text book [11-30].pdf
Document Details
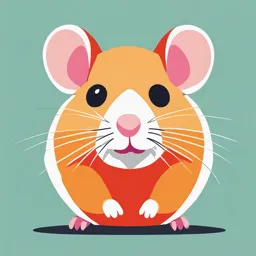
Uploaded by ValiantEmerald
Tags
Full Transcript
1 Gene and Cell Therapy The development of therapeutics to treat human disease has always been a major goal for biomedical research and innovation. Nevertheless, the complexity of the majority of human diseases poses an important and difficult obstacle to overcome. Moreover, the genetic contributi...
1 Gene and Cell Therapy The development of therapeutics to treat human disease has always been a major goal for biomedical research and innovation. Nevertheless, the complexity of the majority of human diseases poses an important and difficult obstacle to overcome. Moreover, the genetic contribution to these conditions complicates the targeting of endogenous and normal processes of cellular functioning, such as transcription and translation. In the past century, the understanding of DNA structure and the development of techniques to manipulate and recombine this molecule allowed the conception of new strategies that could use DNA as a therapeutic agent. In the 1970s, it was proposed for the first time that some human genetic conditions could be treated by the administration of exogenous DNA. The enormous technological advance in the field of biomedicine, with the human genome sequencing or the development of high-throughput techniques, for example, contributed to an effective application of gene therapy in the human context. Recently, the approval of several gene therapy medicines in Europe and the USA definitively established a new paradigm in human disease treatment and opened a new era for gene therapy. article to read : gene therapy for human genetic diseases 1.1 he Concepts of Gene T and Cell Therapy As the name clearly implies, gene therapy refers to the use of genes as “drugs” to treat human diseases. In a simple way, gene therapy could be © Springer Nature Switzerland AG 2020 C. Nóbrega et al., A Handbook of Gene and Cell Therapy, https://doi.org/10.1007/978-3-030-41333-0_1 defined as a set of strategies modifying gene expression or correcting mutant/defective genes, which involves the administration nucleic acids DNA or RNA - to cells. However, more elaborate and complete definitions for gene therapy can be found, especially the ones produced by regulatory agencies. According to the European Medicines Agency (EMA) and to the European Union (EU) directive 2001/83/EC, a gene therapy product consists on a biological medicinal product, which has the following characteristics [1]: (a) it contains an active substance, which includes or consists of a recombinant nucleic acid used in or administered to human beings with a view to regulating, repairing, replacing, adding, or deleting a genetic sequence; (b) its therapeutic, prophylactic, or diagnostic effect relates directly to the recombinant nucleic acid sequence it contains or to the product of the genetic expression of this sequence. Gene therapy medicinal products do not include vaccines against infectious diseases. For the US Food and Drug Administration (FDA), gene therapy is the administration of genetic material to modify or manipulate the expression of a gene product or to alter the biological properties of living cells for therapeutic use [2]. If the idea seems like science fiction, the truth is that in 1972 Friedmann and Roblin discussed this possibility in aScience paper entitled: “Gene therapy for Human Genetic Disease?” [3]. In this very interesting and advanced paper for their time, the authors postulated that gene therapy 1 exampl e: apoptoti c genes like p53 , p21 2 could be used in the future to ameliorate genetic diseases. In a very simple, but very accurate image, they describe how a mammalian cell could be modified by an exogenous source of DNA, following a similar path to that used by a virus (Fig. 1.1). Despite predicting important advantages with this therapy, authors clearly opposed any attempt to perform gene therapy in humans in a foreseeable future. The authors provided three important reasons for that: (i) the understanding on gene regulation and genetic recombination was still inadequate; (ii) the relation between gene and disease phenotype was not clear for many genetic disorders; and (iii) there was no information on gene therapy side effects. Moreover, authors also raised some important ethical concerns about gene therapy, including eugenics, which are still important questions today in gene therapy applications. Less than 20 years later in 1990, the first therapeutic clinical trial using gene therapy started in the USA at the National Institutes of Health (NIH) in Bethesda, Maryland. Michael Blease and French Anderson led the clinical trial in two patients with adenosine deaminase (ADA) deficiency, a monogenic condition causing severe immunodeficiency. In this trial, the two patients, Ashanthi DeSilva and Cindy Kisik, had their autologous T-cells treated ex vivo with the correct ADA gene (using retroviral vectors), which were then reinfused [4]. The success of this trial Fig. 1.1 Strategies for the genetic modification of a cell, as proposed by Friedmann and Roblin in 1972, in their Science paper entitled: “Gene therapy for Human Genetic Disease?”. They posited that delivery of exogenous nucleic acids could be performed using different methods, by which exogenous genes could then be expressed in the modified cells. 1 Gene and Cell Therapy remains controversial as (i) the response of the patients to the treatment was modest and (ii) patients simultaneously received enzyme replacement therapy [5]. Nevertheless, it became the first clinical trial of gene therapy in history and provided an important boost and enthusiasm for the field. However, in 1999 a major drawback led to the partial suspension of gene therapy clinical trials and to the reevaluation of others in the USA. In that year, Jesse Gelsinger died after a severe adverse immune reaction to a adenoviral vector used in gene therapy treatment, only 4 days after the procedure [6]. Gelsinger had ornithine transcarbamylase (OTC) deficiency, which is fatal for most of the carriers, although, in his case, it was partially controlled through drugs and a low-protein diet. Later, it was identified that there were several non-authorized alterations in the clinical protocol and not enough information in the informed consent [7]. Despite this important drawback, several clinical trials using gene therapy continued. One of those trials conducted in Europe treated 10 boys with X-linked severe combined immunodeficiency (X-SCID) [8]. However, after the gene therapy treatment, four boys developed leukemia and one died 60 months after the intervention. Posterior studies showed that the therapeutic transgene was inserted near an oncogene, leading to severe complications starting around 1.1 The Concepts of Gene and Cell Therapy 30 months after the gene therapy. Nevertheless, in a 10-year follow-up of the intervention, the gene therapy was shown to have corrected the disease of the surviving participants [9]. In 2003, due to the adverse events in this study and several other concerns, the FDA suspended gene therapy clinical trials, arguing that not all the safety issues were addressed and more research was needed in the field [10]. Curiously, in the same year, China approved the first gene therapy product, Gendicine®, aiming to treat patients with tumors with p53 gene mutations [11]. However, the therapy was never approved in