Mendelian Inheritance Topic 1 PDF
Document Details
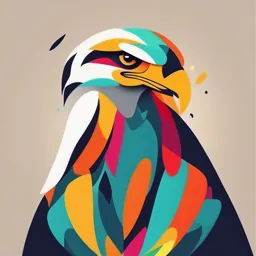
Uploaded by AppreciableDouglasFir
University of Nicosia
Tags
Summary
This document introduces Mendelian inheritance, covering topics such as karyotypes, pedigrees, the laws of segregation and independent assortment, and different types of inheritance. The document also contains learning objectives and recommended reading material.
Full Transcript
Topic 1 Human Genetics: Karyotypes and Pedigrees, Mendelian Inheritance Learning objectives (LOBs) 1. Define and describe the concepts of karyotype and pedigree. 2. Outline the basis of Mendelian genetics. 3. Explain the Mendel laws of segregation and independent assortment. 4. Explain autosomal dom...
Topic 1 Human Genetics: Karyotypes and Pedigrees, Mendelian Inheritance Learning objectives (LOBs) 1. Define and describe the concepts of karyotype and pedigree. 2. Outline the basis of Mendelian genetics. 3. Explain the Mendel laws of segregation and independent assortment. 4. Explain autosomal dominant vs autosomal recessive inheritance. 5. Explain the inheritance of X-linked genetic diseases. 6. Outline the role of genetic testing in identification of human genetic disorders. Recommended reading: Chapter 14, Campbell Biology Overview: Reproduction and inheritance Living organisms are distinguished by their ability to reproduce their own kind Literally, children do not inherit particular physical traits from their parents Offspring acquire genes from parents by inheriting chromosomes It is genes that are actually inherited Inheritance: Terminology Genetics: the scientific study of heredity and variation Heredity: the transmission of traits from one generation to the next Variation: the differences in appearance that offspring show from parents and siblings Chromosomal inheritance: Inheritance of Genes Genes are the units of heredity made up of segments of DNA Genes are passed to the next generation via reproductive cells called gametes (sperm and eggs) Most DNA is packaged into chromosomes Locus: the specific location of each gene on a certain chromosome Mendelian inheritance: Gregor Mendel and Genetics Gregor Mendel: Austrian monk who discovered the basic principles of heredity by breeding garden peas – the father of Genetics Mendel used the scientific approach to identify 2 laws of inheritance: 1. Law of segregation 2. Law of independent assortment Gregor Mendel (July 20, 1822 – January 6, 1884) Mendel’s Experimental Quantitative Approach Mendel made his discoveries using pea plants Advantages of pea plants for genetic study: – Many different characters and traits Characters = distinct heritable features (such as flower colour) Traits = character variants (such as purple or white flowers) – Mating of plants can be controlled – Each pea plant has sperm-producing organs (stamens) and egg-producing organs (carpels) – Cross-pollination: fertilization between different plants; can be achieved by dusting one plant with pollen from another Different characters and traits Traits Character Traits Character Fig. 14-2 Genetic crossings: experimental approach TECHNIQUE 1 Removed stamens (sperm) from purple flowers 2 Transferred sperm bearing pollen from stamens of white flower to egg bearing carpel of purple flower Parental generation (P) Stamens Carpel Pollinated carpel matured into a pod 3 4 Planted seeds from pod RESULTS 5 First filial generation Offspring (F1) Examined offsprings: all purple flowers Mendel’s Experimental Quantitative Approach Genetic crossing: P generation: parental generation F1 generation: first offspring generation (offspring of P generation) F2 generation: second offspring generation (produced by mating F1 individuals) Mendel’s Experimental Approach: The Law of Segregation Hybridization: Mendel mated 2 contrasting true-breeding varieties (white and purple flowered pea plants) True-breeding varieties (homozygotes): plants that produce offspring of the same variants only when they selfpollinate Result of mating of white and purple flowered pea plants: all of the F1 hybrids were purple Result of mating of the F1 hybrids (heterozygotes): a ratio of about 3:1 purple to white flowers in the F2 generation => Purple flower colour: a dominant trait => White flower colour: a recessive trait Fig. 14-3-3 EXPERIMENT P Generation (true-breeding parents) F1 Generation (hybrids) Purple flowers White flowers All plants had purple flowers F2 Generation Ratio of purple to white in the F2 generation= 3:1 705 purple-flowered plants 224 white-flowered plants Mendel’s Experimental Approach: The Law of Segregation Mendelian inheritance: same 3:1 inheritance pattern in 6 other pea plant characters in the F2 generation, each represented by 2 traits Mendel did not know the existence of genes or chromosomes => What Mendel called a “heritable factor” is what we now call a gene Table 14-1 In many traits examined the 3:1 ratio rule Genetic terminology Homozygous: an organism with 2 identical alleles for a character is homozygous for the gene controlling that character (e.g. AA or aa; true-breeding) Heterozygous: an organism that has 2 different alleles for a gene is heterozygous for the gene controlling that character (e.g. Aa; not true-breeding, hybrids) Genetic terminology