FISH Technique PDF
Document Details
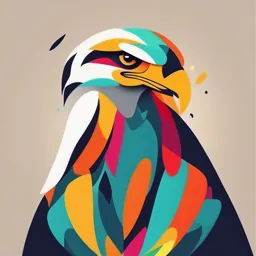
Uploaded by LawfulAccordion328
Tags
Related
- Bio 150 Fluorescence In-Situ Hybridization PDF
- Bio 150: Fluorescence In-Situ Hybridization (FISH) - Midyear 2022-2023 PDF
- Chapter 2: Introduction to Cytology PDF
- GU BMS141 Lecture 1 & 2 Fall 2024 PDF
- UNIT 6 and 7 Molecular Cytogenetics Techniques PDF
- UD4. Cultivo Celular, Técnicas de Diagnóstico (IES Leonardo Da Vinci)
Summary
This document provides an overview of the Fluorescence In Situ Hybridization (FISH) technique. It describes the methodology, various probes used, and applications in medical and biological research. Especially useful for identifying and studying chromosomal aberrations.
Full Transcript
TEXT Introduction Conventional cytogenetic karyotype analysis is still the standard test in genetic diagnostics to identify numerical and structural chromosomal aberrations. Refinements in cytogenetic techniques over the past forty years have allowed the increasingly sensitive detection of chromoso...
TEXT Introduction Conventional cytogenetic karyotype analysis is still the standard test in genetic diagnostics to identify numerical and structural chromosomal aberrations. Refinements in cytogenetic techniques over the past forty years have allowed the increasingly sensitive detection of chromosome abnormalities. In 1969, Pardue and Gall first described the in situ hybridization (ISH) of radioactively labeled repetitive DNA probes on cytological preparations. In 1986, Pinkel and Cremer, reported fluorescence in situ hybridization (FISH) using non-radioactively labeled fluorescence. FISH is a cytogenetic technique developed by biomedical researchers, used to detect and localize the presence or absence of specific DNA sequences on chromosomes. FISH uses fluorescent probes that bind to only those parts of the chromosome with which they show a high degree of sequence homology. Fluorescence microscopy can be used to find out where the fluorescent probe is bound to the chromosomes. The high sensitivity and specificity of the technique and the speed with which the assays can be performed have made FISH a powerful tool with numerous applications and it has gained general acceptance as a clinical laboratory tool. FISH is often used for finding specific features in DNA for use in genetic counseling, medicine and species identification. It supplements conventional karyotyping by providing additional information in certain cases (Figure.1). FISH to localize genes on chromosomes FISH can localize a gene to a specific place on a chromosome. First, metaphase chromosomes are isolated and attached to a microscope slide. The chromosomal DNA is denatured into single-stranded pieces that remain attached to the slide. The fluorescent probe hybridizes to the corresponding gene. When the slide is illuminated, the hybrid molecules fluoresce and reveal the location of the gene of interest. Principle/ Important steps of FISH technique: Cells in metaphase or interphase are fixed on a slide and denatured to change the double stranded DNA into single-stranded DNA. Hybridization: Nucleic acid hybridization is the formation of a duplex between two polynucleotide chains which have complementary sequences. DNA-DNA DNA-RNA RNA-RNA Figure.2: Diagrammatic presentation of hybridization The metaphase or interphase preparation is then hybridized with DNA sequences that are complementary probe to the region of interest and that have been labeled with biotin (Figure.2). The hybridization site is made visible by means of a primary antibody against biotin; this antibody is bound to a fluorochrome, e. g., fluorescein isothiocyanate (FITC) (Figure.3). Since the primary signal is quite weak, a secondary antibody (e. g., avidin) bound to biotin is attached. A further primary antibody can then be attached to the secondary antibody (Figure.3). This amplifies the signal, which can then be demonstrated by bright fluorescence. Visualization of the Probe: Microscopy and Digital Image Analysis DNA probe is labeled with a colored fluorescent molecule. This fluorescent molecule remains attached to the DNA during the hybridization process. The molecule emits a particular color when viewed through a fluorescence microscope that is equipped with the appropriate filter sets, CCD Camera [Digital camera systems, incorporating a variety of Charge Couple Device sensor (Photo Diode), are by far the most common image capture technology employed in modern optical microscopy] (Figure.4). Figure.3: Principle of FISH Figure.4: Observation of slide using fluorescence microscope equipped with appropriate filter sets and CCD Camera Probes so far used for FISH: Probe is a