Transcription in General Genetics (AGN 101) PDF
Document Details
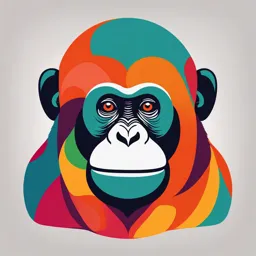
Uploaded by EnergySavingPeach4258
Cairo University
Dr. Shereen Abu El-Maaty
Tags
Summary
This document provides lecture notes on transcription, specifically targeting general genetics (AGN 101) course. It details the process of converting DNA to RNA and explores various types of RNA and their functions. The presentation is accompanied by diagrams and tables that further clarify the concepts.
Full Transcript
Course: General Genetics AGN 101 By Dr. Shereen Abu El-Maaty TANSCRIPTION Synthesis of a single-stranded RNA molecule using the DNA template (1 strand of DNA is transcribed). The process of converting DNA to messenger RNA Takes place in the nucleus RNA STRUC...
Course: General Genetics AGN 101 By Dr. Shereen Abu El-Maaty TANSCRIPTION Synthesis of a single-stranded RNA molecule using the DNA template (1 strand of DNA is transcribed). The process of converting DNA to messenger RNA Takes place in the nucleus RNA STRUCTURE RNA, like DNA, is a polymer consisting of nucleotides joined together by phosphodiester bonds. However, there are several important differences in the structures of DNA and RNA. mRNA Strands mRNA is always synthesized 5’ to 3’ on a 3’ to 5’ template Nonsense strand 5’ 3’ (non template) 3’ 5’ Sense strand (template) 5’ 3’ CLASSES OF RNA 1-Ribosomal RNA ( r RNA) forms complexes called ribosomes with protein, the structure on which mRNA is translated. 2-Messenger RNA ( mRNA) carries the coding instructions for polypeptide chains from DNA to the ribosome. 3- Transfer RNA ( tRNA) transports amino acids to ribosomes during translation. Each tRNA attaches to one particular type of amino acid and helps to incorporate that amino acid into a polypeptide chain. 4- Small nuclear RNAs (snRNAs) are found in the nuclei of eukaryotic cells. forms complexes with proteins used in eukaryotic RNA processing (e.g., exon splicing and intron removal). Small RNA molecules also are found in the cytoplasm of eukaryotic cells; these molecules are called small cytoplasmic RNAs (scRNAs). 5-snoRNA (small nucleolar RNA) : Facilitates chemical modification of other RNAs, particularly rRNA. 6-miRNA (microRNA) : regulates gene expression by binding to mRNA. 7-siRNA (small interfering RNA) :short ~22 nt RNA sequences that bind to 3’ UTR target mRNAs and result in silencing. 8- IncRNA (long non-coding RNA) : regulates gene expression in various ways, often associated with chromatin structure or transcription. All cellular RNAs are synthesized from a DNA template through the process of transcription Transcription: How is an RNA strand synthesized? 1. Regulated by gene regulatory elements within each gene. 2. DNA unwinds next to a gene. 3. RNA is transcribed 5’ to 3’ from the template (3’ to 5’). Similar to DNA synthesis, except: NTPs instead of dNTPs (no deoxy-) No primer No proofreading Adds Uracil (U) instead of thymine (T) RNA polymerase RNA POLYMERASE The enzyme responsible for the RNA synthesis is DNA- dependent RNA polymerase. The prokaryotic RNA polymerase is a multiple- subunit protein of ~480kD. Eukaryotic systems have three kinds of RNA polymerases, each of which is a multiple-subunit protein and responsible for transcription of different RNAs. Catalyze phosphodiesterase bond formation Holoenzyme The holoenzyme of RNA-pol in E.coli consists of 5 different subunits: 2 . RNA-pol of E. Coli subunit MW function Determine the DNA to be 36512 transcribed 150618 Catalyze polymerization 155613 Bind & open DNA template Recognize the promoter 70263 for synthesis initiation Recognition of Origins Each transcriptable region is called operon. One operon includes several structural genes and upstream regulatory sequences (or regulatory regions). The promoter is the DNA sequence that RNA-pol can bind. It is the key point for the transcription control. Each gene has three regions: 1. 