Phenotype: the physical appearance (characteristics) of an organism Genotype: the genetic make up of an organism Alleles: different versions of the same gene found on the same position on homologous chromosomes and responsible for encoding the same characteristic - Dominant alleles: the ones expressed in the phenotype (represented using capital letter) - Recessive alleles: not expressed in the phenotype when combined with a dominant allele, only expressed when combined with a recessive allele (represented using lower case letter) Alleles on homologous chromosomes Allele 1 (dominant or recessive) Locus for specific gene Homologous pair of chromosomes Allele 2 (dominant or recessive) Fig. 14-4 Mendel’s Model 4 related concepts to explain the 3:1 inheritance pattern he observed in F2 offspring generation Mendel’s model now relates to our knowledge about genes and chromosomes Mendel’s Model: Concept 1 Concept 1: alternative versions of genes account for variations in inherited characters Example: the gene for flower color in pea plants exists in 2 versions (purple flowers and white flowers) These alternative versions of a gene are called alleles Each gene resides at a specific locus (position) on a specific chromosome Alleles on homologous chromosomes Allele for purple flowers Locus for flower-colour gene Allele for white flowers Fig. 14-4 Homologous pair of chromosomes Mendel’s Model: Concept 2 Concept 2: for each character an organism inherits 2 alleles, 1 from each parent Mendel made this deduction without knowing that genes are located on chromosomes Homozygotes: The 2 alleles at a locus on a chromosome are identical (e.g. true-breeding plants) Heterozygotes: the 2 alleles at a locus are different (e.g. the F1 hybrids) Mendel’s Model: Concept 3 Concept 3: if the 2 alleles at a locus differ (in heterozygotes), then one (the dominant allele) determines the organism’s appearance (phenotype), and the other (the recessive allele) has no noticeable effect on appearance Flower-color example: - purple flowers –dominant trait - White flowers- recessive trait Mendel’s Model: Concept 4-The law of segregation Concept 4: The law of segregation - The 2 alleles for a gene (heritable character) separate (segregate) during gamete formation (meiosis) and end up in different gametes Segregation of alleles corresponds to the separation of homologous chromosomes to different gametes during meiosis Gametes (egg or sperm) have only 1 of the 2 homologous chromosomes from each pair that are present in the somatic cells of an organism => only 1 of the 2 alleles Figure 13.7-3 Interphase Separation of homologous chromosomes in meiosis Pair of homologous chromosomes in diploid parent cell P A Duplicated pair of homologous chromosomes M Chromosomes duplicate Sister chromatids Allele separation P= paternal homolog M= maternal homolog a A, a= gene alleles A= dominant allele a = recessive allele Diploid cell with duplicated chromosomes Meiosis I A a A P Meiosis I: separates homologous chromosomes a M 1 Homologous chromosomes separate Haploid cells with duplicated chromosomes Meiosis II 2 Sister chromatids separate A P a A P M Haploid cells with unduplicated chromosomes 50% of gametes have allele A (homolog 1) a M Meiosis II: separates sister chromatids 50% of gametes have allele a (homolog 2) Mendel’s Model: Concept 4 Mendel’s segregation model accounts for the 3:1 ratio observed in the F2 generation of his crosses Punnett square: - a diagram for predicting the results of a genetic cross between individuals of known genetic makeup (genotype) - shows the possible combinations of sperm and eggs A capital letter represents a dominant allele A lowercase letter represents a recessive allele Fig. 14-5-3 P Generation Purple flowers Appearance: PP Genetic makeup: Gametes: White flowers pp p P F1 Generation Appearance: Genetic makeup: Purple flowers Pp 1/ 2 Gametes: 1/ 2 P p Sperm F2 Generation Punnett Square p P P Eggs PP Pp Pp pp Phenotype Ratio 3 purple: 1 white p 3 1 Genotype Ratio 1PP: 2Pp: 1pp Genotype vs Phenotype An organism’s traits do not always reveal its genetic composition due to the different effects of dominant and recessive alleles Example of flower colour in pea plants: - PP and Pp plants have the same phenotype (purple) but different genotypes (PP and Pp) Genotype vs Phenotype Genotype Phenotype Fig. 14-6 3 Phenotype Genotype Purple PP (homozygous) Purple Pp (heterozygous) 1 2 1 Purple Pp (heterozygous) White pp (homozygous) Ratio 3:1 Ratio 1:2:1 1 The Testcross How can we tell the genotype of an individual with the dominant phenotype? Such an individual must have 1 dominant allele, but the individual could be either homozygous or heterozygous The answer is to carry out a testcross: breeding the unknown genotype individual with a homozygous recessive individual If any offspring display the recessive phenotype => the unknown parent must be heterozygous Fig. 14-7 Testcross TECHNIQUE Dominant phenotype, unknown genotype: PP or Pp? Recessive phenotype, known genotype: pp Predictions If PP Sperm p p homozygous P Pp Eggs If Pp Sperm p p or heterozygous P Pp Eggs P Pp Pp pp pp p Pp Pp RESULTS or All offspring purple 1/2 offspring purple and 1/2 offspring white Second Mendel’s law: The Law of Independent Assortment Mendel