Test 3 Study Guide PDF
Document Details
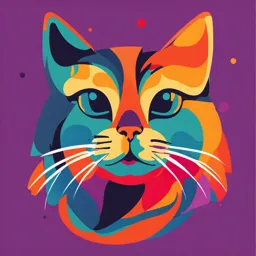
Uploaded by FieryAntigorite2448
Rocky Mountain College
Tags
Summary
This document contains a study guide on various topics in biology, including photosynthesis and genetic inheritance. It covers alternative photosynthetic pathways and the role of probability in inheritance, providing insights into how traits are inherited and how variations arise in offspring.
Full Transcript
1. Alternative types of photosynthesis- typical of plants in hot, dry environments. Why does a plant have a difficult time photosynthesizing in a hot, dry environment? ==================================================================================================================================...
1. Alternative types of photosynthesis- typical of plants in hot, dry environments. Why does a plant have a difficult time photosynthesizing in a hot, dry environment? ========================================================================================================================================================================= Answer: Plants in hot, dry environments face challenges with photosynthesis primarily due to water scarcity and high temperatures. In these conditions, plants often close their stomata, which are tiny openings on the leaves that allow gas exchange. This closure helps to reduce water loss but also limits the intake of carbon dioxide, which is essential for photosynthesis. Without sufficient carbon dioxide, the process of converting light energy into chemical energy is hindered, leading to reduced glucose production. Additionally, high temperatures can lead to increased rates of photorespiration, a process that competes with photosynthesis and can further decrease the efficiency of carbon fixation. To adapt to these challenging conditions, some plants utilize alternative photosynthetic pathways, such as C4 and CAM (Crassulacean Acid Metabolism) photosynthesis. C4 plants, like maize and sugarcane, have a specialized mechanism that allows them to capture carbon dioxide more efficiently even when stomata are partially closed. CAM plants, such as succulents, take in carbon dioxide at night when temperatures are cooler, storing it for use during the day. In summary, the difficulties in photosynthesizing in hot, dry environments arise from water loss, limited carbon dioxide intake, and increased photorespiration, leading to the evolution of alternative photosynthetic pathways in certain plant species. 2\. C4 photosynthesis, CAM photosynthesis,... How do these alternative pathways improve efficiency? Answer: C4 and CAM photosynthesis are alternative pathways that improve efficiency in plants adapted to hot, dry environments in distinct ways. C4 photosynthesis enhances efficiency by minimizing photorespiration. In C4 plants, carbon dioxide is initially fixed into a four-carbon compound in specialized cells called mesophyll cells. This compound is then transported to bundle sheath cells, where it releases carbon dioxide for the Calvin cycle. This separation of initial carbon fixation and the Calvin cycle allows C4 plants to maintain higher concentrations of carbon dioxide, even when stomata are partially closed, reducing water loss while still effectively synthesizing sugars. On the other hand, CAM photosynthesis takes a different approach. CAM plants open their stomata at night to take in carbon dioxide, which is then stored as organic acids. During the day, when the stomata are closed to conserve water, the stored carbon dioxide is released for use in the Calvin cycle. This adaptation allows CAM plants to photosynthesize during the day while minimizing water loss, making them highly efficient in arid conditions. Both pathways allow plants to thrive in environments where traditional photosynthesis would be less effective due to water scarcity and high temperatures. 3.How did Mendel's hypothesis provide answers for the following questions? What exactly is inherited? How is it inherited? What is the role of probability in inheritance? Why do desired traits "disappear" in one generation and "re-appear" in the next? Why do some but not all offspring show the desired trait? (continuity & variation) Answer: Mendel\'s hypothesis laid the groundwork for understanding inheritance through his experiments with pea plants. Here's how his work addressed your questions: A. What exactly is inherited? How is it inherited? Mendel proposed that traits are inherited as discrete units, which he called \"factors\" (now known as genes). He discovered that each trait is determined by alleles, which can be dominant or recessive. The inheritance of these alleles follows specific patterns, where offspring receive one allele from each parent. B. What is the role of probability in inheritance? Mendel introduced the concept of probability in inheritance through his laws of segregation and independent assortment. He showed that the likelihood of inheriting a particular trait can be predicted using probability. For example, in a monohybrid cross, the ratio of dominant to recessive traits can be expressed as a probability, allowing predictions about the traits of future generations. C. Why do desired traits "disappear" in one generation and "re-appear" in the next? Mendel\'s work explained that recessive traits may not be expressed in every generation. When a dominant allele is present, it masks the effect of the recessive allele. However, when two heterozygous individuals are crossed, the recessive trait can reappear in future generations if both parents pass on the recessive allele. D. Why do some but not all offspring show the desired trait? (continuity & variation) The variation in traits among offspring arises from the combination of alleles inherited from both parents. Some offspring may inherit two dominant alleles, some may inherit one of each, and others may inherit two recessive alleles. This genetic variation leads to different expressions of traits, explaining why not all offspring display the desired trait. In summary, Mendel\'s hypotheses provided a framework for understanding the inheritance of traits, the influence of probability, and the patterns of trait expression across generations. 