5’ Promoter, attracts RNA polymerase -10 bp 5’-TATAAT-3’ -35 bp 5’-TTGACA-3’ 2. Transcribed sequence (transcript) or RNA coding sequence 3. 3’ Terminator, signals the stop point Promoter regulatory structural gene sequences 5' 3' promotor RNA-pol 3' 5' Prokaryotic promoter 5' 3' -50 -40 -30 -20 -10 1 10 3' 5' -35 region -10 start TTGACA region AACTGT TATAAT ATATTA (Pribnow box) Consensus sequence The -35 region of TTGACA sequence is the recognition site and the binding site of RNA-pol. The -10 region of TATAAT is the region at which a stable complex of DNA and RNA-pol is formed. STEPS OF TRANSCRIPTION Three Steps to Transcription: 1. Initiation 2. Elongation 3. Termination Occur in both prokaryotes and eukaryotes. Elongation is conserved in prokaryotes and eukaryotes. Initiation and termination proceed differently. 1. Initiation 1. RNA polymerase combines with sigma factor (a polypeptide) to create RNA polymerase holoenzyme RNA-pol recognizes the (promoters) TTGACA region, and slides to the TATAAT region, then opens the DNA duplex. The unwound region is about 171 bp. Sigma factor required for efficient binding and transcription. 2. RNA polymerase holoenzyme binds promoters and untwists DNA Binds loosely to the -35 promoter (DNA is d.s.) Binds tightly to the -10 promoter and untwists 3. Different types and levels of sigma factors influence the level and dynamics of gene expression (how much and efficiency). The first nucleotide on RNA transcript is always purine triphosphate. GTP is more often than ATP. The three molecules form a transcription initiation complex. RNA-pol (2) - DNA - pppGpN- OH 3 No primer is needed for RNA synthesis. The subunit falls off from the RNA-pol once the first 3,5 phosphodiester bond is formed. The core enzyme moves along the DNA template to enter the elongation phase. Fig. 5.4 2. Elongation After 8-9 bp of RNA synthesis occurs, subunit is released and causes the conformational change of the core enzyme. The core enzyme slides on the DNA template toward the 3 end. DNA untwists rapidly, and re-anneals behind the enzyme. Free NTPs are added sequentially to the 3 -OH of the nascent RNA strand. (NMP)n + NTP (NMP)n+1 + PPi elongated RNA strand substrate RNA strand RNA-pol, DNA segment of ~40nt and the nascent RNA form a complex called the transcription bubble. The 3 segment of the nascent RNA hybridizes with the DNA template, and its 5 end extends out the transcription bubble as the synthesis is processing. RNA polymerase completes the transcription at 30-50 bp/second. Transcription bubble 3. Termination The RNA-pol stops moving on the DNA template. The RNA transcript falls off from the transcription complex. Two types of terminator sequences occur in prokaryotes: 1. Type I (-independent) Palindromic, inverse repeat forms a hairpin loop and is believed to physically destabilize the DNA-RNA hybrid. Rho-independent terminators have two common features. First, they contain inverted repeats (sequences of nucleotides on one strand that are inverted and complementary).When inverted repeats have been transcribed into RNA, a hairpin secondary structure forms. Second, in rho-independent terminators, a string of approximately six adenine nucleotides follows the second inverted repeat in the template DNA. Their transcription produces a string of uracil nucleotides after the hairpin in the transcribed RNA. The presence of a hairpin in an RNA transcript causes RNA polymerase to slow down or pause, which creates an opportunity for termination. The adenine–uracil base pairings downstream of the hairpin are relatively unstable compared with other base pairings, and the formation of the hairpin may itself destablize the DNA–RNA pairing, causing the RNA molecule to separate from its DNA template. When the RNA transcript has separated from the template,RNA synthesis can no longer continue. 