derived the law of segregation based on the inheritance pattern of 1 character The F1 offspring produced in this cross were monohybrids (individuals that are heterozygous for 1 character) Monohybrid cross: a cross between heterozygotes for 1 character (monohybrids) Mendel identified his second law of inheritance by observing the inheritance pattern of 2 characters at the same time - e.g. seed colour (green or yellow) and seed shape (round or wrinkled) The Law of Independent Assortment Using a dihybrid cross, Mendel developed the law of independent assortment Dihybrid cross: crossing 2 true-breeding parents (homozygotes) differing in 2 characters produces dihybrids in the F1 generation, heterozygous for both characters A dihybrid cross (cross between F1 dihybrids) can determine whether 2 characters are transmitted to offspring as a package (together) or independently If 2 genes are located on the same chromosome => most likely they will be inherited together => law of independent assortment does not apply If 2 genes are located on different chromosomes => they will be inherited independently => law of independent assortment applies Figure 13.10-3 Independent Assortment of Chromosomes Possibility 2 Possibility 1 P1 M1 P2 M2 P1 2 equally probable arrangements of chromosomes at metaphase I M1 P1 M1 M2 P2 P1 M1 M2 P2 Metaphase II P2 M2 Daughter cells Combination 1 Combination 2 P1 and P2 M1 and M2 Combination 3 Combination 4 P1 and M2 2n possible combinations in gametes (n=haploid species chromosome number) M1 and P2 The Law of Independent Assortment The law of independent assortment: each pair of alleles segregates independently of each other pair of alleles during gamete formation This law applies only to genes on non-homologous (different) chromosomes Genes located near each other on the same chromosome tend to be inherited together (dependent assortment of linked genes) Example Are seed colour and shape inherited together or independently? Fig. 15-2. Part A We will follow 2 genes present on different chromosomes: P Generation Green-wrinkled seeds ( yyrr) Yellow-round seeds (YYRR) Y Y=yellow y= green Y r R R y y r Meiosis R=round r=wrinkled Fertilization Gametes R Y y All F1 plants produce yellow-round seeds (YyRr) r All F1 plants produce yellow-round seeds (YyRr) Fig. 15-2. Part B F1 Generation R R y r Y LAW OF SEGREGATION The two alleles for each gene separate during gamete formation. y r Y LAW OF INDEPENDENT ASSORTMENT: Alleles of genes on non-homologous chromosomes assort independently during gamete formation. Meiosis r R r R Y y Metaphase I Y y 1 1 R r r R Y y Anaphase I Y y r R Metaphase II R r 2 2 Gametes R R 14 F 2 y Y Y Generation YR r r r A n F yr 1 r 14 F 1 Y Y y 14 y Y y Y Yr y y R R 14 yR cross-fertilization 3 3 9 : 3 : 3 : 1 Fig. 15-2 P Generation Yellow-round seeds (YYRR) Y Y R Green-wrinkled seeds (yyrr) y r R y r Meiosis Fertilization y RY Gametes r All F1 plants produce yellow-round seeds (YyRr) F1 Generation R R y r Y Y LAW OF SEGREGATION The two alleles for each gene separate during gamete formation. y r LAW OF INDEPENDENT ASSORTMENT Alleles of genes on non-homologous chromosomes assort independently during gamete formation. Meiosis R r Y y r R Y y Metaphase I 1 1 R r Y y r R Y y Anaphase I R r Y y Metaphase II r R Y y 2 2 Y Y Gametes R 1/ YR 4 F2 Generation R y y r 1/ 4 Y Y r r r 1/ yr 4 Yr y R y R 1/ yR 4 An F1 F1 cross-fertilization 3 3 9 :3 :3 :1 Fig. 14-8 Y= yellow (dominant) y=green (recessive) R=round (dominant) r= wrinkled (recessive) EXPERIMENT YYRR P Generation Yellow Round yyrr Gametes YR F1 Generation Green wrinkled yr YyRr Hypothesis of dependent assortment If genes are located on the same chromosomes Hypothesis of independent assortment If genes are located on different chromosomes Predictions Sperm or Predicted offspring of F2 generation 1/ 4 Sperm 1/ 1/ YR 2 yr 2 1/ 4 1/ 2 Yr 1/ 4 yR 1/ 4 yr YR YYRR YYRr YyRR YyRr YYRr YYrr YyRr Yyrr YyRR YyRr yyRR yyRr YyRr Yyrr yyRr yyrr YR YYRR Eggs 1/ 2 YyRr 1/ 4 Yr Eggs yr YyRr 3/ 4 yyrr 1/ 4 yR 1/ 4 1/ 4 Phenotypic ratio 3:1 yr 9/ 16 108 3/ 16 3/ 16 1/ 16 Phenotypic ratio 9:3:3:1 RESULTS 315 YR 1/ 4 101 32 Phenotypic ratio approximately 9:3:3:1 Figure 13.7-3 Interphase Separation of homologous chromosomes in meiosis Pair of homologous chromosomes in diploid parent cell Duplicated pair of homologous chromosomes P Y- M -y R- -r Chromosomes duplicate Sister chromatids Allele separation P= paternal homolog M= maternal homolog Y/y, R/r = gene alleles YR= dominant alleles yr = recessive alleles Diploid cell with duplicated chromosomes Meiosis I y--y Y- -Y P R- -R 1 Homologous chromosomes separate M R- -r Haploid cells with duplicated chromosomes Meiosis I: separates homologous chromosomes Meiosis II 2 Sister chromatids separate y-Y M P P rR-R Haploid cells with unduplicated chromosomes Y- -y M -r Meiosis II: separates sister chromatids 50% of gametes have alleles YR (homolog 1) 50% of gametes have alleles yr (homolog 2) Figure 13.10-3 Independent Assortment of Chromosomes Possibility 2 Possibility 1 P1 Y- -y 2 equally probable arrangements of chromosomes at metaphase I M1 P2 R- - r M2 Y- P1 R- P2 M1 M2 -y -r P1 Y- -y M1 r- -R P2 M2 Y- P1 M1 -y r- M2 P2 -R Metaphase II Daughter cells Combination 1 Combination 2 P1 and P2 YR M1 