Europe, the USA, or Japan. The first gene therapy medicine approved in these countries came in 2012, when Glybera® received marketing authorization in Europe for the treatment of lipoprotein lipase (LPL) deficiency, with the cost of 1.1 million euros, being, however, withdrawn from the market in the end of 2017 due to efficacy and low demand issues. Currently, several gene and cell therapy products are approved in Europe and the USA, including Strimvelis® to treat ADA-SCID, and more are in the pipeline for approval in the next years (see what is Sect. 1.11). the differenc The concept and application of gene therapy is closely related to the idea of cell therapy, which e can be roughly defined as an approach where between cell cells are used as therapy or vehicle for therapy. therapyOf course, cell therapy has been used for many and years, considering, for example, blood transfugene sions and bone marrow transplants. Currently therapycell therapy per se has an enormous potential in regenerative medicine, even without genetic modifications of the cells. Nevertheless, the immunologic issues associated with cell transplants opened an opportunity for the combination of cell and gene therapy. In fact, several clinical trials have involved both gene and cell therapies, where defective (or not) cells are isolated from patients, treated with the therapeutic gene (using the appropriate vector), and then reinfused into the patient. Therefore, there is a clear overlap between both strategies Combined, they can be defined as a therapeutic intervention based on the administration of genetic material in order to modify or manipulate the expression of a gene 3 product, altering the biological properties of living cells. Despite all the drawbacks pointed before, research and the improvement in gene therapy knowledge and techniques continued. Important discoveries and advances like the RNA interference (RNAi) pathway, the Human Genome Project, or the production of induced pluripotent stem cells (iPSC) contributed to the continued interest in, and development of, gene therapy. More recently, the advance in gene editing techniques like TALENs or CRISPR provided a new boost in gene therapy, promising better, more accurate, and more effective forms to introduce or modifying genes. For some, gene therapy was a promise that was never fulfilled, while others argue that the better is still to come. What offers no doubt is that gene therapy presents both advantages, like the possibility to effectively eradicate disease, and disadvantages, such as important ethical and safety issues that need to be addressed (Table 1.1). Currently, gene therapy is again in the spotlight of clinical and basic research, utilizing new techniques and taking advantage from the accumulated knowledge, the promising results of preclinical and clinical studies, and the interest of pharmaceutical companies due to the recent approval of several gene therapy products. Of Table 1.1 Advantages and disadvantages of gene and cell therapy Advantages Contributes to disease prevention Contributes to eradicate diseases Helps to reduce the disease risk in future generations Extends life expectancy Avoids constant medication Could replace defective cells Unexplored potential Allows a better understanding of how genes work Disadvantages Modification of human abilities Changes the genetic pool Potential increase in diseases Safety problems High costs Ethical concerns Short-time effect of some strategies Might not be effective against complex diseases 1 4 course, this renovated interest makes gene therapy more prone to unethical procedures or poorly designed studies. Thus, important regulation procedures and careful attention to the studies are needed from the regulatory authorities, but also from scientists all over the world. Designing a gene therapy study (in a preclinical or clinical context) is a complex process, where several variables must be considered ensuring the success and safety of the proposed therapy. Questions regarding the therapeutic target, the delivery system, or the immune response, for example, must be carefully studied and addressed before the application of the gene therapy. In the next sections, we will discuss some of the important issues that should be considered when designing a gene therapy study. 1.2 Types of Gene Therapy The presence of genetic material in almost every cell in the human body makes these cells potential targets for gene therapy, including the germline cells. The major division between somatic and germline cells provides a categorization of gene therapy into two types, depending on the target cells. Somatic gene therapy refers to the interventions targeting the vast majority of human cells (somatic cells). On the other hand, we can speak of germline gene therapy if the targets of the intervention are the reproductive cells (Table 1.2). This very simple but clear classification advises that gene therapy directed to humans should be carried out exclusively in somatic cells. The germline gene therapy raises important ethical questions, being at least for now prohibited in Western countries [12]. Nevertheless, the advent of gene editing reopened the debate, and recently a panel of the US National Academy of Sciences considered the possibility of allowing embryo gene editing to prevent a disease, but only in rare circumstances and after further research [13]. Besides the important ethical and moral questions behind the gene editing of the germline, other technical questions also make it difficult: (i) currently the preimplantation diagnosis is able to identify and prevent several disease mutations, thus limiting the need for genome editing; (ii) the current procedures for zygote editing are not infallible, even in rodents; and (iii) offsite adverse and severe modifications can occur from the gene editing procedure. Despite the ethical and technical arguments, gene editing therapy was recently in the world spotlight, as in 2018 Chinese scientist He Jiankui claims that he performed gene editing in two human embryos, which were implanted and had already been born [14]. The scientific community and the world in general were astonished by this bold but highly questionable move, and the veracity of the claim is not completely assured. For sure, the next few years will bring more debate and controversy on this matter, raising the need for rules and the maintenance of high ethical standards. Scientists and regulatory agencies are in the field, and the organization of world forums and summits on human gene editing will bring new regulation proposals. However, some controversies will certainly arise. 