3a). The ATP cofactor was found sandwiched between the two lobes and forming hydrogen bonds between the adenine ring and the hinge region, which established a backbone connecting the lobes. These hydrogen bonds represent important anchor points for the design of ATP mimetic inhibitors and define the basic kinase inhibitor scaffolds. ATP is also coordinated by the glycine-rich loop (G-loop, also called phosphate-binding loop (P-loop)). This is a highly flexible region that is present in the β-sheet structures β1 and β2 with the consensus sequence motif GXGXφG, where φ represents a hydrophobic residue. A second key sequence motif, (V)AxK, is located in β3. A structural hallmark of active kinases is a conserved salt bridge formed between the lysine residue in the (V)AxK motif and a conserved glutamate located in αC positioning αC (‘αC-in’ conformation). The αC helix has NATure RevIeWS | Drug DiSCovEry an important regulatory role, as the (V)AxK salt bridge contributes to the coordination of the phosphate groups of ATP. In inactive kinases, the αC helix dislodges from its position in the active state moving outwards (αC-out) and it often also rotates the central conserved glutamate away from the ATP-binding site. The C terminus of the αC helix in the αC-in state is additionally stabilized by interaction with the con served tripeptide Asp-Phe-Gly motif DFG, which also anchors the N terminus of the activation segment. In its active ‘DFG-in’ conformation, the DFG aspartate residue points towards the phosphate moieties of ATP, coordinating a catalytically important Mg2+ ion. In inac tive kinases, the DFG is mobile, dislodging the phenyla lanine residue. This so-called ‘DFG-out’ conformation opens a deep, mainly hydrophobic binding pocket102 (see below). The DFG-out movement also results in unstruc turing of the activation segment, a mobile structural element of variable length and sequence that harbours the DFG motif, the activation loop (A-loop) and ends with the helical APE (Ala-Pro-Glu) motif. The A-loop also contains phosphorylation sites that regulate kinase activation of kinases that are not constitutively active, by either autophosphorylation or transphosphorylation103. Finally, the catalytic loop harbours a highly conserved Y/HRD (Tyr/His-Arg-Asp) motif with the strictly conserved aspartate, which is required for catalysis. The 3D structure of ePKs is highly conserved, includ ing a network of structurally important hydrophobic residues104. These residues form two spines that in the active state connect and properly align both kinase lobes and position key sequence motifs for catalysis104,105. One spine is called the catalytic spine (C-spine), which is complemented by the adenine ring system of the cofac tor ATP, and connects structural elements at the hinge side of the kinase domain. A second hydrophobic spine called the regulatory spine (R-spine) senses the proper alignment of the β4 sheet, αC, the DFG motif and the lower lobe αE helix when in the active state (Fig. 3b). In inactive states, the displacement of R-spine residues causes the R-spine to be broken. The functional impor tance of spine residues has been validated experimen tally by mutagenesis studies that demonstrated that hydrophobic contact by spine residues is required for proper catalytic function106. Owing to their proximity to the ATP-binding site, many kinase inhibitors inter act with spine residues or even intercalate or alter spine interactions. A residue called the gatekeeper bridges the C-spine and the R-spine (M120 in PKA) and is located at the N terminus of the hinge region. The importance of the gatekeeper residue for drug development was recog nized early107. The presence of a small gatekeeper residue such as threonine, although not common, can enable the design of inhibitors that are selective over other kinases because it makes the back pocket accessible to small mole cules; this means that inhibitors that bind to this pocket are prevented from binding to other kinases that have large hydrophobic residues in the gatekeeper position108. Glycine residues are not present in the gatekeeper posi tion in human kinases, which led to the design of bulky ATP analogues that specifically target kinase gatekeeper volume 20 | November 2021 | 845 0123456789();: Reviews a b β β2 αC β5 β3 β1 A70 Activation segment Mg αC L106 L173 L95 F185 Mg ATP Regulatory spine Adenine (ATP) F54 G-loop Hinge M120 Catalytic spine β4 DFG motif DFG motif F185 L172 αD Y164 αD APE helix αE M128 αG L227 αE M231 αF αF αH c Type 1 d Type 2 αC αC K271 K271 E286 F317 G-loop Hinge G383 Hinge G-loop Y253 F382 F382 D381 3kf4 E286 F317 DFG motif e Type 1.5 DFG motif D381 3kfa f Type 3 αC G-loop K483 αC E501 I141 K97 M219 ATP I216 Hinge H145 D594 Hinge D532 F595 DFG motif Activation segment Mg D208 DFG motif F209 4mne 5csw mutants. However, gatekeeper mutations that shift to bulkier residues that sterically exclude inhibitors are a common cause of kinase drug resistance109. Comprehensive selectivity testing. The development of large panels of kinase assays for screening has ena bled comprehensive assessment of inhibitor selectivity in and outside cells110. The assays are either enzyme kinetic assays, stability-b ased assays111 or displace ment assays using recombinant proteins112 or cellular extracts in combination with mass spectrometry113,114. 