and M2 yr Combination 3 Combination 4 P1 and M2 Yr M1 and P2 yR YyRr x YyRr 1/ 1/ 1/ 1/ 1/ 4 4 4 4 YR Yr yR yr YR Yr YYRR YYRr 1/ 4 4 1/ 9 yellow round: 3 yellow wrinkled 3 green round: 1 green wrinkled / yr yR YyRR YyRr 1 4 4 1/16 1/16 1/16 1/16 YYRr YYrr YyRr YYrr 1/16 1/16 1/16 YyRR 1/16 YyRr 1/16 YyRr yyRR 1/16 yyRr 1/16 1/16 1/16 Yyrr yyRr yyrr 1/16 1/16 1/16 The laws of probability govern Mendelian inheritance Mendel’s laws of segregation and independent assortment reflect the probability rules of multiplication and addition Independent events: when tossing a coin, the outcome of one toss has no impact on the outcome of the next toss In the same way, the alleles of one gene segregate into gametes independently of another gene’s alleles Inheritance patterns are often more complex than predicted by simple Mendelian genetics The relationship between genotype and phenotype is rarely as simple as in the pea plant characters Mendel studied Many heritable characters are not determined by only 1 gene with 2 alleles However, the basic principles of segregation and independent assortment apply even to more complex patterns of inheritance Extending Mendelian Genetics for a Single Gene Inheritance of characters by a single gene may deviate from simple Mendelian patterns in the following situations: – When alleles are not completely dominant or recessive – When a gene has more than 2 alleles – When a gene produces multiple phenotypes Degrees of Dominance Complete dominance: occurs when phenotypes of the heterozygote and dominant homozygote are identical (expression of dominant allele phenotype) Incomplete dominance: when the phenotype of heterozygotes (F1 hybrids) is somewhere between the phenotypes of the 2 parental phenotypes (varieties) (neither allele is expressed; intermediate phenotype) Codominance: 2 dominant alleles affect the phenotype in separate distinguishable ways (both alleles are expressed) Incomplete dominance The heterozygous offspring have an intermediate phenotype Examples: Palomino horses Certain flower types (carnation): Genetic crossing of red and white flowers gives pink offspring Fig. 14-10-3 P Generation Incomplete dominance Red CRCR White CW CW CR Gametes CW Pink CRCW F1 Generation Gametes 1/2 CR 1/ 2 CW Sperm 1/ 2 CR 1/ 2 CW F2 Generation 1/ 2 CR Eggs 1/ 2 CRCR CRCW CRCW CW CW CW Incomplete Dominance: the phenotype of F 1 hybrids is somewhere between the phenotypes of the two parental varieties Codominance Codominance: Both alleles are expressed in the phenotype Example: human blood group types (3 alleles) A and B= codominant alleles O = recessive allele – ΑΑ or ΑΟ genotypes = Blood group A – ΒΒ or ΒΟ genotypes = Blood group B – ΟΟ genotype = Blood group O – ΑΒ genotype = Blood group AB Codominance: blood group inheritance Multiple Alleles: when a gene has more than 2 alleles Most genes exist in populations in more than 2 allelic forms Example: human blood group types - Determined by red blood cell membrane carbohydrates - The 4 phenotypes of the ABO blood group (A, B, AB, O) in humans are determined by three alleles for the enzyme (I) that attaches A or B carbohydrates to red blood cells: IA, IB, and i. The enzyme encoded by the IA allele adds the A carbohydrate The enzyme encoded by the IB allele adds the B carbohydrate The enzyme encoded by the i allele adds neither Fig. 14-11 Allele IA IB Carbohydrate A B i none (a) The three alleles for the ABO blood groups and their associated carbohydrates Genotype Red blood cell appearance Phenotype (blood group) IAIA or IA i A IBIB or IB i B I AI B AB ii O (b) Blood group genotypes and phenotypes The Relation Between Dominance and Phenotype A dominant allele does not subdue a recessive allele; alleles don’t interact Alleles are simply variations in a gene’s nucleotide sequence For any character, dominance/ recessiveness relationships of alleles depend on the level at which we examine the phenotype Frequency of Dominant Alleles Dominant alleles are not necessarily more common in populations than recessive alleles For example, 1 baby out of 400 in the United States is born with extra fingers or toes The allele for this unusual trait is dominant to the allele for the more common trait of 5 digits per appendage In this example, the recessive allele is far more prevalent than the population’s dominant allele Recessive allele= 5 fingers, more common Dominant allele= 6 fingers, less common Pleiotropy Pleiotropy: property of most genes to have multiple phenotypic effects Example: pleiotropic alleles are responsible for the multiple symptoms of certain hereditary diseases, such as cystic fibrosis and sickle-cell disease Extending Mendelian Genetics for Two or More Genes Some traits may be determined/controlled by 2 or more genes Examples: Epistasis: the phenomenon where the effects of 1 gene are modified by other genes Polygenic inheritance: when 2 or more genes control the expression of a single phenotype Epistasis Epistasis: the phenomenon where the effects of 1 gene are modified by 1 or several other genes - when a gene at one locus alters the phenotypic expression of a gene at a second locus => Result: 2 different gene sets affect the same phenotypic characteristic Example: in mice and many other mammals coat color depends on two genes -One gene determines the pigment color (with alleles B for black and b for brown) -The other gene (with alleles C for colour and c for no colour) determines whether the pigment will be deposited in the hair Epistasis: mouse coat colour B = black colour (dominant); b = brown colour (recessive) C allele = dominant allele that allows the expression of other genes responsible for coat colour c allele = recessive allele that inhibits the expression of other genes responsible for coat colour cc homozygotes: no coat colour => white hair The genotype «cc» inhibits the expression of other genes controlling coat colour (e.g. B/b) The genotypes “ CC” and “Cc” allow the expression of other genes controlling coat colour (e.g. B/b) Epistasis B = black (dominant) b = brown (recessive) c = white (no colour; recessive) C= with colour (dominant) BB= black color BbCc bb= brown color BbCc Bb=black color Sperm 1/ 4 BC 1/ 4 bC 1/ 4 Bc 1/ 4 bc Eggs 1/ cc= no pigment deposition 4 BC BBCC 1/ 1/ 1/ CC= pigment deposition BbCC BBCc BbCc 4 bC BbCC bbCC BbCc bbCc BBCc BbCc BBcc Bbcc BbCc bbCc Bbcc bbcc 4 Bc 4 bc 9 : 3 : 4 The gene for pigment deposition (C/c) is epistatic to the gene that codes for black or brown color (B/b) => homozygous for cc will be white Epistasis Human disease example: albinism Albinism: congenital autosomal recessive disorder characterized by the complete or partial absence of pigment in the skin, hair and eyes - due to absence or defect of tyrosinase, the enzyme responsible for the production of melanin The albinism allele in humans inhibits the expression of genes responsible for hair and eye colour Albinism Polygenic Inheritance Polygenic inheritance: when 2 or more genes control the expression of a single phenotype => additive effects Polygenic inheritance is usually characterised by quantitative variation Quantitative characters: characters that vary in the population along a continuum (continuous variation) Example: height or skin colour in humans is an example of polygenic inheritance Fig. 14-13. Polygenic inheritance example: Skin colour inheritance AaBbCc AaBbCc Sperm Skin pigmentation is inherited by at least 3 separately inherited genes (6 alleles) 1/ Eggs 1/ 8 1/ 8 1/ 8 1/ 8 1/ 1/ 8 1/ 1/ 8 8 1/ 8 1/ 64 15/ 8 1/ 1/ 8 8 8 1/ 8 1/ 8 1/ 8 8 Phenotypes: Number of dark-skin alleles: 1/ 64 0 6/ 64 1 15/ 64 2 20/ 3 64 4 6/ 64 5 1/ 64 6 Polygenic inheritance of skin colour: Quantitative (continuous) variation The Environmental Impact on Phenotype ▪ External and internal conditions that can affect some phenotypes: - temperature - chemicals - nutritional habits The Environmental Impact on Phenotype Multifactorial characters: both genetic and environmental factors influence phenotype - Some phenotypes for a character depend on environment as well as genotype Norm of reaction: the phenotypic range of a genotype influenced by the environment => broadest for polygenic characters Example: hydrangea flowers of the same genotype range from blue-violet to pink, depending on soil acidity Integrating a Mendelian View of Heredity and Variation An organism’s phenotype includes its physical appearance, internal anatomy, physiology, and behaviour An organism’s phenotype reflects its overall genotype and unique environmental history Many human traits follow Mendelian patterns of inheritance Humans are not good subjects for genetic research – Generation time is too long – Parents produce relatively few offspring – Breeding experiments are unacceptable However, basic Mendelian genetics is the foundation of human genetics Sets of Chromosomes in Human Cells Karyotype: an ordered display of the pairs of chromosomes from a cell Human somatic cells (any cell other than a gamete) have 23 pairs of chromosomes (diploid, 2n) The 2 chromosomes in each pair are called homologous chromosomes (homologs) Homologous chromosomes: - Have the same length and shape - Carry genes controlling the same inherited characters - Do not necessarily carry the sames alleles Figure 13.3c Human Karyotype 5 m Each somatic cell = 44 autosomes (22 pairs) + 2 sex chromosomes XX (if female) or XY (if male) = 46 chromosomes 74 Sets of Chromosomes in Human Cells Human karyotype: consists of 22 pairs of autosomes and 1 pair of sex chromosomes Sex chromosomes: determine the sex of the individual and are called X and Y - Human females have a homologous pair of X chromosomes (XX) - Human males have one X and one Y chromosome (XY) The remaining 22 pairs of chromosomes are called autosomes Autosomes: chromosomes that do not determine the sex Pedigree Analysis A pedigree is a family tree that describes the interrelationships of parents and children across generations Inheritance patterns of particular traits can be traced and described using pedigrees Pedigrees can also be used to make predictions about future offspring We can use the probability rules to predict the probability of specific phenotypes Fig. 14-15a Pedigree Analysis Key Male Female Affected male Affected female Mating Offspring, in birth order (first-born on left) Fig. 14-15b Pedigree Analysis: Example 1 1st generation (grandparents) 2nd generation (parents, aunts, and uncles) Ww ww ww Ww ww ww Ww Ww Ww ww 3rd generation (two sisters) WW or Ww Widow’s peak ww No widow’s peak (a) Is a widow’s