Transcription and Translation PDF
Document Details
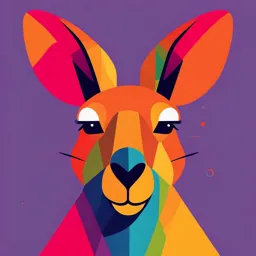
Uploaded by BrighterDalmatianJasper
Anglia Ruskin University
Tags
Related
- Molecular Biology of Gene Regulation PDF
- Gene Expression H. Biology 2023-2024 Albayan University PDF
- Transcription and Translation (Gene Expression) PDF
- Gene Expression - BE101 Lecture 8 PDF
- Biology Chapter 18 Lecture Regulation of Gene Expression PDF
- Biology Chapter 18 Lecture Notes (gene expression) PDF
Summary
This document provides an overview of transcription and translation, including details of the processes involved, different types of genes, and the role of RNA polymerase. It also discusses the genetic code and the synthesis of proteins.
Full Transcript
What is a gene ? Definition depends on who is asking (context dependent) Geneticists: discrete unit of inheritance that is passed from one generation to another – determines traits (Gregor Mendel) Biochemical: sequence of bases that define a functional protein BUT NOTE That protein-coding...
What is a gene ? Definition depends on who is asking (context dependent) Geneticists: discrete unit of inheritance that is passed from one generation to another – determines traits (Gregor Mendel) Biochemical: sequence of bases that define a functional protein BUT NOTE That protein-coding sequences are flanked by regulatory DNA sequences that are not themselves encoding amino acids Also get genes that gives rise to non-protein encoding RNA molecules All cellular RNAs are transcribed from DNA templates Something to think about…. Number of genes in a fruit fly ≈ 16000 genes Number of genes in human genome ≈ 30000 genes (max !) (gene predication has become more accurate so 20000-25000 genes) But we are not just twice as complex as flies ! What drives biological complexity if it is not the number of genes ?? Some essential terminology… The flow of information in gene expression (Central Dogma of Molecular Biology) replication transcription translation DNA mRNA protein genome transcriptome proteome DNA to protein - overview Information in DNA is encoded in sequence of 4 bases This sequence directs assemblage of 20 amino acids in a correct sequence 4 bases code 20 amino acids using a three base code (triplet codon) (three letter words using a four letter alphabet !) DNA is enclosed in the nucleus, protein synthesizing machinery in cytoplasm – the two never meet ! Copies of coded info (sequence of bases) are sent to the cytoplasm by messenger RNA (mRNA) Prokaryotic vs Eukaryotic genes Prokaryotes genes are arranged close together with short ‘spacer segments’ and the coding region (specifying aa sequence) is continuous Eukaryotes the coding region is interrupted by segments of DNA that do not code for aa sequences Coding regions are called exons and noncoding regions are called introns Can have 1-500 introns in a gene, which itself might be 50-20000 nucleotides long Exons tend to be smaller (~150 base pairs) 5’ and 3’ ends of a gene A gene is a sequence of DNA that is transcribed into RNA (strand used is the template strand, strand not used is the coding strand !) Regions of DNA flanking the transcribed sequence have roles in the transcription process – but not transcribed into RNA At the 5’ end of gene is the promoter region required for transcription At the 3’ end is the terminator region, required for termination The first template nucleotide is indicated as +1 and 5’ to this is -1 Structure of a prokaryotic gene and its mRNA First template nucleotide 5’ DNA 3’ transcription mRNA Template strand of duplex DNA is the antisense or noncoding strand Coding strand has same sequence as the transcribed RNA Sense and antisense DNA strands RNA polymerase mRNA synthesized by DNA-dependent RNA polymerase Synthesis of mRNA requires duplex DNA strands to be separated to provide single – stranded template Temporary separation of strands (separation ‘bubble’) RNA synthesis is in the 5’ → 3’ direction (as in DNA replication) RNA polymerase can initiate new chains – does not need primer Synthesis of mRNA RNA polymerase Phases of gene transcription in E. coli 3 phases – initiation, elongation, and termination Initiation: RNA polymerase binds to promoter region (sequence of bases) of gene E. coli promoter has two important (conserved) regions: Pribnow box centred at nucleotide -10 and another -35 RNA polymerase E. coli RNA polymerase core enzyme is a protein with subunit composition α2ββ’ω but alone cannot recognise the correct initiation site Binding with a sigma (σ) subunit (factor) allows holoenzyme (α2ββ’ωσ) to bind consensus sequences and initiation starts Different sigma factors recognise different promoters (selective gene expression) Once RNA synthesis initiated σ (sigma) unit dissociates from core enzyme, α2ββ’ω, carries out