4\. What were the key points of Mendel's experimental system? Sample size, generation time, controlled fertilization,... Answer: Mendel\'s experimental system had several key points that contributed to his groundbreaking discoveries in genetics: -Sample Size: Mendel used a large number of pea plants in his experiments, which allowed for a more reliable analysis of the inheritance patterns. By studying thousands of plants, he could identify consistent ratios in trait inheritance. \- Generation Time: Pea plants have a relatively short generation time, enabling Mendel to observe multiple generations in a relatively short period. This allowed him to track the inheritance of traits across generations more effectively. \- Controlled Fertilization: Mendel carefully controlled the fertilization process by using techniques such as cross-pollination and self-pollination. He ensured that he knew the parentage of each plant, which was crucial for understanding how traits were passed down. \- Choice of Traits: Mendel selected specific traits that were easy to observe and had clear dominant and recessive forms, such as flower color and seed shape. This made it easier to analyze the inheritance patterns. \- Mathematical Analysis: Mendel employed statistical methods to analyze the results of his crosses, leading to the formulation of his laws of inheritance. He was one of the first to apply mathematical principles to biological studies. These key points in Mendel\'s experimental system were fundamental in establishing the principles of heredity and laid the groundwork for modern genetics. 5\. Describe one of Mendel's typical 3-generation experiments Parental traits, F1 and F2 traits, proportions of different traits How does Mendel's model explain the continuity and variation in inherited traits? Laws of Segregation, carriers, genotype, phenotype, recessive, dominant,.. Answer: One of Mendel\'s typical 3-generation experiments involved crossing pea plants with contrasting traits, such as tall and short plants. Here\'s a breakdown of the process: \- Parental Generation (P): Mendel started with true-breeding plants, one tall (dominant trait) and one short (recessive trait). The parental traits were clearly defined, and he ensured that these plants were pure for their respective traits. \- First Filial Generation (F1): When Mendel cross-pollinated the tall and short plants, all the offspring in the F1 generation were tall. This demonstrated that the tall trait was dominant over the short trait. The genotype of the F1 plants was heterozygous (Tt), where \"T\" represents the dominant allele for tallness and \"t\" represents the recessive allele for shortness. \- Second Filial Generation (F2): Mendel then allowed the F1 plants to self-pollinate. In the F2 generation, he observed a phenotypic ratio of approximately 3 tall plants to 1 short plant. The genotypic ratio was 1 homozygous dominant (TT) to 2 heterozygous (Tt) to 1 homozygous recessive (tt). This showed that while the tall trait was expressed in the F1 generation, the recessive short trait could reappear in the F2 generation. Mendel\'s model explains the continuity and variation in inherited traits through his Laws of Segregation and Independent Assortment. \- Law of Segregation states that during the formation of gametes, the two alleles for a trait separate so that each gamete carries only one allele. This explains why offspring can inherit different combinations of traits. \- Carriers are individuals who have one dominant and one recessive allele for a trait (heterozygous) but do not express the recessive trait (phenotype). This contributes to genetic variation in the population. \- Genotype refers to the genetic makeup of an organism (TT, Tt, or tt), while phenotype refers to the observable characteristics (tall or short). The presence of dominant and recessive alleles leads to different phenotypes being expressed in the offspring. In summary, Mendel\'s experiments demonstrated how traits can be inherited in predictable patterns while allowing for variation, laying the foundation for our understanding of genetics. 6\. Mendel's Particulate Model of Inheritance (4 main points) Particles, pairs, interact, Law of Segregation Answer: Mendel\'s Particulate Model of Inheritance is based on four main points: \- Particles: Mendel proposed that traits are determined by discrete units or \"particles,\" which we now refer to as genes. These particles are responsible for the inheritance of specific traits. \- Pairs: Each individual carries two alleles for each trait, one inherited from each parent. These alleles can be either dominant or recessive, and they exist in pairs. \- Interact: The alleles interact with each other during the formation of gametes. The dominant allele can mask the expression of the recessive allele, influencing the phenotype of the offspring. \- Law of Segregation: This law states that during the formation of gametes, the two alleles for a trait segregate from each other so that each gamete carries only one allele. This explains how traits can be passed on to the next generation in a predictable manner. Together, these points form the basis of Mendel\'s understanding of genetic inheritance, highlighting how traits are passed down through generations. 