1.3 Table 1.2 Main features of somatic gene therapy compared with germline gene therapy Somatic gene therapy For the majority of the human cells Alterations restricted to the patients Not passed on to future generations Less bioethical concerns Germline gene therapy Changes will be transmitted to next generations Unknown effect on future generations Important bioethical issues Technical difficulties in inserting genes in germ cells Gene and Cell Therapy Gene Therapy Strategies The application of gene therapy seems very straightforward if one thinks of genetic recessive disorders caused by a dysfunctional gene, where one normal copy of the gene could revert the disease phenotype and thus the only material to transfer is the correct gene (Fig. 1.2). This strategy, also called gene augmentation therapy, would be ideal to treat diseases caused by a gene mutation that leads to a malfunctioning or 1.3 Gene Therapy Strategies Fig. 1.2 Gene therapy strategies. In its simplest form, gene therapy could be performed by adding a new functional gene aiming to compensate a mutation or to improve cellular homeostasis – gene augmentation therapy. However, in some cases restoring a certain protein normal function may not be enough to mitigate the disease phenotype. For example, in genetic dominant diseases, mutant gene expression should instead be silenced, thus preventing the formation of the defective protein that causes the disease – gene silencing therapy. Recently, with the 5 advent of gene editing tools, another strategy became available, in which a cell genome could be directly edited by removing a mutation or an entire gene and/or introducing a correct gene – gene editing therapy. All these three gene therapy strategies aim to revert cellular defects caused by malfunctioning genes. However, in certain diseases such as cancer, the aim is to cause cell death. In these cases, gene therapy could also be used, by introducing, for example, a toxic gene that will cause cellular apoptosis – suicide gene therapy. 6 deficiency of the resulting protein. In the treatment, adding a functional/normal version of the defective gene would theoretically guarantee the success of gene therapy. However, from a more practical point of view, this success is conditioned by at least two factors: (i) the levels of the normal protein produced by the inserted gene have to be sufficient and physiological, and (ii) the effects of the disease are still in a reversible state. This type of gene therapy was used in the first gene therapy clinical trial that was already mentioned for ADA-SCID, but it could also be effective for types of severe combined immunodeficiency or for cystic fibrosis (CF), among many others. However, for many diseases, restoring the normal protein function would not be enough to revert the disease phenotype, and the expression of the mutant gene should actually be inhibited. This strategy, also named gene silencing therapy (or gene inhibition therapy) would be suitable, for example, for some genetic dominant diseases, some types of cancer or certain infectious diseases (Fig. 1.2). In the case of dominant diseases, the theoretical setup of this strategy would be to introduce a gene which could inhibit the expression of the mutant gene or that would interfere with the activity of the mutant protein. This approach became very feasible with the discovery of the RNAi pathway in 1998, by Andrew Fire and Craig Mello [15]. RNAi is an endogenous and conserved cellular pathway able to regulate gene expression through small RNA molecules that are complementary to mRNA (for more details, see Chap. 7). For gene therapy, the RNAi pathway offered an opportunity to use endogenous cellular machinery to control the expression of abnormal/defective genes. The gene silencing strategy has already been tested with success for several diseases in preclinical studies and currently is also being tested in clinical trials [16]. With the advent of gene editing techniques like TALENs or CRISPR, another strategy for gene therapy became available, aiming to edit the genome by removing a mutant gene and/or precisely correcting a gene (Fig. 1.2). 1 Gene and Cell Therapy Of course, all strategies have problems and particularities that should be considered. For example, one of the main safety concerns with gene augmentation therapy is the possibility of the random insertion of the transgene, which could occur in problematic genome locations, such as the vicinity of oncogenes, tumor suppressor genes, or unstable genomic regions. On the other hand, the gene silencing or inhibition strategies, despite important successes, fail to completely shut down the expression of the target gene. Moreover, for gene silencing using the RNAi pathway, safety questions like off-target effects, long-term toxicity of the small RNA molecules or RNAi pathway saturation should also to be addressed and considered. Thus, the guided insertion of the transgene or the replacement of the abnormal/defective gene by a normal functioning gene appears as the ideal form of gene therapy, surpassing some of the problems presented by augmentation and silencing strategies of gene therapy. However, only recently have gene editing tools became easier to manipulate, allowing their use in the human gene therapy context. The enthusiasm in this field is so high that in 2016 a Chinese research group injected a person with cells edited by CRISPR-Cas9 [17]. Also in 2016, the first clinical trial using this system received a favorable opinion from an advisory committee at the US National Institutes of Health (NIH), aiming to be used in cancer therapy [18]. Nevertheless, potential off-target effects with these techniques or undesirable editing phenomena should also be considered and studied carefully. The strategies mentioned above aim to restore the cellular homeostasis trying to revert the pathological abnormalities. However, in certain types of diseases such as cancer, the objective is to kill the defective cells. Gene therapy can also be used in this context, by using a transgene that (i) codifies for a highly toxic protein that kills the diseased cells or (ii) expresses a protein that marks the cell as a target for the immune system (Fig. 1.2). This type of gene therapy is sometimes called suicide gene therapy and is discussed in more detail in Chap. 9. 1.4 1.4 Choice of the Therapeutic Target Choice of the Therapeutic Target Another important issue in designing a gene therapy study is the choice of the target gene or cell, which entails a proper understanding of the genetic and molecular causes of a particular condition or disease. In the case of cell therapy, it is easy to conceive that the target will be the sick or defective cells. Nevertheless, it is crucial to consider important questions: (i) Do the cells used as therapeutics need to be treated with gene therapy? (ii) If stem cells are used, what would be the differentiation stage? (iii) What is the source of the cells? Fig. 1.3 Administration routes in gene therapy. An important consideration when designing a gene therapy application is to define the administration route to efficiently deliver the therapeutic gene to the target cells/ organs. If the genes are directly delivered to the organism, 7 In the case of gene therapy, the choice of the target is not so linear, as several options are available and their suitability depends on condition/ disease pathogenesis. As mentioned before, it is easy to understand that, for a monogenic recessive disease, the gene therapy will consist in the addition of a “healthy” copy of the defective gene. However, in more complex pathologies, for example genetic dominant diseases, this strategy is not enough. In these diseases, one possible strategy for gene therapy would be the use of RNA and small RNA molecules to silence the expression of the abnormal causative gene. The different RNAi molecules, like siRNAs, shRNAs, and miRNAs, could be specifically designed to target the mRNA of the causative gene leading to the gene therapy is called in vivo. On the other hand, if the gene is delivered to cells outside the organism and then these manipulated cells are administrated to a subject, then the gene therapy is named ex vivo. 1 8 its cleavage or preventing its translation. Alternatively, a gene could also be used to treat dominant diseases aiming to improve the cellular function (like a gene to activate autophagy) or leading to cellular death (e.g., “suicide” gene therapy). Recently, the addition of a healthy copy of a gene also became an alternative for dominant diseases, if the abnormal copy is removed using gene editing tools. Importantly, the disease pathophysiology should be carefully weighted when choosing a therapeutic target, as for many of the diseases affecting human health the use of cells would not be suitable. 