Translation-chapter 6 - Copy.pdf
Document Details
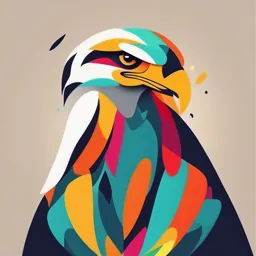
Uploaded by SucceedingLavender
2022
Tags
Full Transcript
Chapter 6 How Cells Read the Genome: From DNA to Protein Copyright © 2022 W. W. Norton & Company, Inc. The genetic code Translation: From RNA to protein. Genetic code: The nucleotide sequence of a gene is translated to the amino acid sequence of a protein by genetic code. 20 different amino acids. C...
Chapter 6 How Cells Read the Genome: From DNA to Protein Copyright © 2022 W. W. Norton & Company, Inc. The genetic code Translation: From RNA to protein. Genetic code: The nucleotide sequence of a gene is translated to the amino acid sequence of a protein by genetic code. 20 different amino acids. Codon 3-letter abbr. 1-letter abbr. Codons are written with the 5’ nucleotide to the left. Most amino acids are represented by 1+ codons. Codons for the same amino acid tend to contain the same nucleotides at the 1st and 2 nd positions and vary at the 3rd. Stop codons do not specify any amino acid but act as termination sites. There are 3 stop codons. AUG acts both as an initiation codon and specifies methionine. The reading frames during mRNA translation The mRNA sequence is read from the 5’ end to the 3’end in consecutive sets of three nucleotides. The same RNA sequence can be translated in any of three possible reading frames, depending on where the decoding begins. Only one of the three possible reading frames encodes proteins. Protein synthesis tRNA (transfer RNA) molecules match amino acids to codons in mRNA tRNAs act as adaptor molecules that recognize and bind to both the codon (on mRNAs) and amino acid. Complementary basepairing in tRNA Anticodon: the sequence of three nucleotides that base pairs with a codon in mRNA. The attached amino acid matching the codonanticodon pair is attached to the 3’ end of the tRNA. Unusual bases. The cloverleaf structure of a tRNA The L-shaped tRNA molecule The symbol of a tRNA molecule A tRNA is about 80 nucleotides in length The linear nucleotide sequence of a tRNA ,color-coded to match the illustration of the cloverleaf structure. ψ: pseudouridine D: dihydrouridine Wobble base pairing between codon and anti-codon tRNAs require accurate pairing at the first two positions and tolerate mismatch at the third position. tRNAs can base pair with more than one codon. The coupling of a specific amino acid and a tRNA Aminoacyl-tRNA synthetases couple each amino acid to its tRNA molecule Most cells have a different synthetase for each amino acid (20 synthetases for 20 amino acids). Step 1: The amino acid is linked to an AMP at its carboxyl group, forming the adenylated amino acid. This reaction is driven by ATP hydrolysis. Step 2: The AMP-linked carboxyl group on the amino acid is transferred to a hydroxyl group at the 3’end tRNA molecule, forming the aminoacyl-tRNA. STEP 1 STEP 2 The structure of the aminoacyl-tRNA The carboxyl end of the amino acid form an ester bond to ribose. The amino acid linked to tRNA is highly activated and ready to be added to a growing polypeptide chain. Ester bond The ribose on the 3’ end of the tRNA The genetic code is translated by two adaptors: aminoacyl-tRNA synthetase and tRNA The aminoacyl-tRNA synthetase couples a particular amino acid to its corresponding RNA. The tRNA molecule uses its anticodon to base pair with the codons in mRNA. Editing by aminoacyl-tRNA synthetase ensures accuracy Step 1: The incorrect amino acids, especially those larger than the correct amino acid, are excluded from the synthesis site. Step 2: If the incorrect amino acid has been linked to AMP or the tRNA (often happens when the incorrect amino acid has a structure similar to the correct amino acid), the synthetase force the adenylated amino acid into a second editing pocket. The incorrect amino acid is removed by hydrolysis. Amino acid accepting arm on tRNA Aminoacyl-tRNA synthetase The synthesis of a protein from an mRNA template The incorporation of an amino acid into a protein Amino acids are added to the C-terminus of a growing polypeptide chain. A peptide bond between the carboxyl group on the growing polypeptide and the free amine group of the new amino acid is formed. This C-terminus is “activated” because it attaches to the peptidyl tRNA. Protein synthesis is performed by ribosomes Ribosomes attached to endoplasmic reticulum Free ribosomes Ribosomes are composed of protein and ribosome RNA (rRNA) Eukaryotic and bacterial ribosomes have similar structures and functions. Ribsomes are composed of small and large subunits. The small subunit: provides a framework on which the anticodon of tRNA matches the codon on mRNA. The large subunit: catalyze the formation of the peptide bond The RNA binding sites in a ribosome Each ribosome has one binding site for mRNA and