nucleic acid that can be labeled with a marker which allows identification and quantitation. Probes are the complementary sequences of target nucleic acids, designed against the sequence of interest. Probes are tagged with fluorescent dyes like fluorescein, biotin, digoxigenin (Figure.5). The differences between the various FISH techniques are usually due to variations in the sequence and labeling of the probes; and how they are used in combination. Probe size ranges from 20 bp to 1000 bp. Probe size is important because longer probes hybridize less specifically than shorter probes, a short strand of DNA or RNA which is complementary to a given target sequence, it can be used to identify or locate the target. Quality of FISH probe Radioactive (32P, 35S, 14C, 3H) Fluorescent Biotinylated (avidin-streptavidin) Direct and indirect labelling: Direct labelling: FISH probes are labeled directly using fluorochrome-conjugated nucleotides (e.g. fluorescein-dUTP). Label is bound to the probe. Less sensitive (Figure.6). Indirect labelling: Require an additional step before detection. Indirectly using reporter molecules (e.g. biotin-dUTP) by nick-translation, random priming, PCR, or various other molecular genetic techniques. Probe detected using antibodies conjugated to labels like Alkaline phosphatase. Results in amplification of signal Fluorescent signals of bound probes are inspected using filter-equipped epifluorescence microscope and computer software. Figure.6: Types of probes/ Choice of probes/ Specificity of probes: A large number of different probes designed to identify specific chromosomes and parts thereof are available for diagnostic purposes. The choice of probe and the simultaneous use of multi-probe essays depend on the particular application in question. One of the most important considerations in FISH analysis is the choice of probe. A wide range of probes can be used, from whole genomes to small cloned probes. There are broadly four types of probe, each with a different range of applications (Figure.11): 1. Whole-Chromosome Painting Probe (WCP)/ Partial Chromosome Probe (PCP) Routine application of the technique on human chromosomes started in 1996 with the simultaneous use of all 24 human whole chromosome painting probes in multiplex-FISH (M-FISH) and Spectral Karyotyping (SKY). Since then different approaches for chromosomal differentiation based on multicolor-FISH (mFISH) assays have been described. Different names have been introduced for more or less the same probe sets: Multiplex-FISH (M-FISH) (Speicher et al., 1996) Spectral Karyotyping (SKY) Schröck et al., 1996); Multicolor FISH (mfish) (Senger et al., 1998; Tanke et al., 1998); COBRA-FISH (= Combined Binary RAtio labelling-FISH) (Tanke et al., 1999); or 24-color-FISH (Azofeifa et al., 2000). M-FISH and SKY allow painting of the entire chromosome complement in a single hybridization through labelling each chromosome with a different combination of fluorophores. Images are collected with a fluorescence microscope that has filter sets for each fluorochrome and a combinatorial labelling algorithm allows separation and identification of all chromosomes, which are visualized in characteristic pseudo-colours. M-FISH and SKY differ only in the method used to discriminate the differentially labelled probes. SKY uses a dedicated imaging system that incorporates a cooled charge couple device (CCD camera). M-FISH uses specific narrow fluorescence filter sets to reduce crosstalk and digital imaging equipment as part of a conventional epifluorescence microscope, with appropriate computer software. The high efficiency of modern epifluorescence microscopes reduce typical exposure times limiting the effects of photobleaching. The main applications for M-FISH have been chromosomal rearrangements and marker chromosomes in solid tumours, which are often distinguished by complex karyotypes. In common with other whole chromosome painting methods, both M-FISH and SKY are not suitable for discriminating intrachromosomal rearrangements such as duplications, deletions or inversions. Chromosome painting probes [whole chromosome paint (wcp) and partial chromosome paint (pcp)] obtained by flow sorting or micromanipulator-mediated chromosome microdissection, allow the labeling of individual chromosomes in metaphase spreads and the identification of both numerical and interchromosomal structural rearrangements (Figure.7). Figure.7: Showing WCP by FISH 2. Repetitive sequence probes/ telomeric probes: Specific for telomeres. Hybridize to these specific chromosomal regions or structures that contain short sequences which are present in many thousands of copies. Have specificity for a single human chromosome