846 | November 2021 | volume 20 Recently, assay systems have also been established that allow studies on inhibitor binding to full-length kinases and selectivity in live cells115,116. In particular, besides the well-established potency criteria, kinetic aspects of ligand binding have also emerged as important parameters for ligand optimization117,118. Strategies for rational inhibitor design. The highly dynamic nature of protein kinases allows for the design of inhibitors that recognize the active or diverse inac tive conformations. The inactive state of kinases is www.nature.com/nrd 0123456789();: Reviews ◀ Fig. 3 | Structural features of the kinase catalytic domain and inhibitor binding modes. a | Structural overview of the kinase catalytic domain, based on the structure of protein kinase A (PKA) in ribbon representation235. The main secondary structure elements are labelled in parts a and b. The ATP cofactor is shown in ball and stick representation. Mobile structural elements that regulate kinase activity including the glycine-rich loop (G-loop; blue), the activation segment (turquoise) and αC helix (red) are highlighted. Two Mg2+ ions are shown as solid spheres. b | Aligned catalytic (blue) and regulatory (yellow) spine residues in the PKA active state. The gatekeeper residue (M120, grey) bridges the two spines. The adenine ring of ATP bridges N-terminal and C-terminal lobe catalytic spine residues. The secondary structure elements are coloured in light green for sheets and red for helices in parts c–f. c | Details of the type 1 binding mode in ABL kinase. The binding site boundaries are shown as a transparent surface. Key residues are shown in ball and stick representation. Hydrogen bonds are shown as dotted lines. d | Details of the type 2 binding mode in ABL kinase. Labelling of residues and structural elements is identical to panel c. The large pocket created by the Asp-Phe-Gly motif (DFG)-out movement that is characteristic of this binding mode is highlighted by a dashed circle. e | Details of the type 1.5 binding mode in BRAF kinase complexed with dabrafenib. Binding of the inhibitor breaks the regulatory spine by inducing an αC-out movement. The salt bridge between αC E501 and K483 is not present in this inactive conformation. f | Example of a type 3 inhibitor binding mode in the kinase MEK1. The inhibitor occupies a pocket (dashed circle) created by an αC-out movement and an inactive helical conformation of the activation segment. The cofactor ATP–Mg2+ shown in ball and stick representation binds to the ATP-binding site and the DFG motif is in a DFG-in conformation. APE, Ala-Pro-Glu motif. particularly structurally diverse and has been extensively explored in drug development. More than 80 possible ligand-binding sites that are present in the kinase cata lytic domain have been catalogued and described3,119. A detailed description of this area is beyond the scope of this Review and we restrict our description to the main binding modes that are now well-established in kinase drug discovery (for detailed reviews, see refs25,120). Type 1 inhibitors target the kinase active state (Fig. 3c). The central purine moiety of the inhibitor mimics hinge-binding hydrogen bonds of ATP, and both αC and the DFG motif are in their active ‘in’ position. However, in this structure, the G-loop is not in an active confor mation forming an aromatic stacking interaction with the purine ring and Y253. Aromatic amino acids are fre quently found at the tip of the G-loop. In the ATP-bound active state, these residues are oriented away from the ATP site. Interestingly, so-called ‘folded P-loop confor mations’ have been observed in many kinase inhibitor complexes, and this conformation has been associated with favourable inhibitor selectivity121. Type 2 inhibitors bind to an inactive kinase state, which is characterized by the DFG-out conformation (Fig. 3d). As shown in the figure, the outward movement of the DFG motif creates a large hydrophobic pocket that is targeted by the trifluoromethylphenyl moiety of this inhibitor. The αC helix maintains its active state αC-in position, as indicated by the formation of the canonical salt bridge between (V)AxK K271 and the conserved αC glutamate E286, while the G-loop maintains its folded conformation. Many intermediate binding modes between those of canonical type 1 and type 2 inhibitors exist, and some authors have introduced type 1.5 binding modes. The definition of type 1.5 inhibitors in the kinase literature is not clear, however. A stringent definition could be an inhibitor that disrupts the R-spine, but the term has also