1.5 Administration Routes The localization of the target cells/organs is probably the main factor in deciding the administration route, along with the choice of the gene delivery vehicle, which is normally named vector. Broadly, we can consider two options of administration routes for gene therapy: the direct delivery of the genes to organisms, also named in vivo therapy, and the delivery of genes to cells, which are then transplanted to the organism, named ex vivo therapy (Fig. 1.3). In the in vivo administration, the therapeutic sequence is delivered directly to the target cells, organs, or the whole body, which could be a less invasive method but is more prone to have off-target effects. On the other hand, in ex vivo therapy cells are treated outside the body and then transplanted to the patients, allowing more control of the treated cells, but being technically more complex (Table 1.3). Nevertheless, this rather simple categorization of the administration routes is in fact far more complex. For example, in the direct in vivo administration, important questions should be considered: (i) Are the target cells/organs accessible to a direct application? (ii) In a whole organism administration, which fraction of the therapy reaches the target cells/organs? (iii) Could the remaining fraction be toxic? These and other questions need to be considered when designing Gene and Cell Therapy Table 1.3 Comparison of ex vivo and in vivo administration routes used in gene therapy In vivo (direct delivery) Less invasive Technically more simple Vectors introduced directly Safety check more difficult Reduced control of treated cells Could be applied to a high number of diseases More definitive (depending on the delivery system) Difficult to reach some cells/tissues More off-target effects Ex vivo (cell-based delivery) More invasive Technically more complex No vectors introduced directly Safety check easier More control of treated cells Applied only to a small number of diseases Could be transient (cell lifetime) Possibility of accumulation of mutations Specificity for the treated cells a gene therapy study and before its application. For example, when targeting the central nervous system, the direct delivery route should consider the blood-brain barrier (BBB) and its selectivity. One way to circumvent the BBB would be the intraparenchymal injection into the brain or the infusion into the cerebrospinal fluid (the gene delivery to the central nervous system is discussed in detail in Chap. 4). However, these routes are highly invasive and greatly limit their selection in human patients. The ex vivo administration is also complicated by the source of the cells to be used. If allogenic cells are used, there is the problem of immune compatibility, whereas autologous cells sometimes are defective and are not suitable for the therapy. 1.6 Delivery Systems The delivery of exogenous genetic material into a cell or tissue is not a straightforward or easy process, as organisms developed several strategies and barriers to prevent it (see Chap. 4 for more details). Thus, one of the main issues to consider in a gene therapy strategy is the way to deliver the therapeutic sequence, that is, which delivery system is more suitable to ensure the success of the 1.7 Expression and Persistence of the Therapy therapy. In a broad way, two main groups of delivery systems are currently considered: the viral and the non-viral systems (Fig. 1.4). The viral systems take advantage from the broad diversity of viruses and their innate ability to infect/transduce cells. The key advantage of these systems is their high efficiency, whereas the main drawback is the safety concerns on using modified viruses. On the other hand, non-viral systems include several chemical or physical methods, which have as their principal advantage their safety profile, whereas the main disadvantage is their relatively low efficiency (Table 1.4). The choice of the correct/ideal delivery system for a given gene therapy is dependent on several variables, including the size of the gene, the expected effect, and the toxicity profile, among others. The different delivery systems used in gene and cell therapy are described in more detail in Chaps. 2 and 3. 1.7 Expression and Persistence of the Therapy Another important concern on gene and cell therapy applications is the expression levels of the inserted transgene/sequence, as it is virtually impossible to introduce a single copy of the transgene into the target cells. Importantly, the number of copies introduced is often different among the target cells. Both factors lead to (i) expression differences between target cells and (ii) increased expression levels relative to basal conditions. Moreover, if the transgene is integrated (using, e.g., retroviral vectors), expression will be continuous, producing expression levels that might be different from physiological basal levels (probably much higher), which could lead to toxicity effects. Thus, the implementation of a gene therapy in a clinical setting must ensure very tight and consistent regulation of transgene expression, which could be achieved using regulatable promoters. A proper gene regulation system should display several features, including [19]: (i) a low basal expression of the transgene, (ii) the expression should be triggered by the administration of a molecule and be responsive to 9 a wide range of doses, (iii) be specific to the target cells/organs, (iv) do not interfere with endogenous gene expression, and (v) allow a rapid and effective induction or repression of the transgene expression. Gene regulation systems can be categorized into two main groups: (i) exogenously-regulated systems, which use exogenous compounds to regulate gene expression and which are the most widely used in gene therapy applications, and (ii) endogenously-controlled systems, which rely on internal stimuli to control the transgene expression. Within the first group, the tetracycline (Tet) regulation systems are the most exploited and used tool for controlling gene expression, although others have been developed, like the rapamycin-regulated or the RU486-regulated systems. In the second group of systems, the promoter is sensitive to physiological parameters and conditions, such as glucose levels or hypoxia. However, this endogenous regulation is difficult, and thus most of the systems used are based on the administration of exogenous molecules. Tetracyclines and their derivates like doxycycline (dox) have been widely used in the clinical setting as antibiotics, binding to the bacterial 30S ribosomal subunit and thus inhibiting protein translation. The Tet systems have two variants, the Tet-off system, which was the first one developed and that is based on the negative control by tetracycline [20], and the Tet-on system, that is currently more used and which is based on the positive control of expression by