peak a dominant or recessive trait? Dominant trait: 2 affected parents have unaffected child Fig. 14-15c 1st generation (grandparents) 2nd generation (parents, aunts, FF or Ff and uncles) Ff Ff ff ff ff Ff Ff Ff ff ff FF or Ff 3rd generation (two sisters) Pedigree Analysis: Example 2 Attached earlobe Free earlobe (b) Is an attached earlobe a dominant or recessive trait? Recessive trait: 2 unaffected parents have affected child Human Genetic Disorders A lot of human diseases are inherited Can be studied using pedigree analyses Endogamy in kingdoms Habsburg lip Inherited human disorders Autosomal dominant disorders Autosomal recessive disorders Sex-chromosome linked disorders Inherited human disorders Disease Frequency Autosomal dominant Familial hypercholesterolaemia 1 in 500 Polycystic kidney disease 1 in 1250 Huntington disease 1 in 2,500 Hereditary spherocytosis 1 in 5,000 Marfan syndrome 1 in 20,000 Autosomal recessive Sickle cell anaemia 1 in 625 (African Americans) Cystic fibrosis 1 in 2,000 (Caucasians) Tay-Sachs disease 1 in 3,000 (American Jews) Phenylketonuria 1 in 12,000 Mucopolysaccharidoses 1 in 25,000 Glycogen storage diseases 1 in 50,000 Galactosaemia 1 in 57,000 X- linked recessive Duchenne muscular dystrophy 1 in 7,000 Haemophilia 1 in 10,000 Values are for liveborn infants Recessively Inherited Disorders Many genetic disorders are inherited in a recessive manner Recessively inherited disorders show up only in individuals homozygous for the allele Carriers are heterozygous individuals who carry the recessive allele but are phenotypically normal Example: Albinism is a recessive disorder characterized by a lack of pigmentation in skin and hair Fig. 14-16 Albinism Parents Normal Aa Normal Aa Sperm A a A AA Normal Aa Normal (carrier) a Aa Normal (carrier) aa Albino Eggs Autosomal recessive disorders Due to a mutation in an allele located in one of the 22 pairs of autosomes The mutant allele is recessive (a) Α= normal allele (dominant) a = disease (abnormal) allele (recessive) ΑΑ and Αa genotypes= healthy individuals aa genotype= individuals with the disease Autosomal recessive disorders The most common type of genetic disorders May not appear in the phenotype, but the parents may be heterozygous healthy carriers of the disease allele Examples: cystic fibrosis, sickle cell anaemia, thalassaemia, albinism Two heterozygous carriers (Aa) of the allele have a probability of 25% to produce an offspring with the disease (aa) Autosomal recessive disorders Normal allele Α Mutant (disease) allele α Follow the Mendelian inheritance pattern Autosomal recessive disorders More common than autosomal dominant disorders because the disease alleles “hide” in the healthy heterozygous carriers which have a normal phenotype In autosomal dominant disorders, the disorder is obvious as the phenotype is affected The Behavior of Recessive Alleles If a recessive allele that causes a disease is rare, then the chance of two carriers meeting and mating is low Consanguineous matings: - matings between close relatives -increase the chance of mating between 2 carriers of the same rare allele Most societies and cultures have laws or taboos against marriages between close relatives Sickle-Cell Disease: Autosomal recessive disorder Sickle-cell disease affects 1 out of 400 African-Americans The disease is caused by the substitution of a single amino acid (Glu→ Val) in the haemoglobin protein in red blood cells Symptoms: physical weakness, pain, organ damage, and even paralysis Heterozygotes (carriers of the sickle-cell disease allele) are usually healthy but may suffer some symptoms 10% of African Americans have sickle cell trait (allele) Heterozygotes are less susceptible to the malaria parasitethere was a survival advantage for being heterozygous in the mediterranean/sub-tropical regions (natural selection) Sickle cell anaemia - Due to a mutation in the haemoglobin gene => sickle shaped RBC - Autosomal recessive inheritance Ηaemoglobin Haemoglobin is a globular protein consisting of four polypeptides: 2 alpha and 2 beta chains β Chains Iron Heme α Chains Hemoglobin Fig. 5-22 Normal hemoglobin Primary structure Val His Leu Thr Pro Glu Glu 1 2 3 4 5 6 7 Secondary and tertiary structures β subunit Normal hemoglobin (top view) 1 2 3 Normal red blood cells are full of individual hemoglobin moledules, each carrying oxygen. 6 7 β subunit β Sickle-cell hemoglobin α Function α Molecules interact with one another and crystallize into a fiber; capacity to carry oxygen is greatly reduced. 10 µm Red blood cell shape 5 Exposed hydrophobic region β Molecules do not associate with one another; each carries oxygen. 4 α Quaternary structure β Function Secondary and tertiary structures Val His Leu Thr Pro Val Glu β α Quaternary structure Sickle-cell hemoglobin Primary structure 10 µm Red blood cell shape Fibers of abnormal hemoglobin deform red blood cell into sickle shape. Fig. 5-22c 10 µm Normal red blood cells are full of individual hemoglobin molecules, each carrying oxygen. 