2- Type II (-dependent) Rho Involves rho () factor proteins, believed to break the hydrogen bonds between the template DNA and RNA. The factor, a hexamer large protein, is a ATPase and a helicase Multiple mRNA Transcription Making a single mRNA strand is not very efficient In fact, multiple strands are produced by multiple RNA polymerases New RNA Chain RNA Polymerase 3' 5' DNA 5' 3' Multiple mRNA Transcription THE BASIC RULES OF TRANSCRIPTION 1. Transcription is a selective process; only certain parts of the DNA are transcribed. 2. RNA is transcribed from single-stranded DNA. Normally, only one of the two DNA strands—the template strand—is copied into RNA. 3. Ribonucleoside triphosphates are used as the substrates in RNA synthesis. Two phosphates are cleaved from a ribonucleoside triphosphate, and the resulting nucleotide is joined to the 3-OH group of the growing RNA strand. 4. RNA molecules are antiparallel and complementary to the DNA template strand. Transcription is always in the 5 :3 direction, meaning that the RNA molecule grows at the 3 end. 5. Transcription depends on RNA polymerase—a complex, multimeric enzyme. RNA polymerase consists of a core enzyme, which is capable of synthesizing RNA, and other subunits that may join transiently to perform additional functions. 6. The core enzyme of RNA polymerase requires a sigma factor in order to bind to a promoter and initiate transcription. 7. Promoters contain short sequences crucial in the binding of RNA polymerase to DNA; these consensus sequences are interspersed with nucleotides that play no known role in transcription. 8. RNA polymerase binds to DNA at a promoter, begins transcribing at the start site of the gene, and ends transcription after a terminator has been transcribed. NEXT 1-mRNA Transcription in eukaryotes 2-Post-transcriptional Processing of mRNA 3-Genes that do not code proteins TRANSCRIPTION IN EUKARYOTES Transcription is more complicated in eukaryotes. Eukaryotes possess three RNA polymerases: 1. RNA polymerase I, transcribes three major rRNAs 12S, 18S, 5.8S 2. RNA polymerase II, transcribes mRNAs and some snRNAs 3. RNA polymerase III, transcribes tRNAs, 5S rRNA, and snRNAs Transcription initiation needs promoter and upstream regulatory regions. The cis-acting elements are the specific sequences on the DNA template that regulate the transcription of one or more genes We can divide eukaryotes promoter into two regions: 1. The core promoters elements. The best characterized are A short sequence called Inr (Initiator) TATA Box = TATAAAA, located at about position -30 *AT-rich DNA is easier to denature than GC-rich DNA 2. Promoter proximal elements (located upstream, ~-50 to -200 bp) “Cat Box” = CAAT and “GC Box” GGGCGG Different combinations occur near different genes. Transcription regulatory proteins (activators) and enhancers also are required. Cis-acting element cis-acting element structural gene GCGC CAAT TATA exon intron exon start TATA box (Hogness box) enhancer CAAT box GC box TATA box Transcription regulatory proteins = Activators High-level transcription is induced by binding of activator factors to DNA sequences called enhancers. Enhancers are usually located upstream of the gene they control, they modulate transcription from a distance. Can be several kb from the gene Silencer elements and repressor factors also exist Transcription factors The eukaryotic RNA-pol requires co-factors to bind to the DNA template together in the transcription process. RNA-pol does not bind the promoter directly. RNA-pol II associates with six transcription factors, TFII A - TFII H. The trans-acting factors are the proteins that recognize and bind directly or indirectly cis-acting elements and regulate its activity. TF for eukaryotic transcription Order of binding is: IID + IIA + IIB + RNA poly. II + IIF +IIE +IIH Fig. 5.7. Pre-initiation complex (PIC) TBP of TFII D binds TATA TFII A and TFII B bind TFII D TFII F-RNA-pol complex binds TFII B TFII F and TFII E open the dsDNA (helicase and ATPase) TFII H: completion of PIC Pre-initiation complex (PIC) RNA pol II TF II F TF II E TF II TBP TAF TF II A TATA B TF II H DNA b. Elongation The elongation is similar to that of prokaryotes. The transcription and translation do not take place simultaneously since they are separated by nuclear membrane. c. Termination The termination sequence is AATAAA followed by GT repeats. The termination is closely related to the post-transcriptional modification. Production of the mRNA molecule Three main parts: 1. 5’ untranslated region (5’ UTR) or leader sequence 2. Coding sequence, specifies amino acids to be translated 3. 3’ untranslated region ( 3’ UTR) or trailer sequence may contain information that signals the stability of the particular mRNA mRNA differences between prokaryotes and eukaryotes: Prokaryotes 1. mRNA transcript is mature, and used directly for translation without modification. 2. Since prokaryotes lack a nucleus, mRNA also is translated on ribosomes before it is transcribed completely (i.e., transcription and translation are coupled). 3. Prokaryote mRNAs are polycistronic, they contain amino acid coding information for more than one gene. Eukaryotes 1. mRNA transcript is not mature (pre-mRNA); must be processed. 2. Transcription and translation are not coupled (mRNA must first be exported to the cytoplasm before translation occurs). 3. Eukaryote mRNAs are monocistronic, they contain amino acid sequences for just one gene. Fig. 5.9. Prokaryotes and Eukaryotes Post-Transcriptional Modification The nascent RNA, also known as primary transcript or heteronuclear RNA (hnRNA)., needs to be modified to become functional tRNAs, rRNAs, and mRNAs. hnRNA are larger than matured mRNA by many folds. The modification is critical to eukaryotic systems. Post-Transcriptional Modification Modification includes Capping at the 5- end Tailing at the 3- end mRNA splicing RNA edition 1-Capping at the 5- end After 20-30 nucleotides have been synthesized, the 5’-end of the mRNA is capped 5’ to 5’ with a guanine nucleotide. Results in the addition of two methyl (CH3) groups. Essential for the ribosome to bind to the 5’ end of the mRNA. (guanine + CH3 group) Capping at the 5- end OH OH O N NH O O O O 5' H2N N N H2C O P O P O P O CH2 N NH2 N 5' O O O O HN N O CH 3 O OH Pi O P O AAAAA-OH 3' O m7GpppGp---- The 5- cap structure is found on hnRNA too. The capping process occurs in nuclei. The cap structure of mRNA will be recognized by the cap-binding protein required for translation. The capping occurs prior to the splicing. 2. POLY-A TAILING AT 3 - END There is no poly(dT) sequence on the DNA template. The tailing process dose not depend on the template. The tailing process occurs prior to the splicing. The tailing process takes place in the nuclei. o 50-250 adenine nucleotides are added to 3’ end of mRNA. o Complex enzymatic reaction. o Stabilizes the mRNA, and plays an important role in transcription termination. 3. mRNA SPLICING Many eukaryotic genes contain exons and introns, both of which are transcribed into RNA, but introns are later removed by RNA processing. The number and size of introns vary from gene to gene; they are common in many eukaryotic genes but uncommon in bacterial genes. The matured mRNAs are much shorter than the DNA templates. Exon and intron Exons are the coding sequences that appear on split genes and primary transcripts, and will be expressed to matured mRNA. Introns are the non-coding sequences that are transcripted into primary mRNAs, and will be cleaved out in the later splicing process. The process of RNA splicing takes place within a structure called the spliceosome, which is composed of several small nuclear RNAs and proteins. RNA splicing takes place in a two-step process: that entails RNA–RNA interactions among snRNAs of the spliceosome and the pre-mRNA. snRNP’s recognize sequences and cut out the introns and join the exons. mRNA splicing of exons and removal of introns: 1. Introns typically begin with a 5’-GU and end with AG-3’. 