tetracycline [21] (Fig. 1.5). Both systems are based on the bacterial Tet operon, namely, in the Tet repressor protein (TetR) and the tet operator (tetO) DNA elements. In the eukaryotic Tet-off system, the TetR was modified with a transcription activation domain (AD) from the VP16 protein of the herpes simplex virus, creating a tetracycline- controlled transcriptional activator (tTA). Moreover, the tetO sequences were fused with a TATA box-containing eukaryotic promoter to construct the tetracycline-responsive promoter (Ptet). In the absence of tetracycline (or its derivates), the tTA will bind to the tetO sites in the Ptet, thus activating the expression of the downstream transgene. On the other hand, the pres- 10 Fig. 1.4 Overview of the delivery systems used in gene therapy. Organisms and cells have developed several barriers to prevent the entry of exogenous genetic material. Therefore, overcoming these barriers to deliver the therapeutic gene is crucial to the success of gene therapy. In a broad manner, delivery systems for gene therapy can be 1 Gene and Cell Therapy classified into two groups: non-viral vectors and viral vectors. The first group refers to physical and chemical methods, such as microinjection or cationic liposomes. On the other hand, the second group is based on engineered recombinant viruses that are used to deliver the therapeutic transgene. 1.8 Cell Targeting 11 Table 1.4 Main advantages and disadvantages of non- viral and viral systems to used deliver therapeutic genes Advantages Non-viral systems Easy production Low toxicity Unlimited cloning capacity Viral systems High efficiency in gene transfer both in vivo and ex vivo Persistence of therapeutic strategy (in some cases) High variety of cells able to be transduced High variety of viruses to be engineered Natural tropism to infect/transduce cells Natural DNA transport mechanism into nucleus (in some cases) Disadvantages Low efficiency/low expression No transgene integration Low tropism Possible immune/ inflammatory response Safety/toxicity concerns Limited cloning capacity Complex production Limited tropism (in some cases) Possibility of mutagenesis Limited knowledge on molecular mechanisms of infection ence of tetracycline alters the conformation of the TetR domain of tTA, which prevents the binding to tetO sites and blocks the transgene expression (Fig. 1.5, upper panel). From a gene therapy clinical view, the Tet-off system is not very suitable, as long-term administration of tetracycline might be needed in order to repress transgene expression. To overcome this problem, the Tet-on system was developed, allowing the activation of transgene expression in response to the presence of tetracycline. This was achieved by a mutation in the TetR, which allows it to function in a reverse way (rTetR), binding to tetO in the presence of the effector. The system is completed by the fusion of AD to reverse-tTA (rtTA) that binds Ptet and activates the transcription of the downstream transgene in the presence of tetracycline (Fig. 1.5, lower panel). Several improvements on these and other systems were made and are already used in gene therapy clinical trials, namely in cancer [22]. 1.8 Cell Targeting In most human diseases, different cells and organs are not affected, and thus a gene or cell therapy must ensure preferential treatment of the affected cells and organs. Targeting specificity will increase the therapy efficacy, raising the concentration of the therapeutic molecule in the most affected cells/organs and avoiding its sequestration, dilution, or inactivation in non-target cells, at the same contributing for the increase of the safety profile of the therapy. Taking into account the existing experience with conventional drugs, as well as with cellular transplants, several strategies could be employed in gene therapy aiming at specific targeting [23]: (i) physical strategies, where the molecule/cell is delivered locally into the target area; (ii) physiological strategies, based on natural physiological mechanisms of distribution; and (iii) biological strategies, based on biological alterations of the vehicles to achieve a specific localization (Fig. 1.6). Local delivery is probably the most straightforward way of administering a gene therapy; nevertheless, the procedure is extremely invasive, and particular cells/organs may be difficult to access. Taking advantage of physiological mechanisms such as blood circulation is another strategy for gene delivery. Despite the fact that it could be used in some contexts, systemic delivery has to deal with several physiological barriers, for example the BBB, when accessing the central nervous system. The biological strategy implies the modification of the vector/cell, altering its entry proprieties or modulating post-entry features, for example by using a promoter specific to the target cells (Table 1.5) [24]. Depending on the type of vector (viral or non-viral), different modifications could be made in the surface of the vector (mode details on these modifications are detailed in Chaps. 2 and 3). As an example, the lentiviral vector envelope can be modified using glycoproteins from other viruses, thus altering its tropism. In the case of adeno-associated virus (AAV), different serotypes have tropism to different cells, providing a wide 1 12 Gene and Cell Therapy Fig. 1.5 Tetracycline (Tet) regulation system for the control of gene expression. This system is based on the bacterial Tet operon and the administration of tetracyclines or their derivates to control gene expression. The Tet system has two main variants: the Tet-off system (upper panel) and the Tet-on system (lower panel). In the Tet-off system, the TetR is modified with a transcription activation domain from the VP16 protein of the herpes simplex virus, creating a tetracycline-controlled transcriptional activator (tTA). Additionally, the tetO sequences are fused with a TATA box-containing eukaryotic promoter to construct the tetracycline-responsive promoter (Ptet). In the absence of tetracycline (or its derivates), the tTA will bind to the tetO sites in the Ptet, thus activating the expression of the downstream transgene. On the other hand, the presence of tetracycline alters the conformation of the TetR domain of tTA, which prevents the binding to tetO sites and blocks the transgene expression. On the contrary, the Tet-on version allows the activation of transgene expression in the presence of tetracycline. This is achieved by a mutation in the TetR, which allows it to function in a reverse way (rTetR), binding to tetO in the presence of the effector. The system is completed by the fusion of AD to reverse-tTA (rtTA) that binds Ptet and activates the transcription of the downstream transgene in the presence of tetracycline. range of selectable choices. Other viruses like herpes simplex virus have a natural tropism to neurons, which makes them particularly suitable as delivery systems to the nervous system. In the case of non-viral vectors, especially the chemical-based vectors, the use of additional molecules helps to ensure a more directed targeting of particular cells. For example, the use of transferrin allows liposomes to enter the brain, surpassing the BBB. 1.9 Immune Response to the Therapy Circumventing the immune response is a major issue in gene therapy (except if the goal is vaccination or tumor lysis), especially when using viral vectors (see Chap. 4 for more details). The immune system comprehends a complex array of mechanisms protecting the body against 1.9 Immune Response to the Therapy Fig. 1.6 Cell targeting strategies in gene therapy. The delivery of the therapeutic gene to the target cells/organs can be performed using physical strategies, in which there is a local and direct delivery of the gene using devices, such as catheters, or by using physiological strategies, in which endogenous physiological mechanisms, e.g., the blood circulation, are used to deliver the therapeutic gene. Finally, different biological strategies that take advantage of different biological mechanisms can also be used to tar- 13 get cells. For example, the therapeutic gene and vector can have a natural tropism to the target cell or organ, while not targeting other cells. Another strategy involves the use of specific molecules to be recognized by specific target cell receptors, thus specifically delivering the therapeutic gene to these cells. Finally, the use of cell-specific promoters could also limit the expression of the therapeutic gene to the target cells. 