three binding sites, E (exit), P (peptidyl-tRNA), and A (aminoacyl-tRNA) for tRNA. No more than two sites are thought to contain tRNA during protein synthesis. The structure of a bacterial ribosome E site P site A site An mRNA molecule is through a ribosome mRNA Each new amino acid is added to an elongating peptide chain by four steps. tRNA binding New Peptide bond formation Large subunit translocation small subunit translocation Elongation factors, EF-Tu and EF-G, drive translation forward and improve its accuracy. Mechanism 1: EF-Tu can bind to both GTP and aminoacyl-tRNAs, a correct codon-anticodon match will bind more tightly than an incorrect interaction. Mechanism 2: The 16s rRNA in the small subunit of the ribosome assesses the correctness of the codonanticodon match by folding around it. When a correct match is found, the rRNA closes tightly around the codon-anticodon pair, causing a conformational change in the ribosome that triggers GTP hydrolysis by EF-Tu. Once GTP is hydrolyzed, EF-Tu releases the aminoacyl-tRNA and allows it to be used in protein synthesis. Incorrect codon-anticodon matches do not trigger the conformational change and the incorrect tRNA falls off. EF-G binds to ribosome and the hydrolysis of GTP leads to rearrangement of the ribosome structure, moving the mRNA exactly three nucleotides through the ribosome. The initiation of protein synthesis in eukaryotes Efficient translation initiation requires the poly-A tail of the mRNA bound by poly-Abinding proteins. In this way, the translation apparatus ensures that both ends of mRNA are intact before initiating protein synthesis. The initiator tRNA-methionine (met-tRNA) complex is first loaded onto the small subunit along with eukaryotic initiation factor 2 (eIF2). Only met-tRNA can bind to the small unit without the complete ribosome and the P site. The small subunit binds to the 5’ end of an mRNA, which is recognized by its 5’ cap bound to eIF4G and eIF4E. The small ribosomal subunit moves forward along mRNA, searching for the first AUG (start codon, also codes for methionine). Translation initiates when the first AUG is encountered and the eIFs dissociate. The large ribosome subunit binds to form a complete ribosome. The vacant A site is bound by an aminoacyl-tRNA charged with a new amino acid. The first peptide bond forms between the Met and the new amino acid. * See slide 24 for step 1 and step 2. Prokaryotic ribosomes initiate translation at ribosome binding sites There can be multiple ribosome binding sites located anywhere along a bacterial mRNA molecule. Bacteria can synthesize more than one type of protein from a single mRNA molecule. Stop codons mark the end of the translation Three stop codons (UAA, UAG or UGA) specify the end of a reading frame. Stop codons do not recognize by any tRNA, but are bound by release factors. Release factors bind to any ribosome with a stop codon at the A site, forcing the peptidyl transferase in the ribosome to catalyze the addition of a water molecule. This reaction frees the carboxyl end of a growing polypeptide to the ribosome. The completed protein chain is released to the cytoplasm. The ribosome releases the mRNA and dissociates into large and small subunits. Proteins are made on polyribosomes Polyribosomes (or polysomes): large cytoplasmic assemblies made up of several ribosomes along a single mRNA molecule. Allow the cell to translate the same mRNA molecule simultaneously. Nonsense-mediated mRNA decay acts to prevent translation of damaged mRNAs Nonsense-mediated mRNA decay prevents defective mRNAs from escaping the nuclear envelope. Abnormal splicing of a pre-mRNA introduces a premature stop codon into the reading frame. An mRNA molecule, bearing EJCs is quickly “scanned” by a ribosome. As the mRNA passes through the ribosome, the EJCs are stripped off and successful mRNAs are released for translation. If an in-frame stop codon is encountered before the final EJC is reached, the mRNA undergoes nonsense-mediated decay, which triggers by the Upf proteins. Protein folding, modification, and assembly The nascent polypeptide must be processed to become functional A growing polypeptide is folding into its secondary and tertiary structure as it emerges from a ribosome Molecular Chaperons help guide the folding of most proteins during protein synthesis Many molecular chaperons are called heat-shock proteins (hsp). Major families of molecular chaperons include hsp60 and hsp70. Members in hsp70 help protein folding in repeated cycles of three steps: -Trigger: short stretches of hydrophobic amino acids that are abnormally exposed in misfolded proteins trigger the hydrolysis of ATP to ADP in hsp70 proteins. -Trap: The hsp70 proteins close down their substrate, trapping it in its extended form. -Release: The rebinding of another ATP opens the