arm. They contain a locus estimated to be within 300 kb of the end of the chromosome. Examples of this probe type are Pan- telomeric probes targeting the tandemly repeated (TTAGGG) sequences present on all human chromosome ends. In most instances, these sequences are distinct, such that a- satellite probe derived from one chromosome will hybridize to that chromosome only (Figure.8). Figure.8: Showing distribution of telomeric probes 3. Locus-specific probes/ Locus Specific Identifiers (LSI): Interphase FISH can be used for the detection of any chromosome abnormality for which there is an appropriate probe. Probes of this class are particularly useful for detecting structural rearrangements such as specific chromosomal abnormalities: Deletion: Deletion probes Translocation: Translocation probes Gene detection and localization probe Gene amplification probes Usually genomic clones, which vary in size depending on the nature of the cloning vector, from Plasmids (1–10 kb) to the larger PAC [The PAC (P1-derived artificial chromosome) are DNA constructs that are derived from the DNA of P1 bacteriophage. They can carry large amounts (100-300 kb) of other sequences for a variety of bioengineering purposes)], YAC [YAC (Yeast artificial chromosomes) are genetically engineered chromosomes derived from the DNA of the yeast, Saccharomyces cerevisiae, which is then ligated into a bacterial plasmid. By inserting large fragments of DNA, (100–1000 kb), the inserted sequences can be cloned and physically mapped using a process called chromosome walking)] and BAC [BAC (bacterial artificial chromosome) is a DNA construct, based on a functional fertility plasmid (or F-plasmid), used for transforming and cloning in bacteria (usually E. coli.) vectors (80 kb to 1 Mb)]. Example: A locus-specific probe for the BCR/ bcl (breakpoint cluster region) gene at 22q11.2 detected with a green flurochrome. A locusspecific probe for the Abl (leukemia virus which carries a similar protein: Abelson oncogene) gene t9q34 detected with a red fluorochrome will appear as a bright yellow spot (the combination of green and red fluorochromes) in leukaemia cells when viewed via fluorescence microscopy, characterized by the Bcl/ Abl fusion gene, resulting from t(9;22)(q34.1; q11.2). [This is a reciprocal translocation, creating an elongated chromosome 9 (termed a derivative chromosome, or der 9), and a truncated chromosome 22 (the Philadelphia chromosome). In agreement with the International System for Human Cytogenetic Nomenclature (ISCN), this chromosomal translocation is designated as t (9; 22)(q34;q11) (Figure.9A & B). Figure.9A: FISH image of BCR/Abl abnormalities Figure.9B: Diagrammatic presentation of BCR/ Abl reciprocal translocation 4. Centromeric probes/ Chromosome Enumerator Probes (CEP) For chromosome enumeration, probes detecting chromosome-specific α-satellite regions near the centromeres are preferred [centromeric probe (cep)] (Figure.10). Generated from repetitive sequences found in centromeres. Most are Alpha and Satellite III Probes. Centromere regions stained brighter - means they are rich in A-T bonds. Centromeric probes, which target all human centromeres, are also available. Satellite DNA probes hybridize to multiple copies of the repeat unit present at the centromeres resulting in a two very bright fluorescent signals in both metaphase and interphase diploid cells, hence making centromere-specific probes particularly suitable for the detection of monosomy, trisomy and other aneuploidies in both leukaemias and solid tumours. A centromere-specific probe for chromosome 8 has been used to detect trisomy by FISH in newly diagnosed patients with acute myeloid leukaemia (AML). Figure.10: Chromosome morphology using CEP Figure.11: Major types of Fish probes Molecular beacons as a fluorescent probe: A molecular beacon is a fluorescent probe molecule that is designed to fluoresce only when it binds to a specific DNA target sequence. This probe has two engineered regions at the ends of the probe sequence (Figure.12): On the 5¢ side, a fluorescent tag is added (F), and On the 3¢ side, a quenching group is added (Q) DNA sequence of 20 to 30 bases. The central region of the probe is complementary to the target sequence. The terminal half dozen bases at each end of the probe are complementary and form a short double- stranded region as shown in Figure. In the stem and loop conformation the quenching group is next to the fluorophore and so prevents fluorescence. Just inside the two tags are six base pairs that can form a stem-loop structure. In this state the probe cannot fluoresce. When the probe binds to the target sequence, the stem-loop structure is lost. Since the