1 14 Table 1.5 Different gene promoters and their cell specificity Promoter CMV PGK UbC hAAT TBG Desmin MCK Synapsin CaMKII mGluR2 GFAP MBP Cytomegalovirus Phosphoglycerate kinase Ubiquitin Human α-1-antitrypsin Thyroxine-binding globulin Desmin Muscle creatine kinase Synapsin Ca2+/calmodulin- dependent protein kinase II Metabotropic glutamate receptor 2 Glial fibrillary acidic protein Myelin basic protein Specificity Ubiquitous Liver Skeletal muscle Neurons Astrocytes Oligodendrocytes Gene and Cell Therapy Several strategies were developed to ensure the success of gene therapy by overcoming the immune response [26]. One strategy is to avoid the expression of the delivered gene in antigenpresenting cells (APCs), such as dendritic cells, B-cells, or macrophages. The regulated expression of the transgene could also be used as a strategy to avoid the immune response, by delaying the expression of the gene until the tissue recovers from the inflammation associated with the vector administration. Another strategy is to deliver the genes into immune-privileged sites, like the brain or the eye. Additional strategies also include the modifications of the vector used, for example, performing genetic (viral vectors) or chemical and nonchemical modifications to its structure (both viral and non-viral vectors). Finally, another type of strategy is based on immunosuppression, similar to the procedure following organ transplantation. However, the use of immunosuppression strategies should be carefully planned, as they could interfere with other aspects of the gene therapy, such as modifying the vector internalization, stability, and transduction efficiency or leading to long-term complications such as the increased risk of malignancies. p athogens like viruses and bacteria. The system is divided into two main responses: (i) the innate immune response, which is the initial, rapid and unspecific defense, and (ii) the adaptive immune response, which is stimulated later and more complex than the innate response. The latter response involves the specific, antigen-1.10 Highlights in the History mediated, recognition of the pathogen, its elimiof Gene and Cell Therapy nation through humoral and/or cellular responses, and a memory component that allows improved Many events contributed to gene therapy develresistance to future infections. opment; thus, it is not easy to select the main Both viral and non-viral vectors can induce an highlights, taking also into account that for many immune response, which could lead to the elimi- of these important marks to happen, a lot of studnation of the vector and of the transduced cells, ies and developments were also made. Several of thus decreasing the efficacy of the therapy. the important milestones that we selected for Moreover, the production of proinflammatory these highlights were already referred, including cytokines and chemokines also has a harmful the first gene therapy clinical trial or the first effect on the organism. Diverse factors influence approved gene therapy product in Europe. It is the immune response to a vector, including [25] also important to note that several discoveries (i) the route of administration, (ii) the dose of the and studies were not related directly to gene thervector, (iii) patient-related factors (e.g., age, gen- apy, but contributed decisively for its developder, immune status, drug intake), (iv) the type of ment, taking as an example the discovery of the promoters and/or enhancers used, and (v) the RNAi mechanism. Furthermore, several advances alterations made to the vector genome sequence related to cell therapy were also important for the and/or structure. gene therapy history, for example, the development of iPSC. 1.10 Highlights in the History of Gene and Cell Therapy It is virtually impossible to date the beginning of gene therapy. However, two pioneer papers are probably in the genesis of the idea and possible implications of human gene therapy. In 1967, Marshall Nirenberg formulated the question: Will society be prepared?, recognizing that genetic messages could be synthesized chemically and then be used to program cells [27]. The author recognized the enormous potential of this approach but also the obstacles and ethical problems behind it. Later, in 1972 Friedmann and Roblin asked: Gene therapy for human genetic disease? [3]. If these two papers probably gave the starting shoot on the discussion about gene therapy and its implications, two important scientific advances contributed decisively for the concretization of gene therapy: the first virusmediated gene transfer in 1968 [28] and the creation of the first recombinant DNA molecule in 1972 [29]. As pinpointed throughout this book, the delivery system of the gene is essential to the success of gene therapy and, since the first attempts, a lot of effort was directed to that development. Therefore, another important advance for gene therapy came with the construction of a retrovirus vector. In 1984, Cepko, Roberts, and Mulligan [30] reported the development of a murine retrovirus vector, which allowed the efficient introduction of DNA into mammalian cells. The first approved protocol to introduce an exogenous gene into humans was approved in 1988 and the study published in 1990 [31]. The study was not therapeutic, but rather described the introduction of a bacterial gene into tumor-infiltrating lymphocytes and the tracking of the persistence and localization of the cells after reinfusion into patients with advanced melanomas. Also in 1990, the first gene therapy clinical trial for ADA-SCID took place [4] and launched gene therapy interventions in humans. The initial idea was to perform ex vivo gene therapy using autologous hematopoietic stem cells (HSCs) and retrovirus; however the preliminary studies in nonhuman primates were disappointing, with low levels of viral transduction and engraftment. Instead, the researchers used autologous T-cells 15 treated with the functional ADA gene delivered by a gamma retrovirus vector, which were stimulated to divide in vitro and then the cells were reinfused into the patients. More than the success of the intervention, this clinical trial was a mark for gene therapy, as it proved that it could be applied in humans in a feasible and safe manner. Until now, dozens of ADA-SCID patients have been treated using gene therapy with enormous success, opening