Theme 2 Module 4 Script PDF
Document Details
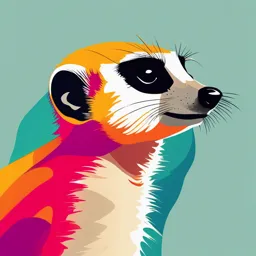
Uploaded by ExquisiteAntigorite1841
null
Tags
Summary
This document is a script of a lecture or presentation on the complex proteome. It covers learning objectives, the human proteome, protein synthesis and processing, and cellular responses to stimuli such as glucose. The script details the role of proteins, genes and the interactions between them in regulating cellular processes.
Full Transcript
Theme 2 Module 4 Script 1 Slide 1: Theme 2, Module 4: The complex proteome YOUR SUMMARY NOTES: Slide 2: The learning objectives in this module are to: Identify how protein diversity relates to the fact...
Theme 2 Module 4 Script 1 Slide 1: Theme 2, Module 4: The complex proteome YOUR SUMMARY NOTES: Slide 2: The learning objectives in this module are to: Identify how protein diversity relates to the fact that one gene can code for many proteins Examine how protein activity can be controlled based on post-translational modifications and relative location Describe the importance of alternate protein forms Understand how interacting proteins can lead to complex and regulated cellular responses Slide 3: Unit 1: From genome to proteome Slide 4: The human proteome represents the full number of proteins that are expressed by all the hereditary information in our DNA (also referred to as our genome). While the human genome project has revealed only 20-25,000 protein encoding genes, we have identified that there are other regulatory mechanisms that contribute to the over 1,000,000 proteins that are encoded by our genome. This suggests that single genes can encode multiple proteins. The great complexity of our proteome relative to our genome is largely attributed to RNA processing and post-translational modifications. Theme 2 Module 4 Script 2 Slide 5: YOUR SUMMARY NOTES: One of the defining characteristics of eukaryotes is the segregation of genetic information inside the double membraned nuclear envelope. Transcription of DNA into RNA, along with RNA processing also occurs within the nucleus. The double membrane of the nucleus is continuous and presumably evolved from the membranous network of the single-membrane endoplasmic reticulum. In addition to all the other membrane enclosed compartments of the eukaryotic cells, compartmentalization allows for a more intricate control in the regulation of cellular processes. As seen in eukaryotes, following transcription from protein coding genes, mRNAs are prepared for translation. Mature mRNAs are then exported out of the nucleus and into the cytosol where free or ER bound ribosomes facilitate translation into polypeptides. The cascade of events that occur from reading and interpreting the genomic information in the DNA blueprint to the final processing and translation of this message are essential to produce a functional protein. During these events, alternative splicing and post- translational modifications further contribute to the complexity of the proteome. The composition of our proteome can change in response to various factors. This can include an organism’s developmental stage and especially in response to internal and external signals. Slide 6: Unit 2: Detecting a signal Theme 2 Module 4 Script 3 Slide 7: Cells are able to detect changes in their YOUR SUMMARY NOTES: environment in numerous ways. These changes often serve as stimuli that will result in important cellular responses. Take for example what occurs in your body following a meal. Many cells will be sensitive and respond to this stimulus. The stimulus can include for example, an increase in blood glucose levels. These glucose levels are regulated in our body. This regulation comes about due to sensory responses in specialized Beta islet cells of the pancreas that will lead to a cascade of events that can return measured blood glucose back to normal levels. In response to a high blood glucose levels, the pancreas will modulate the synthesis and secretion of an increased amount of its own signal. A protein called insulin. Insulin is the effector protein that is produced by the pancreatic beta cells that is then able to communicate with and produce a response on target cells, and therefore lead to a decrease in blood glucose levels. The process from stimulus to a cellular response during this cascade of events is highly regulated and is dependent on the action of important proteins and cell to cell communication. Slide 8: Following a meal, glucose is absorbed into the bloodstream. While some glucose absorption can occur in the mouth across thin epithelial surfaces that are intimately associated with underlying blood vessels or capillaries, a large amount of glucose absorption occurs in the microvilli cells of the small intestine. Slide 9: The microvilli cells of the small intestine are also intimately associated with very small blood vessels. These microvilli absorb the glucose that is found within the