7\. Definitions: allele, genotype, phenotype, homozygous, heterozygous Answer: \- Allele: An allele is a variant form of a gene that can exist at a specific locus on a chromosome. Each individual inherits two alleles for each gene, one from each parent, which can be the same or different. \- Genotype: The genotype refers to the genetic makeup of an individual, specifically the combination of alleles they possess for a particular trait. It is often represented using letters, such as AA, Aa, or aa, where uppercase letters denote dominant alleles and lowercase letters denote recessive alleles. \- Phenotype: The phenotype is the observable physical or biochemical characteristics of an individual, which result from the interaction of the genotype with the environment. For example, a phenotype could be traits like flower color, height, or eye color. \- Homozygous: An individual is homozygous for a trait when they have two identical alleles for that trait. For example, if an individual has two dominant alleles (AA) or two recessive alleles (aa), they are considered homozygous. \- Heterozygous: An individual is heterozygous for a trait when they have two different alleles for that trait. For example, if an individual has one dominant allele and one recessive allele (Aa), they are considered heterozygous. These definitions are fundamental to understanding genetics and inheritance. 8\. Punnett squares for monohybrid crosses Answer: A Punnett square is a useful tool for predicting the outcomes of monohybrid crosses, which examine the inheritance of a single trait. Here's how to create and interpret a Punnett square for a monohybrid cross: \- Identify the Parents: Start by determining the genotypes of the two parents. For example, let\'s say we have one parent that is homozygous dominant (AA) and another that is homozygous recessive (aa). \- Set Up the Punnett Square: Draw a grid with two rows and two columns. Label the top of the columns with one parent\'s alleles (A and A) and the side of the rows with the other parent\'s alleles (a and a). \- Fill in the Punnett Square: Combine the alleles from each parent in the boxes of the grid. For our example: \- The first box (top left) will be AA (from A and A). \- The second box (top right) will be AA (from A and A). \- The third box (bottom left) will be aa (from a and a). \- The fourth box (bottom right) will be aa (from a and a). The completed Punnett square will look like this: \`\`\` A A Aa Aa ---- ---- Aa Aa a a Analyze the Results: From this Punnett square, we can conclude that all offspring (100%) will have the genotype Aa, which means they will all express the dominant phenotype. This method can be applied to any monohybrid cross to visualize the potential genetic combinations of the offspring. 9\. How is incomplete dominance produced? What patterns of inheritance? Answer: Incomplete dominance is a form of inheritance where neither allele is completely dominant over the other. Instead, the phenotype of the heterozygous individual is a blend of the two parental traits. This results in a third phenotype that is distinct from both homozygous forms. Here\'s how incomplete dominance is produced: \- Alleles Involved: In a typical scenario, you have two different alleles for a trait, such as a red flower allele (R) and a white flower allele (r). \- Homozygous Parents: When you cross two homozygous parents, one with the genotype RR (red flowers) and the other with rr (white flowers), the offspring will inherit one allele from each parent. \- Heterozygous Offspring: The resulting offspring will all have the genotype Rr. In incomplete dominance, this heterozygous genotype expresses a phenotype that is intermediate between the two homozygous phenotypes. So, instead of producing red or white flowers, the flowers of the heterozygous offspring will be pink. \- Patterns of Inheritance: Incomplete dominance follows a specific pattern of inheritance where the phenotypic ratio in the offspring can be observed through a Punnett square. For example, if we cross two pink flowers (Rr x Rr), the phenotypic ratio of the offspring would be: \- 1 Red (RR) \- 2 Pink (Rr) \- 1 White (rr) This results in a 1:2:1 ratio of phenotypes in the offspring. In summary, incomplete dominance produces a blended phenotype in heterozygous individuals, leading to distinct patterns of inheritance that can be predicted using Punnett squares. 10\. Cell division during growth and repair (Mitosis) and reproduction (Meiosis) Answer: Mitosis and meiosis are two types of cell division that serve different purposes in organisms. Mitosis is the process of cell division that occurs for growth and repair. It results in two genetically identical daughter cells, each with the same number of chromosomes as the original cell. Here's how it works: \- Phases of Mitosis: Mitosis consists of several phases: prophase, metaphase, anaphase, and telophase. \- In prophase, the chromosomes condense and become visible, and the nuclear membrane begins to break down. \- During metaphase, the chromosomes line up at the cell\'s equator. \- In anaphase, the sister chromatids are pulled apart to opposite poles of the cell. \- Finally, in telophase, the nuclear membrane reforms around each set of chromosomes, and the cell divides through cytokinesis. \- Purpose of Mitosis: Mitosis is essential for growth, tissue repair, and asexual reproduction in some organisms. It ensures that new cells have the same genetic material as the parent cell. Meiosis, on the other hand, is