the way for the approval of Strimvelis® to treat ADA-SCID, which was the second gene therapy product approved in Europe. In 1998, an important discovery that contributed decisively to the success of gene therapy was published. Scientists Andrew Fire and Craig Mello discovered RNAi [15], a mechanism of gene expression regulation, based on small RNA molecules (siRNAs and microRNAs) complementary to messenger RNAs (mRNAs), which activates a degradation pathway for those mRNAs. But what is the importance of RNAi to gene therapy? In the classical view of gene therapy, a functional or normal copy of a malfunctioning gene is introduced in a patient to treat a disease. Theoretically, in some recessive conditions, this intervention will lead to a complete cure, as one single functional copy may be enough to prevent the disease phenotype. However, for dominant conditions, the introduction of a normal gene is not enough to revert the disease. In this sense, RNAi offered the possibility to treat dominant diseases with gene therapy, by using these small RNA molecules. These molecules can be designed to be complementary to the target mRNA, leading to a reduction of target protein levels and in theory to the mitigation or even cure of the disease phenotype. The use of RNAi or antisense oligonucleotides greatly contributed to the development of gene therapy silencing strategies, aiming to abrogate or reduce the expression of a defective protein causing a disease. In 1999, an important complication that negatively impacted the development of the gene therapy field occurred, with the death of Jesse Gelsinger in a clinical trial for ornithine transcarbamylase (OTC) deficiency. This is a metabolic 16 disease that affects ammonia elimination, being fatal in the first days after birth. However, some patients have a partial OTC deficiency that may be controlled by a strict diet and pharmacological drugs. Jesse Gelsinger had this partial deficiency and was considered an ideal candidate for the gene therapy intervention. He received a dose of 3.8 × 1013 recombinant adenoviral vectors containing the normal OTC gene directly in vivo in the hepatic artery [7]. Jesse died 4 days after the intervention, due to a severe immune reaction to the vector, which induced shock syndrome, cytokine release, acute respiratory distress, and multi- organ failure. It is, however, important to refer that the other 17 subjects that participated in this study, including asymptomatic ones, presented transient mild adverse effects such as muscle aches and fevers. It is also very important to point out that by February 2000 (some months after Jesse death), more than 4000 subjects were already subjected to gene therapy in approximately 400 clinical trials, and Jesse was the only reported death [32]. Nevertheless, at that time, several clinical trials of gene therapy were halted, reviewed, or suspended, and, in the USA, FDA and the National Institutes of Health (NIH) promoted the development of new two programs, trying to enhance the monitoring of gene therapy clinical studies. In 2000, a gene therapy clinical trial conducted in Europe was published in Science, reporting the treatment of 10 boys with a type of immunodeficiency (X-SCID) [8]. The treatment was successful, and 10 years after the trial, the disease was corrected [9]. However, the insertion site of the therapeutic gene led to the development of leukemia in four of the treated boys, and one died because of that. Following this event, FDA suspended gene therapy clinical trials in 2003. Contrastingly, in 2003 China approved the first gene therapy product, Gendicine®, which started to be commercialized in 2004. The product consists of the p53 gene delivered in an adenoviral vector aiming to treat patients with head and neck squamous cell carcinoma [11]. The reported data showed very good therapeutic results and no major adverse side effects, and until 2013 more than 10,000 patients were treated 1 Gene and Cell Therapy with Gendicine® for different types of cancer [33]. Despite all of this, the product was never approved in Europe, the USA, or Japan. The halt in the clinical trials did not stop the development and improvement of gene therapies and strategies. In 2004, EMA granted the first commercial Good Manufacturing Practice (GMP) certification in the EU for the production of commercial supplies of gene-based medicines. The product licensed was Cerepro®, which is an adenoviral vector with the gene thymidine kinase from herpes simplex virus aiming to treat malignant brain tumors. Despite several clinical trials, including a phase III trial, the product did not receive marketing authorization from EMA. In 2004, an important advance for science in general and for gene therapy, in particular, was achieved. In a major international effort, the human genome was fully sequenced, thus completing the Human Genome Project [34] and providing the possibility of precisely locating all human genes. Among other important applications, human genome mapping provided a framework for the development of gene editing techniques for gene therapy. In 2006, another major breakthrough was published by Shinya Yamanaka, which reported the development of induced pluripotent stem cells (iPSC) [35]. These pluripotent stem cells were reprogrammed from adult fibroblasts using four genes, now known as the Yamanaka reprogramming factors: Oct3/4, Sox2, Klf4, and c-Myc. The potential of this advance was soon perceived, namely for regenerative medicine. In the field of gene therapy, it expanded the possibilities of using autologous cells in ex vivo gene therapy applications. In 2014, the first application of iPSC-derived cells in humans started in Japan, for macular degeneration, which is the most prevalent retinal disease in aged people [36]. Despite the reported positive results, the clinical trial was halted 1 year later, due to safety concerns [37]. In the following years, constant research aiming at improving the safety of delivery vectors and at developing better assays for risk assessment led to the return of gene therapy clinical trials for different conditions, such as Leber’s congentinal amaurosis, β-thalassemia, or hemo- 1.12 Ethical Questions and Concerns About Gene and Cell Therapy philia B [38]. The continuous push and effort by many researchers both in preclinical and clinical gene therapy studies led to the approval [39] in 2012 of the first gene therapy product in Europe, Glybera®. The product was based on the delivery of a functional copy of the lipoprotein lipase gene by an AAV vector. However, at the end of 2017, the company producing the product did not renew the marketing authorization, and it was removed from commercialization. More recently, the development of techniques based on nucleases to precisely edit the genome brought a new advance to the gene therapy field, with the possibility of treating the genetic cause of a disease by directly editing or replacing the causative gene(s). These technologies utilize meganucleases, zinc finger nucleases (ZFN), transcription activator-like effector nucleases (TALENs), and the clustered regularly interspaced palindromic repeats (CRISPR) systems. ZFN was the first gene editing system reaching a phase I clinical trial [40]; however, currently most of the studies and research is directed to the CRISPR-Cas system. The very high efficiency and an easy and rapid construction provided an enormous boost in its use compared to the other