intestinal tract, and from there, the absorbed glucose molecules are transported into the blood vessels. The glucose molecules will then travel through the circulatory system. After a meal, the specialized pancreatic beta cells are able to detect an increase in blood glucose levels and adjust the amount of synthesis and secretion of the insulin protein. It is this insulin protein that will act as an effector to help reduce blood glucose levels. How is this protein secretion regulated? Slide 10: Checkpoint question #1 Theme 2 Module 4 Script 4 Slide 11: YOUR SUMMARY NOTES: Unit 3: The release of functional proteins Slide 12: Insulin biosynthesis, much like many other proteins, is regulated at both the transcriptional and translational levels. While many factors will control insulin biosynthesis, glucose metabolism is an important physiological event which leads to an increase in insulin gene transcription and mRNA translation. Seen in this image is an electron micrograph showing the dense rough endoplasmic reticulum that can be found within a beta cell of the pancreas. Because insulin is secreted from the cells it is within this dense endoplasmic reticulum network that the insulin protein is produced. Slide 13: Insulin is a small protein. The translated polypeptide that is coded in the insulin gene is 110 amino acids in length. However, the functional insulin protein that is secreted from the beta cells of the pancreas is made up of a total of 51 amino acids. Yet this does not add up to the 110 amino acid sequence that we initially translated from the insulin gene. What leads to this difference? In 1969, using X-ray crystallography techniques, Dorothy Hodgkin was able to determine the structure of the functional insulin protein. It is in fact made up of two amino acid chains. An alpha chain that is 21 amino acids in length and a beta chain that is 30 amino acids length. These two amino acid chains form a dimer that makes up the functional insulin protein. The processing of the insulin protein from a single polypeptide of 110 amino acids to a protein structure containing 2 polypeptides of 21 and 30 amino acids is achieved by post- translational modifications. Slide 14: The insulin gene encodes a 110-amino acid precursor of the mature insulin protein known as preproinsulin. Much like other proteins that are translated by bound ribosomes and then processed within the endoplasmic reticulum, preproinsulin contains an N-terminal signal sequence which interacts with signal recognition particles (or SRP particles) to facilitate translocation of preproinsulin into the lumen of the rough endoplasmic reticulum. Initially, the preproinsulin is processed through cleavage of the signal sequence, and as a result yielding a proinsulin molecule. Theme 2 Module 4 Script 5 Slide 15: YOUR SUMMARY NOTES: Proinsulin is further modified by other post- translational modifications in order to obtain the mature insulin protein that is secreted from the pancreatic beta cells. Proinsulin will undergo folding in addition to the formation of three disulphide bonds. The process of accurate protein folding requires the assistance of chaperone proteins that are found within the rough endoplasmic reticulum. The folded proinsulin protein is then transported from the rough endoplasmic reticulum to the Golgi apparatus, where further cleavage occurs, forming the mature insulin dimer (containing both the A and B chains) and in the process, releasing a small C-chain. These post-translational modifications of the preproinsulin peptide into the mature insulin protein are crucial, for it is only when these modifications occur that the N- terminal and C-terminal amino acid residues in the A and B chains are able to bind to the insulin receptors on the target cells. Slide 16: Cleavage, as seen with insulin is just one of many post-translational modifications that can be done to many translated proteins. And it is these post-translational modifications that will increase the functional diversity of the proteome. Aside from cleavage, folding and disulphide bridge formation, other modifications can also include the covalent attachment of other molecules and even degradation of entire proteins. Three examples of a covalent attachment of other molecules include phosphorylation, methylation and acetylation. Phosphorylation is a reversible post-translational modification that involves the covalent attachment of a phosphate group to serine, threonine or tyrosine amino acid residues in a protein by enzymes called kinases. Similarly, methylation involves the covalent addition of a methyl group, while acetylation involves the addition of an acetyl group to a specific amino acid residue in a protein. Ultimately, the myriad of post-translational modifications that can occur to various proteins adds to the production of mature and functional proteins that are synthesized in a cell. Slide 17: Checkpoint question #2 Theme 2 Module 4 Script 