polymerisation – core enzyme does not specifically bind promoters RNA polymerase RNA chain grows in the 5’ → 3’ direction (just like DNA polymerase) RNA polymerase is processive - transcription proceeds without dissociation of the enzyme from the template Transcription is rapid 20-50 nucleotides/s (slower than DNA replication ~1000 nt/s Error rate of transcription is high (1 in 104 transcribed) but this tolerable because genes are repeatedly transcribed (many copies), genetic code is redundant (more on this later) Termination of transcription 2 mechanisms Formation of a stem-loop structure by internal base pairing (G-C pairs so quite stable !). This disrupts the elongation process, by preventing binding An additional protein, Rho factor, attaches to new mRNA and unwinds (helicase activity) the RNA-DNA duplex and releases mRNA Eukaryotic RNA polymerase Basic reaction same as in prokaryotes - multiple forms and control seq Eukaryotes have three different RNA polymerases (I, II, III) Differ in the RNA they synthesize (pol II transcribes mRNA ie protein- encoding genes) In eukaryotes, gene expression highly regulated process – is not translated as it is produced Primary transcript undergoes several modifications Eukaryotic RNA polymerase has to negotiate histones – not totally stripped away from DNA template – mechanism not completely understood ! Major modifications of eukaryotic mRNA Addition of a ‘cap’ structure at the 5’ end (capping) Addition of polyA tail to 3’ end (can be up to 250) Slicing to remove introns (noncoding regions) Alternative splicing Splicing can occur in different patterns so that a particular combination of exons forms one mRNA 5’ cap of eukaryotic mRNAs Consists of 7-methylguanosine (m7G) residue joined at the transcripts initial (5’) nucleotide – added when transcript is about 30nt long Identifies eukaryotic translation start site Involves several enzymes – removal of leading phosphate group from mRNA 5’ terminal triphosphate group (RNA triphosphatase) Guanylylation of mRNA by capping enzyme (guanylyltransferase) – requires GTP – note the triphosphate bridge Methylation of guanine by guanine-7-methyltransferase Further methylations at first and second nucleotides Capping protects mRNAs from exonucleolytic degradation/initiation of translation Poly(A) tails Mature eukaryotic mRNA have 3’-ends terminating in poly(A) tails of approx. 250nt Enzymatically added, generated from ATP Not required for mRNA translation Shorten as transcript ages in cells – suggesting a protective role Splicing removes introns Key difference between eukaryotic and prokaryotic genes is that eukaryotic genes are interspersed with unexpressed regions Primary transcript (pre-mRNA or heterogenous nuclear RNA –hnRNA) much larger than sizes of proteins Caps and tails of mRNA appear in cytosolic mRNA Intervening sequences (introns) are excised and the flanking expressed sequences are sliced (joined) together Number of introns in a gene varies from zero to several hundred Splicing starts during the elongation phase of transcription Steps in the production of mature mRNA Exons are sliced in a two-stage reaction Exon-intron junctions have a high degree of homology with invariant GU at the intron’s 5’ boundary and AG at 3’ boundary There are other signals that define the splice site Splicing occurs via two transesterification reactions 2’-OH group of an adenine nucleotide in the intron chain attacks the 5’ phosphate of the G nucleotide, forming a lariat structure Mechanism of Splicing 2’-OH group of an adenine nucleotide in the intron chain attacks the 5’ phosphate of the G nucleotide, breaking chain and forming a lariat structure The 3’-OH (of exon 1) than attacks the 5’end of exon 2 – joining the exons (no energy required) Mechanism of Splicing and significance In eukaryotes, splicing reaction in the nucleus is catalysed by very complex protein-RNA molecular structure called a spliceosome The RNA molecules are called small nuclear RNAs (snRNAs) and when complexed with proteins are termed small nuclear ribonucleoproteins (snRNPs)…pronounced “snurps” Spliceosome – complicated machine (>300 polypeptides), highly dynamic with components associating and dissociating during specific stages of splicing process Significance of splicing: rapid protein evolution and single genes being able to encode several different proteins with different functions (alternative splicing) Protein synthesis- translation Translation – language of nucleotide bases is translated to the language of protein amino acids Involves use of transfer RNA (tRNA) – bring amino acids to and recognises codon sequences in mRNA by base pairing Occurs on ribosomes (RNA and protein structures) present in cytosol, move along mRNA and assemble amino acids into a sequence The Genetic Code – key points Triplet codons correspond to particular amino acids With a few exceptions the code is almost universal ! Three codons are serve as STOP signals (UAA, UAG and UGA) encode no amino acids (nonsense codons) 61 codons all code for amino acids – one amino acid is likely to have more than one codon (genetic code is degenerate) Only two amino acids, methionine and tryptophan, have single codons (AUG for methionine) AUG and GUG (less frequently) are start codons The Genetic Code The Genetic Code Use the code by reading from the centre to the outside Example: AUG codes for Methionine Name the Amino Acids GGG? Glycine UCA? Serine CAU? Histidine GCA? Alanine AAA? Lysine The genetic code… When several codons exist for one amino acid, the codons tend to be closely related and vary mainly in the third base eg isoleucine AUU, AUC and AUA Also, codons for similar amino acids tend to be similar eg isoleucine and leucine are similar aliphatic amino acids, codons are CUU (leucine) and AUU (isoleucine) What are the genetic consequences of these two points ? Genetic buffering ! Peptide synthesis- basic chemistry Joining two AAs together requires energy (peptide bond) Need to activate each amino acid, activation links each AA to a transfer RNA (tRNA) = adapter molecule Activation involves attaching AA to correct tRNA by an ester bond between the -OH group on the ribose at 3’ terminus of tRNA and -COOH of amino acid. Requires ATP and is cytosolic process Family of enzyme called aminoacyl-tRNA synthetases Each activates specific AA and recognises specific tRNA molecules Transfer RNA (tRNA) All tRNAs have similar structures – all interact with ribosomes in similar ways Tertiary structure of tRNAs is maintained by extensive stacking interactions and base pairing within and between its helical stems Transfer RNA (tRNA) Small RNAs less than 100 nucleotides Clover leaf structure as internal base pairing forms the step loops Three unpaired bases form the anticodon and the 3’-CAA terminal nucleotide flexible arm to which AA is attached Anticodon is complementary to a codon eg if codon is 5’UUC3’ anticodon on tRNA is 5’GAA3’ Ribosomes Small organelles sites of protein synthesis (large content of rRNA) Ribosome binds mRNA and reads codons with high fidelity Ribosomes also have specific binding sites for tRNA molecules Ribosomes mediate interactions of other nonribosomal protein factors that allow polypeptide initiation, elongation, and termination Catalyse peptide bond formation Undergoes movement so that it translates codons in a sequential manner (correct reading frame) Ribosomal RNA (rRNA) Ribosomes consist of two subunits Eukaryotic ribosomes larger than prokaryotic ribosomes Highly folded compact structures due to internal base pairing Fig shows RNA of E. coli ribosome 50S Initiation of protein synthesis (E. coli) 30S mRNA transcript start codon AUG attaches to the small ribosomal subunit (two different tRNAs both specific for methionine !) The initiating aminoacyl-tRNA has structural features that are recognized by an initiating factor or protein (IF2) – helps to deliver tRNA to the initiation complex Shine-Dalgarno sequence on the mRNA – complementary to a section of the 16S rRNA – correctly positions mRNA on the small subunit Translation initiation in E.coli Elongation Peptidyl transferase reaction Growing peptide is added to incoming tRNA’s amino acid residue ie transferred from P site to A site After peptide bond formation, the peptidyl-tRNA is translocated from A site to P site The uncharged tRNA in the P site moves to the E site and dissociate Elongation Polypeptide synthesis occurs from N- N-terminus terminus to C-terminus (peptidyl transferase activity) Chain elongation occurs by linking polypeptide to the incoming tRNA’s amino 5’ acid residue Ribosomes read mRNA in the 5’→3’ direction Translation occurs on polysomes – multiple ribosomes on single mRNA (polyribosome or polysomes = beads-on-a string 3’ Elongation factors in E.coli (EF-Tu and EF-G) Termination Stop codons In E. coli two release factors RF1 and RF2 which recognise stop codons UAG and UGA, respectively Release factors carry a molecule of water to the ribsome that hydrolyses the ester bond between the polypeptide chain and the final tRNA – this releases the polypeptide Ribosome complex is disassembled and the bound tRNA and mRNA released The end product: a polypeptide The end products of protein synthesis is the primary structure of a protein A sequence of amino acid bonded together by peptide bonds The primary structure is determined by the sequences of bases on DNA (genetically defined !) Newly synthesized polypeptide chain is in an unfolded state but rapidly folds to achieve the correct confirmation – probably a stepwise process (main features followed by side chain tweeks)