gene editing plastforms. In 2016, the first clinical trial using the system was approved and launched in China [17]. Gene therapy has come a long way, following a path of successes and drawbacks since the first clinical trial back in 1990. A huge effort by scientists, clinicians, and biotech companies led to the development and continuous improvement of the techniques, systems, and safety of gene therapy applications. The future is of course unknown; nevertheless the recent approval of several gene and cell therapy products in Europe and the USA might indicate that gene therapy is here to stay. 1.11 urrent Status of Gene C Therapy Until November 2017, more than 2600 approved gene therapy clinical trials took place around the world (Fig. 1.7) [41]. Most of these clinical trials were directed to treat cancer, followed by mono- 17 genic, infectious, and cardiovascular diseases (Fig. 1.8). The recent boost in gene therapy and its related safety and technical developments led to the approval of 13 gene therapy products in Europe and/or the USA (Table 1.6). Several others are in the final stages of development and could receive marketing authorization in the near future. 1.12 Ethical Questions and Concerns About Gene and Cell Therapy Considering several issues already mentioned in this book, it is clear that gene therapy is very prone to raise important ethical questions, controversies, and debates. It is evident that genetic manipulation comes with many disadvantages, but also with enormous potential and opportunities (Table 1.7). Thus, gene therapy, as any other medical intervention involving human subjects, has to important requirements and considerations, such as (1) guaranteeing informed consent, considering the ethical principle of respect for persons; (2) having a favorable risk-benefit balance, considering the ethical principle of beneficence/non-maleficence; and (3) having a fair and rigorous selection of the research subjects, considering the ethical principle of justice. However, the complex issues surrounding gene therapy, such as the possibility of altering the personal genetic information, raise specific ethical quandaries: Should germline gene therapy be allowed? How to distinguish between gene enhancement and gene therapy? How to regulate the application of gene therapy? Do we understand the full implications of gene alterations? Is gene therapy only available to people with higher monetary incomes? And so on. A fundamental ethical question in gene therapy concerns the somatic versus germline gene therapy debate. From the accepted point of view, all gene therapies in human subjects should target somatic cells; however, even in this case, enhancement and safety problems should be contemplated. The prohibition on gene therapy targeting the germline cells ensures that its 18 1 Gene and Cell Therapy Fig. 1.7 Gene therapy clinical trials approved by the end of 2017. More than 2500 clinical trials using gene therapy were performed worldwide until 2017. Fig. 1.8 Human conditions addressed in gene therapy clinical trials. Different gene therapy strategies have already been applied to different human conditions, espe- cially to cancer (65% of all the gene therapy clinical studies performed), monogenic diseases (11.1%) and cardiovascular diseases (6.9%). nonethical application is prevented altogether. This issue is now even more relevent, with the development of gene editing techniques allowing the direct and locally specific modification of genes in cells and patients, which fomented novel ethical questions and concerns around gene therapy. Even though the matter of not performing germline gene therapy in humans was a relatively consensual issue among the medical and scientific communities, the recent claim of human embryo editing sounded the alarm and reinforced the regulatory concerns. Although the gene editing procedure was not yet completely verified and certified, the very possibility that is true united the scientific and medical communities against that type of manipulation. Something that undoubtedly emerges from these controversies and debates is the need for a clear regulation on gene therapy application in human subjects, which could be seconded by most of the developed and developing countries having the technology and the means to apply it. Kymriah Luxturna Yescarta Strimvelis Zalmoxis Kynamro Spinraza Exondys 51 Onpattro (patisiran) Tegsedi (inotersen) Zolgensma Zynteglo 1 2 3 4 5 6 7 8 9 10 13 Ex vivo In vivo In vivo In vivo In vivo In vivo In vivo Ex vivo Ex vivo Ex vivo In vivo Ex vivo Administration route In vivo BA-T78-Qglobin SMN1 ASO ASO ASO RNAi ΔLNGFR and HSV-TK Mut 2 ASO CD19-specific CAR T ADA CD19-specific CAR T RPE65 Gene GM-CSF CAR T chimeric antigen receptor T-cell a Caused by mutations in both copies of the RPE65 gene b Per eye c Per infusion d The first infusion and then 375,000 per year for life e Per year f Currently under review by EMA 12 11 Product Imlygic Lentiviral vector AAV9 – – Lipid nanoparticles – – Lentiviral vector Adeno- associated vector Y-Retroviral vector Retroviral vector Retroviral vector Vector HSV-1 β-thalassemia Homozygous familial hypercholesterolemia (HoFH) Spinal muscular atrophy (SMA) Duchenne muscular dystrophy Hereditary transthyretin-mediated amyloidosis Hereditary transthyretin-mediated amyloidosis Spinal muscular atrophy (SMA) Received a stem cell transplant ADA-SCID Non-Hodgkin lymphoma (NHL) Acute lymphoblastic leukemia (ALL) Inherited retinal diseasea Indication Melanoma Table 1.6 Current gene therapies approved for human use in Europe and the USA. Intravenous infusion Cell transplant Intrathecal injection Intravenous Intravenous infusion Subcutaneous Injection Cell transplant Cell transplant Yes Nof Yes Yes No Yes No Yes Yes No No Subretinal injection Cell transplant Yes Approval in Europe Yes Targeting Intralesional injection Cell transplant No Yes Yes Yes Yes Yes Yes No No Yes Yes Yes $2.1 million €1,600,000 $450,000e $750,000d $300,000 $450,000e $176,000 €149,000c €594,000 $373,000 $425,000b $475,000 Approval in the USA Price Yes $65,000 1.12 Ethical Questions and Concerns About Gene and Cell Therapy 19 1 20 Table 1.7 Main advantages and disadvantages of gene manipulation Advantages Less risk of genetic diseases Possibility of preventing disease transmission to next generations Increase in life expectancy Increase in the quality of life Disadvantages Access could be limited to high income individuals Reduction of genetic diversity Unknown interaction between genes Irreversiblte alteration of the human gene pool Gene and Cell Therapy design and implementation. • The story of gene therapy is full of advances and setbacks; however, there are currently several gene therapy products approved in Europe and the USA. • Several ethical problems arise with gene therapy, as it became evident in 2018, with the claim of the first human embryo gene editing procedure with the CRISPR-Cas system. Review Questions However, the huge advance in technical aspects and their availability to almost everyone will certainly constitute an important and difficult challenge for authorities and for the scientific community. This Chapter in a Nutshell • Gene therapy encompasses a set of strategies for modifying gene expression or correcting mutant/defective genes, involving the administration of nucleic acids. • Somatic gene therapy refers to the interventions targeting somatic cells. • Germline gene therapy targets the reprod