quenching group is no longer next to the fluorescent tag, the probe can now fluoresce. When the molecular beacon binds to the target sequence it is linearized. This separates the quenching group from the fluorophore, which is now free to fluoresce. Figure.12: Molecular Beacon Technical improvement and recent development of FISH technique: 1. Centromere-specific Multi Color FISH Recently, an all-human centromere-specific multicolour-FISH approach (cenM-FISH) for the ascertainment of the origin of structurally abnormal, cytogenetically unidentifiable chromosomes – so-called marker chromosomes. This technique allows the simultaneous characterization of all human centromeres using differently labeled centromeric satellite DNA as probes. 2. Comparative genomic hybridization (CGH): One of the most significant developments for FISH in relation to genome-wide screening was the introduction of comparative genomic hybridization (CGH). This modification of quantitative two colour fluorescence in situ hybridization utilizes genomic DNA from the sample under test to generate a map of DNA copy number changes in tumour genomes making it an ideal tool for analyzing chromosomal imbalances in tumour material and for examining possible correlations between findings and tumour phenotypes. In CGH, the genomic DNA from the specimen and control DNA extracted from an individual with a normal karyotype (46,XX or 46,XY) are differentially labelled with green and red fluorochromes respectively, mixed in equal amounts and co-hybridized to reference human metaphase chromosomes. The relative difference in DNA content between the normal and specimen DNA is represented by a difference in the green: red fluorescence ratios. 3. Virtual Karyotype Virtual karyotyping is another cost-effective, clinically available alternative to FISH panels using thousands to millions of probes on a single array to detect copy number changes, genome-wide, at unprecedented resolution. Currently, this type of analysis will only detect gains and losses of chromosomal material and will not detect balanced rearrangements, such as translocations and inversions which are hallmark aberrations seen in many types of leukemia and lymphoma. FISH to detect and measure mRNA levels A variety of different mRNA species are expressed at any one time within a tissue. Not all cells express the same genes or express them at the same level. If the target cells are probed with fluorescently labeled DNA (red), this will bind to the corresponding mRNA (blue). Notice that the probe DNA does not bind to the nuclear DNA because in this procedure the cells were not treated to denature the chromosomal DNA. The target gene in this example was only expressed in two of the cells (Figure.13). Protocol for FISH A. Reagents and Equipment necessary for FISH 1. 20x Saline-sodium citrate buffer (SSC: 3 M NaCl, 0.3 M sodium citrate, pH 7) 2. RNase A 100 µg/ml in 2x SSC. 3. Pepsin 40 units/ml in 10 mM HCl. 4. Paraformaldehyde, EM grade freshly depolymerized, 4% w/v in water. 5. Ethanol. 6. Labeled probe. Plasmid DNA is labeled with biotin-11-dUTP using nick translation random priming or the polymerase chain reaction 7. Hybridization mix solution: 50%, 10% dextran sulfate 0.1% SDS, 0.5-1.5 ng/µl labeled probe and 300 ng/ml Salmon Sperm DNA in 2x SSC. 8. Wash buffer: 20% formamide in 0.1x SSC. 9. Detection buffer: 0.2% Tween 20 in 4x SSC. 10. Blocking buffer: 5% bovine albumin in detection buffer. 11. Antibody or detection compound (e.g., Streptavidin-Cy3 in blocking buffer. 12. DAPI 2 µg/ml in antifade mounting medium. 13. Fluorescence microscope, filters and optional triple band pass filter (x58, Omega Optics). 14. Glass slides. 15. Plastic cover slips for incubation and hybridization steps. 16. Heat block/ modified thermocycler. 17. Coplin jars for washing steps). B. Slide Preparation 1. Start with chromosome preparations from any cell type. 2. Incubate with 200 µl RNase for 1 hour at 37 °C 3. Wash slides in 2x SSC for 5 minutes, repeat. 4. Rinse slides in 10 mM HCl. 5. Incubate with 200 µl pepsin for 10 minutes at 37 °C. 6. Rinse slides in deionized H2O. 7. Wash slides in 2x SSC for 5 minutes, repeat. 8. Stabilize slides in paraformaldehyde for 10 minutes. 9. Wash slides in 2x SSC for 5 minutes, repeat. 10. Dehydrate slides in an ethanol series: 70%, 80%, 95%; 2 minutes each. 11. Air dry. C. Hybridization 1. Prepare 30 µl hybridization solution per slide. Heat to 70 °C. for 10 minutes and place on ice. 2. Place 30 µl of hybridization solution on each slide and cover with a plastic cover slip. 3. Denature slide at 65-70 °C for 5 minutes on heat block. 