6 Slide 18: YOUR SUMMARY NOTES: Unit 4: Different protein forms are important Slide 19: Once the pancreatic beta cells release insulin in response to increases in blood glucose levels, these insulin effector molecules will bind to receptors that are expressed on specific target tissues. Receptors are important proteins that receive and interpret information from such signaling molecules like insulin. There are thousands of different types of cellular receptors, each with their ability to bind to specific signals or ligands and subsequently produce a response within the cell that is receiving the signal. The insulin protein will bind to specific insulin receptors that fall into a family of receptors called receptor kinases. The binding of insulin to these receptors enables many cells in our body to transport glucose across the plasma membrane into the cytosol of the cell. Receptor kinases exist in monomeric forms. When a signal such as insulin binds to each receptor monomer on the extracellular surface of the cell, a conformational change causes the receptor monomers to pair up (or dimerize). This leads to the activation of cytoplasmic domains of the receptor which have the ability to act like kinase proteins. Kinase proteins are able to engage in phosphorylation of specific amino acids. These cytoplasmically situated receptor kinase domains phosphorylate each other at many regions on the receptor tails and can lead to the binding and activation of other important cytoplasmic proteins. Slide 20: As a result of intracellular signal amplification, the extracellular insulin signal causes a series of cytoplasmic proteins to become sequentially activated and will lead to an intracellular response. The intracellular signal ultimately leads to the activation of glucose transporter proteins at the cell surface, and as a result, the absorption of glucose into the cell. Theme 2 Module 4 Script 7 Slide 21: YOUR SUMMARY NOTES: Once insulin has bound to its receptor, the conformational changes and autophosphorylation of the receptor followed by activation of other cytoplasmic proteins induces intracellular signals through the activation of a series of diverse transducer and amplifier proteins that are downstream from the activated receptor. The initiation and maintenance of a signal is regulated by positive-feedback loops to keep the signal and amplification on. However, many elements in a signaling pathway can also activate negative- feedback loops which can lead to intracellular signal termination. An important feature of intracellular signaling also involves double- negative feedback, where an inhibitor of the signal can also be inhibited. This provides fine control in a cell in response to an extracellular signal. Slide 22: Unit 5: mRNA processing through alternative splicing Slide 23: Many tissues that have insulin receptor kinases are able to detect changes in the blood glucose levels and can contribute to the absorption of glucose from the blood. The fat cells in adipose tissue will take up glucose and fatty acids and store the excess as fats in the form of triglycerides. The liver and muscle cells are able to take up glucose from the blood and store the excess as glycogen. However, some tissues are more efficient at absorbing glucose relative to others. For example, skeletal muscles are able to absorb glucose more efficiently than liver cells. How is this achieved? Theme 2 Module 4 Script 8 Slide 24: YOUR SUMMARY NOTES: By regulating RNA processing, eukaryotes, are able to produce more than one mRNA transcript from a single gene. The end result is that this allows for a single gene to encode for more than one protein product, and thus contributes to our proteomic complexity. As we have learned, a pre-mRNA transcript is processed in the nucleus prior to being released as a mature mRNA into the cytosol of the cell. To produce more than one mRNA transcript from a single protein-coding gene, alternative splicing of pre-mRNAs must occur. Slide 25: Recall that exons are the regions of the pre- mRNA that are included in the mature mRNA and that introns are the parts of the pre-mRNA that are removed during the splicing process. During a splicing event, non-protein coding introns are removed from the pre-mRNA, and exons are joined or spliced together to produce a mature mRNA that will then serve as a template for the translation into a functional protein. Alternative splicing enables one pre-mRNA molecule to be spliced at different junctions to result in many different mature mRNA molecules that each contain different combinations of transcribed exons. During alternative splicing, some exons may be excluded during the splicing process (being removed much like the introns), leading to the production of many isoforms or different types of mature mRNA from the same pre-mRNA transcript. This occurs because what the spliceosome will sometimes recognize as an exon in some primary transcripts, can