10 µm Fibers of abnormal hemoglobin deform red blood cell into sickle shape. Sickle cell anaemia Thalassaemia Due to a mutation in the haemoglobin gene (α or β chains => α or β thalassaemia) 25% chance that the offspring will have thalassaemia Cystic Fibrosis: Autosomal recessive disorder Cystic fibrosis is the most common lethal genetic disease in the United States, striking 1 in 2,500 people of European descent The cystic fibrosis allele results in defective or absent chloride transport channels in plasma membranes Characterised by scarring (fibrosis) and cyst formation within the pancreas Symptoms include mucus buildup in some internal organs and abnormal absorption of nutrients in the small intestine Cystic Fibrosis: Autosomal recessive disorder Clinical correlations: transporter protein disorders Batzios S, Tal G, DiStasio AT, Peng Y, Charalambous C, Nicolaides P, Kamsteeg EJ, Korman SH, Mandel H, Steinbach PJ, Yi L, Fair SR, Hester ME, Drousiotou A, Kaler SG. Newly identified disorder of copper metabolism caused by variants in CTR1, a highaffinity copper transporter. Human Molecular Genetics. 2022; 00 (00): 1–10. CTR-1 deficiency: Autosomal recessive disorder CTR-1: Copper transporter protein 1 Channel protein located mostly on intestinal epithelial cells responsible for copper (Cu+2) absorption CTR-1 deficiency: newly discovered autosomal recessive disorder due to mutations in CTR-1 protein Mutations result in defective copper transport protein Copper: essential trace element responsible for embryonic development, myelin production, mitochondrial/cellular respiration enzyme function Symptoms: neurodegenerative disorder, severe developmental delay Autosomal dominant disorders Less common than autosomal recessive disorders: -The affected individuals usually do not survive until adulthood to produce offspring Aa Aa aa aa Aa - Even if they survive, the disorder presents in the phenotype (=> against the natural selection principles) Disease allele Α Normal allele a aa Autosomal dominant disorders Autosomal dominant disorders are rare because affected individuals usually die before mating and passing the gene on Aa Exception: disorders that express symptoms late in life (late onset of symptoms) - Example: Huntington disease Aa aa aa Aa Disease alleleΑ Normal allele a aa Autosomal dominant disorders Some human disorders are caused by dominant alleles Dominant alleles that cause a lethal disease are rare and arise by mutation Examples: Huntington’s disease: neurodegenerative disorder Achondroplasia: a form of dwarfism caused by a rare dominant allele Fig. 14-17 Achondroplasia Parents Dwarf Dd Normal dd Sperm D d d Dd Dwarf dd Normal d Dd Dwarf dd Normal Eggs Huntington’s Disease Huntington’s disease is a degenerative disease of the nervous system The disease has no obvious phenotypic effects until the individual is about 35 to 40 years of age (late onset) The timing of onset of the symptoms of a disease significantly affects its inheritance (late onset allows mating and offspring production) Once the deterioration of the nervous system begins the condition is irreversible and fatal Huntington’s Disease Sex chromosome linked disorders Due to mutations on the alleles on the sex chromosomes Since the Υ chromosome has very few genes, mostly due to mutations on genes on the X chromosome (called Xlinked disorders) Usually, can be either X-linked dominant or X-linked recessive Very few Y-linked disorders X-linked recessive disorders are much more common than X-linked dominant disorders X-linked recessive disorders are much more common in men than women (due to the absence of the allele on the Y chromosome) X-linked recessive disorders Disorder allele on the Χ chromosome ▪ Absence of a normal allele on the Y chromosome If the mother is the carrier, 50% of her sons is affected Women are rarely affected by the disorder - Normal allele XA: dominant - Mutant allele Xα: disease allele (recessive) X-linked recessive disorders Males affected much more than females Τhe mutant allele is recessive (α) and located on the Χ chromosome Examples: haemophilia, colour blindness, Duchenne muscular dystrophy ΧΑΧΑ and ΧΑΧα = normal woman (healthy female ) ΧαΧα = affected woman (woman with the disease) ΧΑΥ = normal male ΧαΥ= male with the disease X-linked recessive disorders X-linked dominant disorders Less common than X-linked recessive disorders Extremely rare, usually fatal The males are not affected more than females Τhe mutant allele is dominant (Α) and found on the Xchromosome Examples: Vitamin D resistant (hypophosphataemic) rickets, Alport syndrome ΧΑΧΑ and ΧΑΧα = affected females ΧαΧα = normal female ΧΑΥ = affected male ΧαΥ= normal male X-linked dominant disorders All daughters affected, all sons healthy 50% of the children affected (sons or daughters) Multifactorial Disorders Many diseases, such as heart disease and cancer, have both genetic and environmental components Little is understood about the genetic contribution to most multifactorial diseases Genetic Testing and Counseling Genetic counselors can provide information to prospective parents concerned about a family history for a specific disease Using family histories (pedigrees), genetic counselors help couples determine the odds that their children will have genetic disorders Genetic counseling is based on Mendelian genetics and probability rules Carrier detection: tests for identifying carriers Genetic tests are available that identify carriers of various diseases and help define the odds that the offspring