4. Gradually decrease temperature to 37 °C. 5. Hybridize at 37 °C overnight in humidity chamber. D. Detection of cytogenetic abnormalities under fluorescence microscope: 1. Wash slides in 2x SSC to remove coverslip. 2. Wash slides in wash buffer at 40 °C for 5 minutes, repeat. 3. Wash slides in 0.1x SSC at 40 °C for 5-15 minutes. 4. Wash slides in 2x SSC at 40 °C for 5-15 minutes. 5. Cool slides to room temperature. 6. Equilibrate slides in detection buffer for 5 minutes. 7. Block in blocking buffer for 20-30 minutes. 8. Incubate with 50 µl antibody or detection compound for 30-60 minutes (e.g., 5 µg/ml Streptavidin-Cy3 in blocking buffer). 9. Wash slides in 2x SSC for 5 minutes, repeat twice. 10. Counter stain with DAPI solution for 10 minutes. 11. Rinse briefly and mount in antifade mounting medium. 12. Analyze with fluorescence microscope. Applications of FISH The high sensitivity and specificity of FISH and the speed with which the assays can be performed have made FISH a pivotal cytogenetic technique that has provided significant advances in both the research and diagnosis. FISH has greatly expanded the capabilities of cytogenetics and pathology laboratories through its high sensitivity, specificity and rapid turnover with a high efficiency of hybridization and detection. The broad applications of FISH techniques are: 1. Diagnosis of genetic diseases: A. From a medical perspective, FISH can be applied to detect genetic abnormalities such as aneuploidy, loss of a chromosomal region or a whole chromosome or to monitor the progression of an aberration serving as a technique that can help in both the diagnosis of a genetic disease or suggesting prognostic outcomes. Often parents of children with a developmental disability want to know more about their child's conditions before choosing to have another child. These concerns can be addressed by analysis of the parents' and child's DNA. In cases where the child's developmental disability is not understood, the cause of it can potentially be determined using FISH and cytogenetic techniques. Examples of diseases that are diagnosed using FISH include: Cri-du-chat/ cat's cry syndrome: Also known as 5p - (5p minus) syndrome, is a chromosomal condition that results when a piece of chromosome 5 is missing. Infants with this condition often have a high-pitched cry that sounds like that of a cat. Down syndrome: Down syndrome result from trisomy 21, which means each cell in the body has three copies of chromosome 21 instead of the usual two copies. Velocardiofacial syndrome: Velocardiofacial Syndrome, also known as VCFS or as 22q11.2 Syndrome or as DiGeorge Syndrome, is the most common syndrome associated with cleft palate. Velocardiofacial Syndrome is an autosomal dominant condition. Genetic studies of children with this condition show that a microscopic segment on the long arm of chromosome 22 is missing. Prader-Willi syndrome: Prader-Willi syndrome is a rare genetic disorder in which seven genes (or some subset thereof) on chromosome 15 (q 11– 13) are deleted or unexpressed (chromosome 15q partial deletion) on the paternal chromosome. Angelman syndrome: Angelman syndrome is a genetic disorder, most often caused by problems with a gene located on chromosome 15 called the ubiquitin protein ligase E3A (UBE3A) gene. Normally, only the maternal copy of the UBE3A gene is active in the brain. Most cases of Angelman syndrome occur when part of the maternal copy is missing or damaged. In a few cases, Angelman syndrome is caused when two paternal copies of the gene are inherited, instead of one from each parent. Phelan-McDermid syndrome/ 22q13 deletion syndrome: It is a disorder caused by the loss of a small piece of chromosome 22. The deletion occurs near the end of the chromosome at a location designated q13.3. Microdeletion syndromes: Microdeletion syndrome is a syndrome caused by a chromosomal deletion (< 5Mb) spanning several genes that is too small to be detected by conventional cytogenetic methods or high resolution karyotyping (2-5 Mb). Monitoring the success of bone marrow transplantation Prenatal diagnosis B. FISH can also be applied to such research applications as gene mapping or the identification of novel oncogenes or genetic aberrations that contribute towards various cancers. Material for FISH can be processed in 4–24 h, and the analysis of 1000–2000 cells accomplished in 15–45 min, enabling the information on the cytogenetic pattern of tumour cells to be achieved within a sufficient time frame for use in treatment strategies. C. FISH on sperm cells is indicated for men with an abnormal somatic or meiotic karyotype as well as those with oligozoospermia, since approximately 50% of oligozoospermic men have an increased rate of sperm chromosome abnormalities. The analysis