sometimes be identified as an intron in other primary transcripts. These alternative-splicing forms can sometimes be produced in the same cell, or in different cell types. Ultimately, alternative splicing will help in the regulation of gene expression since the same primary transcript can be spliced in different ways to produce mature mRNA isoforms that can then lead to the production of different, but related proteins. Theme 2 Module 4 Script 9 Slide 26: YOUR SUMMARY NOTES: An excellent example of alternative splicing is observed during the processing of the primary transcript of the gene that encodes the human insulin receptor. The insulin receptor gene has 22 exons. In skeletal muscle cells, exon 11 is removed from the mature mRNA product (along with the transcript introns) during the splicing process. This mRNA isoform of the insulin receptor will then be translated into a higher affinity version of the insulin receptor in muscle cells. These skeletal muscle cells will then be able to mount a higher response of glucose uptake in response to an insulin signal. This is an ideal insulin receptor isoform to have at skeletal muscles, since while they contribute to lowering blood glucose levels, they also allow the muscle cells to absorb enough glucose to meet their high energy needs. In contrast, liver cells produce an insulin receptor with lower affinity to insulin. The difference lies in one key difference. During the splicing of the pre-mRNA to mature mRNA in liver cells, exon 11 is retained in the mature mRNA molecule. As a result, we see that while the message in the DNA blueprint is the same, alternative splicing leads to the processing of multiple different mRNA molecules and eventually, the translation of alternate protein isoforms. Slide 27: Our bodies are fine-tuned to maintain steady state blood glucose levels. As we have seen, following a meal, blood glucose levels are elevated, but there is a systemic response that leads to a return to resting blood glucose levels due to the interactions of various cell types and the amplification of signals through cellular signaling cascades. Once the high glucose stimulus is detected by the sensor cells of the pancreas, insulin acts as an effector signal which targets cells of the body to absorb glucose from the bloodstream. This signal to increase glucose absorption into various cell and tissue types is also regulated. Once blood glucose levels are returned to resting levels, there will be feedback to bring the entire system back to the starting or resting point. In this case, we will see a negative feedback loop wherein the drop in blood glucose will be detected by the pancreatic cells and there will be a decrease in the secretion of insulin. As a result, the feedback of this information to the sensor cells limits any further response in the entire system. Theme 2 Module 4 Script 10 Slide 28: The proteome is not nearly as uniform as the YOUR SUMMARY NOTES: genome would predict it to be. While the genome is made up of four nucleotides and these nucleotides are identical in all of our cells, we have structurally and functionally mature proteins that come in different forms (or isoforms). It is the diversity of cellular proteins which contribute to the vast complexity and interactions that underlies various cellular processes. For this reason, any change to alternative splicing mechanisms, or even post-translational modifications of many cellular proteins can lead to detrimental cellular effects. For example, if the insulin protein were not processed correctly following translation, there may not be any ability for this protein to bind to the insulin receptors on target tissues. As we have seen, the insulin receptor is encoded by a single gene, in which alternate splicing results in one of two isoforms. If the insulin receptor isoform was incorrectly spliced during mRNA processing, there also would be no ability to activate glucose transport proteins that allow for the import of glucose from the blood stream at these target regions. A defect in either the insulin protein or the insulin receptor can lead to the inability to take up glucose, resulting in hyperglycemia and eventually diabetes. This is a fine example as to how the complex proteome can vary due to different patterns of gene expression and protein modification, and that these processes contribute to the role that proteins serve as functional molecules of the cell. Slide 29: Checkpoint question #4 Slide 30: In this module we have learned that: the complexity of the human proteome is due to the fact that one gene can code for more than one protein. In addition, we have seen that the ability of a gene to code for more than one protein is brought about by alternative splicing and post-translational modifications Finally, we have learned that this can lead to the production of proteins that are important in regulating the cellular responses that underlie complex systemic regulation