will have the disorder Carrier detection example: β-thalassaemia - 16 % of the Cypriot population are heterozygous carriers of the βthalassaemia trait (allele) - Screening policy in Cyprus to reduce the incidence of βthalassemia since the 1970s: - includes premarital screening, prenatal screening and abortion (recently also includes the option of in vitro fertilisation with healthy embryo) - has reduced the number of children born with the hereditary blood disease from 1 in 158 births to almost zero Similar screening policies in other countries (e.g. Iran) Prenatal screening Prenatal screening: testing for diseases or conditions in a fetus or embryo before it is born Detect birth defects such as: - Chromosomal abnormalities (e.g. Down syndrome) by karyotyping - Genetic disorders (sickle cell anaemia, thalassemia, cystic fibrosis, muscular dystrophy, etc) by genetic testing Prenatal screening: Fetal Testing Amniocentesis: the amniotic fluid is removed and tested Chorionic villus sampling (CVS): a sample of the placenta is removed and tested Non-Invasive Prenatal Diagnosis (NIPD): a sample of mother’s blood, which contains some fetal cells, is removed and tested (new genetic test) All 3 techniques are followed by karyotyping (or genetic testing) of fetal cells which can reveal possible abnormalities Other techniques, such as ultrasound and fetoscopy, allow fetal health to be assessed visually in utero Fig. 14-18 Amniotic fluid withdrawn Centrifugation Fetus Fetus Placenta Uterus Placenta Cervix Suction tube inserted through cervix Chorionic villi Fluid Fetal cells Several hours Biochemical tests Several weeks Several weeks (a) Amniocentesis Karyotyping Several hours Fetal cells Several hours (b) Chorionic villus sampling (CVS) Newborn Screening Some genetic disorders can be detected at birth by simple tests that are now routinely performed in most hospitals Example of a newborn screening program: phenyketonuria - Autosomal recessive disorder (1 in 10 000/15 000 births) - Cannot metabolize the aminoacid phenylalanine Accumulation in blood and urine mental retardation - Early detection: treatment with diet low in phenylalanine Figure 14.UN03 Relationship among alleles of a single gene Complete dominance of one allele SUMMARY Description Heterozygous phenotype same as that of homozygous dominant Incomplete dominance Heterozygousphenotype intermediate between of either allele the two homozygous phenotypes Codominance Both phenotypes expressed in heterozygotes Example PP Pp CRCR CRCW CWCW IAIB Multiple alleles In the whole population, some genes have more than two alleles Pleiotropy One gene is able to affect Sickle-cell disease multiple phenotypic characters ABO blood group alleles IA, IB, i Figure 14.UN04 Relationship among two or more genes Epistasis SUMMARY Description The phenotypic expression of one gene affects that of another Example BbEe BE BbEe bE Be be BE bE Be be 9 Polygenic inheritance A single phenotypic character is affected by two or more genes AaBbCc :3 :4 AaBbCc Summary of Mendelian genetics: Mendel's laws of inheritance Law Definition Law of segregation (first law) During gamete formation, the alleles for each gene segregate from each other so that each gamete carries only one allele for each gene. Law of independent assortment (second law) Genes for different traits can segregate independently during the formation of gametes. SUMMARY OF GENETIC DISORDERS Inheritance mode Genetic disorder Autosomal recessive Cystic fibrosis Sickle cell anaemia α/β thalassaemia Albinism Autosomal dominant Huntington’s disease Achondroplasia X-linked recessive Ηaemophilia Colour blindness Duchenne muscular dystrophy X-linked dominant Vitamin D resistant rickets Alport syndrome Y-linked Swyer syndrome (XY gonadal dysgenesis) Mitochondrial disorders Mitochondrial myopathy, Leber’s optic neuropathy Topic 1 Topic 2 SBA example 1 A red carnation and a white carnation give offsprings that are all pink. The type of inheritance pattern observed is: A. Complete dominance B. Incomplete dominance C. Codominance SBA example 2 If the allele for tall plants (T) is dominant and the allele for short plants is recessive (t), what offsprings would you expect from a ΤΤ x Tt crossing? A. ½ tall; ½ short B. ¾ tall; ¼ short C. All tall SBA example 3 Τhe hair colour in rabbits shows an incomplete dominance pattern of inheritance BB rabbits are brown BW rabbits are beige WW rabbits are white What are the expected phenotypes of the offsprings of the BW x WW crossing? A. 3 white: 1 brown B. 3 white: 1 beige C. 2 white: 2 beige SBA example 4 Which of the following statements describes best autosomal dominant disorders? Α. They are associated with Χ-chromosome linked genes Β. Their symptoms often have a late onset C. They can occur due to the mating of heterozygous healthy carriers D. They occur more frequently than autosomal recessive disorders SAQ example: Law of independent assortment Find the genotypes and phenotypes of the offsprings of the crossing RrYy x rrYy R= round seeds Υ= yellow seeds r= wrinkled seeds y= green seeds F1 generation (offsprings) x RY Ry rY ry rY RrYY RrYy rrYY rrYy ry RrYy Rryy rrYy rryy rY RrYY RrYy rrYY rrYy ry RrYy Rryy rrYy rryy 3 yellow round: 3 yellow wrinkled:1 green round: 1 green wrinkled