been used to describe inhibitors for which bind ing results in any type of structural deviation from the NATure RevIeWS | Drug DiSCovEry ideal active conformation, including abnormal G-loop conformations and auto-inhibitory conformations of the activation segment122. As many of these structural elements are highly mobile, and their conformations may depend on crystal packing, we consider it more useful to limit this binding mode type to inhibitors that disrupt the R-spine without targeting the canonical type 2-specific DFG-out pocket (Fig. 3e). In the BRAF– dabrafenib complex, the αC helix is moved outwards, resulting in a broken R-spine and a broken (V)AxK (K483) αC glutamate (E591) salt bridge, while the DFG motif remains in a DFG-in position123. Non-canonical binding modes represent attractive design strategies, as these compounds are often highly selective compared with canonical type 1 and type 2 inhibitors and have prolonged target residence times. For instance, the EGFR inhibitor lapatinib binds to a DFG-in conformation, but it induces large structural rearrange ments that explain its high selectivity for EGFR124. Similarly, the protein kinase R (PKR)-like endoplasmic reticulum kinase (PERK) inhibitor GSK2606414 and the MET inhibitor SGX523 target an inactive conformation of the activation segment. The ERK1/ERK2 inhibitor SCH772984 specifically interacts with a unique bind ing pocket created by an αC-out and a folded P-loop conformation125–128. The Kinase–Ligand Interaction Fingerprints and Structures (KLIFS) database provides a valuable resource for studies on ligand interactions and the distribution of diverse binding modes across the kinome129. Recent studies have also examined the role of water in ligand design. Early models considered the displacement of water based only on the simplistic view of entropic gain when ligands displace binding-site waters. More refined computational tools now characterize each water molecule seen in structural models in detail to evalu ate the role of water in ligand potency, conformational change and ligand off-rates130,131. Trends in the chemistry of approved kinase inhibitors. The ATP-binding site is the main site targeted by approved SMKIs, accounting for 63 of the 71 FDA- approved drugs. Similar to the ATP adenine ring, ATP mimetic inhibitors are anchored to the kinase hinge backbone by hydrogen bonds. Several scaffolds have been developed that act as hinge binders (Fig. 4). Fused six-membered ring systems, such as (iso)quinoline and quinazoline, which was first established in the develop ment of fasudil and subsequently gefitinib and erlotinib, have been the most frequently used ATP mimetics, with 18 approved SMKIs. The aminopyrimidine and closely related aminopyridine moiety, which was used in imati nib, have also remained frequently used hinge binders, with 17 approved SMKIs. The third group of hinge binder moieties are fused five- and six-membered ring systems such as pyrrolo pyrimidines and pyrrolopyrides, purines, oxoindoles and pyrrolo or imidazo triazines. Structurally, pyra zolo aminopyrimidines and imidazo aminopyrazines are also related to this class of hinge binders, but based on their hinge-binding modes, they should be consid ered as derivatives of aminopyrimidines. Non-aromatic volume 20 | November 2021 | 847 0123456789();: Reviews N Diverse scaffolds (17) N Isoquinoline (3) N H2N N H2N N N N N Pyrazolo and aminopyrimidine N H N 20% 24% Imidazo aminopyrazine (2) Aminopyrimidine (14) N N N H N N Purine (2) N N N N N Pyrrolo and triazine 14% N Imidazo triazine (2) O 7% N H Oxoindole (2) N Amino pyridine (3) N Quinazoline (10) N 7% N H N O NH2 (R) Carboxamides (3) N N N H Pyrrolo pyridine (2) N N H Pyrrolo pyrimidine (5) N Quinoline (5) Fig. 4 | Chemical scaffolds used in approved kinase inhibitors. Chemical structures of the major hinge-binding scaffolds used in approved kinase inhibitors. Shown is the distribution of hinge-binding scaffolds for all ATP mimetic inhibitors (centre) as well as the structures of the most frequently used moieties. The number of approved drugs using each major scaffold is provided in brackets and each chemotype has been linked to the central pie chart by colour and numbering. Hydrogen bond donors and acceptors are indicated by red arrows. hinge-binding motifs are represented by carboxamides. A total of 17 SMKIs harbour diverse hinge-binding scaffolds, but the narrow chemical space explored in the development of two-thirds of the approved SMKIs is astounding. Allosteric inhibitors. Most signalling kinases are expressed in an inactive state that is activated by many different mechanisms, such as structural changes induced by post-translational modification or through interaction with scaffolding proteins or flanking reg ulatory domains. Thus, the key role of kinases reg ulating cellular signalling requires a high degree of catalytic domain plasticity. This characteristic has been widely explored through the development of