2. Cleavage occurs first at the 5’ end of intron 1 (between 2 exons). 3. The now free G joins with an A at a specific branch point sequence in the middle of the intron, using a 2’ to 5’ phosphodiester bond. Intron forms a lariat-shaped structure. 4. Lariat is excised, and the exons are joined to form a spliced mRNA. 5. Splicing is mediated by splicosomes, complexes of small nuclear RNAs (snRNAs) and proteins, that cleave the intron at the 3’ end and join the exons. 6. Introns are degraded by the cell. 4- mRNA editing: RNA editing is a molecular process through which some cells can make discrete changes to specific nucleotide sequences within a RNA molecule after it has been generated by RNA polymerase. 1. Adds or deletes nucleotides from a pre-RNA, or chemically alters the bases, so the mRNA bases do not match the DNA sequence. 2. Can results in the substitution, addition, or deletion of amino acids (relative to the DNA template). 3. Generally cell or tissue specific. Genes that do not code proteins also are transcribed: 1. rRNA, ribosomal RNA Catalyze protein synthesis by facilitating the binding of tRNA (and their amino acids) to mRNA. 1. tRNA, transfer RNA Transport amino acids to mRNA for translation. 2. snRNA, small nuclear RNA Combine with proteins to form complexes used in RNA processing (splicosomes used for intron removal). 1. Synthesis of ribosomal RNA and ribosomes: 1. Cells contain thousands of ribosomes. 2. Consist of two subunits (large and small) in prokaryotes and eukaryotes, in combination with ribosomal proteins. 3. E. coli 70S model: 50S subunit = 23S (2,904 nt) + 5S (120 nt) + 34 proteins 30S subunit = 16S (1,542 nt) + 20 proteins 4. Mammalian 80S model: 60S subunit = 28S (4,700 nt) +5.8S (156 nt) + 5S (120 nt) + 50 proteins 40S subunit = 18S (1,900 nt) + 35 proteins 5. DNA regions that code for rRNA are called ribosomal DNA (rDNA). 6. Eukaryotes have many copies of rRNA genes tandemly repeated. THE RIBOSOMAL RNAS FORM TWO SUBUNITS, THE LARGE SUBUNIT (LSU) AND SMALL SUBUNIT (SSU). Type Size Large subunits Small subunits Prokaryotes 70S 50S (5S, 23S) 30S (16S) Eukaryotes 60S (5S, 5.8S, 80S 40S (18S) 28S) Both prokaryotic and eukaryotic ribosomes can be broken down into two subunits (the S in 16S represents Svedberg units). The S units of the subunits cannot simply be added because they represent measures of sedimentation rate rather than of mass. The sedimentation rate of each subunit is affected by its shape, as well as by its mass. 2. Synthesis of tRNA: 1. tRNA genes also occur in repeated copies throughout the genome, and may contain introns. 2. Each tRNA (75-90 nt in length) has a different sequence that binds a different amino acid. 3. Many tRNAs undergo extensive post-transcription modification, especially those in the mitochondria and chloroplast. 4. tRNAs form clover-leaf structures, with complementary base- pairing between regions to form four stems and loops. 5. Loop #2 contains the anti-codon, which recognizes mRNA codons during translation. 6. Same general mechanism using RNA polymerase III, promoters, unique TFs, plus posttranscriptional modification from pre-tRNA. Figure 6.9 3. Synthesis of snRNA (small nuclear RNA): Form complexes with proteins used in eukaryotic RNA processing, such as splicing of mRNA after introns are removed. Transcribed using RNA polymerase II or III. Associated with small nuclear ribonucleoproteins (snRNPs). Also function in regulation of transcription factors and maintenance of telomeres.