hsp70 clamp, releasing the substrate in its extended form. The three-dimensional structure of hsp70 Hsp70 members bind to the ribosome and act on a newly synthesized protein The repeated cycles of hsp70 clamping and release help fold the target protein until the protein is properly folded. Hsp60 proteins form a large barrel and help protein folding after the protein is fully synthesized. The hsp60-like proteins refold the misfolded proteins in three steps: - Capture: a misfolded protein is captured by hydrophobic interactions with the surface of the opening barrel. - Refolding: binding of ATP and a GroES cap releases the substrate into an enclosed space, where the protein has a chance to refold. - Release: ATP hydrolysis weakens the binding of the cap, and the binding of another ATP ejects the cap and releases the protein. Capture Refolding Release The structure of a hsp60-like protein in bacteria GroES GroEL The incompletely folded proteins are digested by the proteasome Both molecular chaperons and proteasomes recognize large patches of exposed hydrophobic residues. The structure of a proteasome The misfolded proteins are degraded in the central cylinder. The cylinder is hollow and formed by multiple protein subunits that assemble into a stack of 4 heptameric rings. Proteases are on the subunits, with their active sites facing into the cylinder. Processive protein digestion by the proteasome Recognition: the cap of the proteasome recognizes and binds to proteins marked by ubiquitin for destruction. Translocation: the proteasome cap moves the protein to the cylinder core by a threading reaction, driven by ATP-hydrolysis. Ubiquitin removal: the ubiquitin is cleaved from the substrate protein and recycled Unfolding: the unfoldase ring in the proteasome cap unfolds the protein as it moves through the cap. Destruction: the protein is exposed to the active sites lining in the proteasome core for destruction. The structure of the proteasome cap The ubiquitin receptor holds a ubiquitylated protein in place during the protein substrate translocation. The ubiquitin hydrolase cleaves ubiquitin from the protein. The unfoldase ring unfolds the protein substrate during pulling. The structure of the proteasome cap Ubiquitin receptor and ubiquitin hydrolase (Unfoldase ring) A hexameric protein unfoldase unfolds and pulls the substrate protein into the proteasome core The ATP-bound form of the unfoldases binds a folded substrate protein by its ubiquitin tag. The conformational change of the hexameric ring driven by ATP hydrolysis pulls the substrate into the central core and strains the ring structure. Many cycles of ATP hydrolysis cause the protein substrate to unfold and be pulled into the hexameric ring. The substrate protein enters the proteasome core. Inducing the destruction of a specific protein The degradation signal is in the protein: the misfolded proteins have patches of hydrophobic residues on the surface and can be recognized. There are two ways to induce the destruction of a specific protein: 1) by activating a ubiquitin ligase, and 2) by creating ubiquitylation sites in a protein. Inducing the protein destruction by activating of a ubiquitin ligase The active ubiquitin ligase adds ubiquitin tags to the protein to be degraded. The activity of a ubiquitin ligase is turned on by 1) E3 phosphorylation, 2) an allosteric transition in E3 caused by its binding to a ligand, or 3) a protein substrate. Inducing protein destruction by activating a degradation signal The activated degradation signal will serve as a ubiquitin ligase recognition site. The phosphorylation of a specific site on a protein unmasks a normally hidden degradation signal. The regulated dissociation of a protein subunit unmasks the degradation signal. The cleavage of a single peptide bond creates a destabilizing N-terminal site that is recognized by a specific E3 protein. From DNA to protein Take home messages The tRNA molecules act as adaptors to bring the correct amino acid that matches the codon on the mRNA. The initiation, elongation, and termination of protein synthesis require ribosomes, specific translation initiation and elongation factors, and start and stop codons on mRNA. Several types of molecular chaperons help newly synthesized proteins fold correctly. Misfolded or incompletely folded proteins are recognized by the hydrophobic patches on their surface and sent to proteasomes for destruction. Activation of ubiquitin ligase and degradation signal regulates protein destruction by proteasomes. Quiz What is the function of HSP 60? 1. It allows proteins a chance to fold correctly 2. It binds to and degrades incorrectly folded proteins 3. It marks a protein for degradation 4. It is a subunit of the HSP 70 complex 5. It binds to the ribosome at a stop codon to terminate translation