of chromosomes 21, X, and Y is enough to identify oligozoospermic individuals at risk. D. A traditional examination involving metaphase chromosome analysis is often unable to identify features that distinguish one disease from another, due to subtle chromosomal features; FISH can elucidate these differences. E. FISH can also be used to detect diseased cells more easily than standard Cytogenetic methods, which require dividing cells and requires labor and time- intensive manual preparation and analysis of the slides by a technologist. FISH, on the other hand, does not require living cells and can be quantified automatically, a computer counts the fluorescent dots present. However, a trained technologist is required to distinguish subtle differences in banding patterns on bent and twisted metaphase chromosomes. F. FISH can be incorporated into Lab-on-a-chip microfluidic device. This technology is still in a developmental stage but, like other lab on chip methods, it may lead to more portable diagnostic techniques. 2. Biological species identification A. FISH is often used in clinical studies. If a patient is infected with a suspected pathogen, bacteria, from the patient's tissues or fluids, are typically grown on agar to determine the identity of the pathogen. Many bacteria, however, even well-known species, do not grow well under laboratory conditions. FISH can be used to detect directly the presence of the suspect on small samples of patient's tissue. B. FISH can also be used to compare the genomes of two biological species, to deduce evolutionary relationships. A similar hybridization technique is called a zoo blot. C. FISH is widely used in the field of microbial ecology, to identify microorganisms. Biofilms, for example, are composed of complex (often) multi-species bacterial organizations. Preparing DNA probes for one species and performing FISH with this probe allows one to visualize the distribution of this specific species within the biofilm. Preparing probes (in two different colors) for two species allows to visualize/ study co-localization of these two species in the biofilm, and can be useful in determining the fine architecture of the biofilm. Different approaches for the future development of FISH technique Recent advances, such as improved hybridization protocols and strategies, novel commercial and non-commercial probe sets, alongside the efforts of the Human Genome Project, coupled with hardware development such as image analysis software and specific filter sets for newly established fluorochromes, facilitate the collection of new important data for the understanding of genetic diseases. Disadvantages of FISH Technique [Negative aspects]: FISH and to a lesser extent SKY and M-FISH can only detect known genetic aberrations, providing the specific probe is available (in other words a probe for a known genetic aberration has to be hybridized to the specimen in order for the FISH technique to indicate the presence or absence of that specific genetic aberration alone). FISH analysis with locus specific probes or chromosome-specific DNA libraries is restricted to the targeted chromosome or chromosomal sub region. Therefore, and in strong contrast to chromosome banding-based karyotype analysis, while most valuable in the confirmation of previously characterized chromosomal aberrations, FISH cannot serve as a screening test for chromosomal rearrangements since most FISH techniques can only detect known imbalances. SKY (and M-FISH) can detect multiple karyotype abnormalities simultaneously, but both techniques are dependent on combined fluorochrome probes. M-FISH and SKY are not useful for distinguishing intrachromosomal rearrangements such as duplications, deletions or inversions. Concluding remarks: Despite the minor disadvantages discussed in the previous section, the advent of FISH in cytogenetics in has proved invaluable in both diagnostics and research. The power of its ability to identify specific genetic aberrations has propelled FISH-based techniques to the forefront of screening procedures for prenatal, paediatric and adult cases in a wide variety of cell types, including paraffin-embedded tissue, making FISH analysis data a useful tool in the decision of therapy to combat cancer. The ultimate goal of FISH utilization would be an arraybased screen using the complete oncogenic repertoire to diagnose any prenatal or postnatal aberration(s). Improved aetiology through techniques such as FISH may be crucial in the fight against cancer, with the knowledge acquired effectively directed towards the research and development of better treatment strategies to benefit the sufferers of diseases based on these genetic aberrations.