allosteric inhibitors that prevent kinase activation. Allosteric kinase inhibitors differ in the activation mechanisms that they prevent and they have therefore been classified only by their location of binding. Type 3 inhibitors bind adjacent to the ATP-binding site in a pocket induced by an αC-out conformation and in some cases by an inactive conformation of the A-loop (Fig. 3f). All four clinically approved MEK1/MEK2 inhibitors are examples of type 3 inhibitors that bind to the allosteric back pocket. The DFG is in an active ‘in’ conformation, allowing simultaneous binding of the type 3 inhibitor 848 | November 2021 | volume 20 and ATP. Some type 3 inhibitors also form polar inter actions with the phosphate backbone of ATP, resulting in an ATP-uncompetitive mode of action. However, an increasing number of type 3 inhibitors bind to the canonical back pocket but stabilize a DFG-out con formation. Examples include allosteric inhibitors that target FAK132, TRKA133, PAK1 (ref.134) and IGF1R135. Remarkably, these inhibitors show isoform selectivity against closely related family members. Type 4 inhibitors bind to allosteric sites that are dis tantly located from the ATP-binding site (schematic representations of binding types in Fig. 5). An example of a type 4 inhibitor is the ABL inhibitor GNF-2, which binds to an induced pocket in the C-terminal kinase lobe that also serves as a binding site for lipids such as myristate136 (Box 2). This type 4 inhibitor makes use of the unique ABL activation mechanism: in inactive ABL kinases, a myristoylated residue at the N terminus of ABL binds to the C-lobe binding pocket, which results in the stabilization of a closed inactive conformation137. In the oncogenic fusion protein BCR–ABL, this mecha nism of inactivation is lost owing to the presence of the N-terminal fusion partner BCR, which results in con stitutively active ABL. GNF-2 and the investigational type 4 inhibitor asciminib (ABL001) mimic the binding of myristate and induce an inactive state similar to that www.nature.com/nrd 0123456789();: Reviews observed for the inactive wild-type protein. Interestingly, combining myristate mimetic ABL type 4 inhibi tors with conventional ATP-competitive drugs could reduce the risk of development of drug-resistant mutations138. Several other type 4 inhibitors have entered clin ical testing, including the allosteric AKT1 inhibitor MK-2206 (refs 139,140) , which binds at the interface between the AKT catalytic domain and the pleckstrin homology (PH) domain. The kinase is locked in an inactive conformation when it is targeted and bound by MK-2206. This also prevents recruitment of AKT1 to the plasma membrane, which is a re-localization event that is required for AKT1 activation. A wide range of preclin ical allosteric inhibitors that target diverse binding sites such as the PDK1-interacting fragment (PIF) pocket141 have now been reported120. Allosteric inhibitors also allow selective targeting of mutations, as demonstrated by EAI045, which targets the EGFR resistance mutants EGFR-L858R/T790M and EGFR-L858R/T790M/C797S while largely sparing wild-type EGFR142. Allosteric targeting of protein kinases offers the opportunity to mimic natural regulatory mechanisms in a highly specific manner. The therapeutic potential of these modulators of kinase activity is enormous and the kinase field has only started to explore this targeting strategy in a clinical setting. Molecular glues In the context of targeted protein degradation, molecular glues are small molecules that induce association of ubiquitin ligases with their target via monovalent interactions. However, designing a small molecule that can bind to both a ubiquitin ligase and a kinase in this way is highly challenging, and so molecular glues are most often identified by screening compound libraries. Proteolysis-targeting chimeras (PROTACs). Bivalent macromolecules composed of a flexible linker that is capped with protein-binding moieties designed to bring ubiquitin ligases and a target such as the kinase of interest into close proximity to promote its degradation. Although this strategy could enable more specific targeting of a given kinase, the large molecular size of PROTACs may pose challenges related to drug characteristics such as oral bioavailability. Covalent inhibitors. Several covalent kinase inhibitors have been approved since the pioneering approvals of the covalent EGFR inhibitor afatinib143 and the BTK inhibitor ibrutinib by the FDA in 2013 (see above). Seven additional SMKIs have been recently approved that target either EGFR mutants or BTK (Supplementary Table 1). All currently approved covalent kinase inhib itors except acalabrutinib use an acrylamide group as the electrophile for covalent bond formation (Fig. 5b). Substitution of the alpha position in an acrylamide by an electron-withdrawing group such as a cyano group allows for the design of reversible covalent inhibitors144. This has been explored in the development of sev