the type of cell division that occurs in the formation of gametes (sperm and egg cells) for sexual reproduction. It results in four genetically diverse daughter cells, each with half the number of chromosomes of the original cell. Here's how meiosis differs: \- Phases of Meiosis: Meiosis consists of two rounds of division: meiosis I and meiosis II. \- In meiosis I, homologous chromosomes pair up and exchange genetic material (crossing over) before being separated into two daughter cells. \- Meiosis II resembles mitosis, where the sister chromatids are separated, resulting in four unique gametes. \- Purpose of Meiosis: The main purpose of meiosis is to produce gametes for sexual reproduction, ensuring genetic diversity in the offspring. Each gamete has half the chromosome number, which is restored when fertilization occurs. In summary, mitosis is for growth and repair, producing identical cells, while meiosis is for reproduction, creating diverse gametes with half the chromosome number. 11\. End products of synthesis and mitosis Answer: The end products of synthesis and mitosis are quite distinct in terms of their functions and outcomes. During synthesis, which typically refers to the synthesis phase of the cell cycle (S phase), the primary end product is the replication of DNA. This means that each chromosome is duplicated, resulting in two identical copies called sister chromatids. This prepares the cell for mitosis, ensuring that when the cell divides, each daughter cell will receive a complete set of chromosomes. In mitosis, the end products are two genetically identical daughter cells. Each of these cells has the same number of chromosomes as the original parent cell. This process ensures that the genetic information is preserved and passed on accurately during cell division. To summarize: \- End product of synthesis: Replicated DNA (sister chromatids). \- End products of mitosis: Two identical daughter cells. These processes are crucial for growth, repair, and maintaining genetic consistency in cells. 12\. End products of synthesis, meiosis I, and meiosis II Answer: The end products of synthesis, meiosis I, and meiosis II each serve different purposes in the life cycle of cells. \- Synthesis (S phase): The end product of the synthesis phase is the replication of DNA. Each chromosome is duplicated, resulting in two identical sister chromatids for each chromosome. This ensures that genetic material is prepared for the upcoming cell division. \- Meiosis I: At the end of meiosis I, the outcome is two daughter cells, each with half the original chromosome number. These cells are haploid and contain one chromosome from each homologous pair. Importantly, genetic variation is introduced during this phase through crossing over and independent assortment. \- Meiosis II: The end product of meiosis II is four genetically diverse haploid gametes. Each of these gametes has one copy of each chromosome, resulting from the separation of sister chromatids. This diversity is crucial for sexual reproduction. In summary: \- End product of synthesis: Replicated DNA (sister chromatids). \- End products of meiosis I: Two haploid daughter cells. \- End products of meiosis II: Four genetically diverse haploid gametes. These processes are essential for growth, reproduction, and genetic diversity in organisms. 13\. Basic terminology and definitions: Answer: \- Chromosome: A structure made of DNA and proteins that contains genetic information. Humans have 23 pairs of chromosomes. \- Gene: A segment of DNA that contains the instructions for making a specific protein or functional RNA. Genes are the basic units of heredity. \- Locus: The specific physical location of a gene or a genetic marker on a chromosome. \- Allele: Different versions of a gene that may produce distinguishable phenotypic effects. For example, a gene for flower color may have a red allele and a white allele. \- Phenotype: The observable physical and physiological traits of an organism, which are determined by its genotype and environmental factors. For example, tall or short plant height. \- Genotype: The genetic makeup of an organism, consisting of the alleles it possesses for a particular gene. For instance, a genotype could be represented as AA, Aa, or aa. \- Haploid: A cell that contains a single set of chromosomes (n). Gametes (sperm and egg cells) are haploid in humans. \- Diploid: A cell that contains two sets of chromosomes (2n), one from each parent. Most somatic (body) cells in humans are diploid. \- Homologous Chromosomes: A pair of chromosomes, one inherited from each parent, that are similar in shape, size, and genetic content. \- Sister Chromatids: Identical copies of a chromosome that are connected by a centromere. They are formed during DNA replication and are separated during cell division. These terms are fundamental in understanding genetics and how traits are inherited. 14\. Basic structure of DNA (Figs. 9.4-9.6) sugar-phosphate backbone, nucleotides, complementary pairing Answer: \- Sugar-Phosphate Backbone: The backbone of the DNA molecule is made up of alternating sugar and phosphate groups. The sugar in DNA is deoxyribose. The phosphates link the sugars together, forming a long chain that provides structural support. \- Nucleotides: DNA is composed of units called nucleotides. Each nucleotide consists of three components: a phosphate group, a deoxyribose sugar, and a nitrogenous base. There are four types of nitrogenous bases in DNA: adenine (A), thymine (T), cytosine (C), and guanine (G). \- Complementary Pairing: The nitrogenous bases pair specifically with each other through hydrogen bonds, creating the rungs of the DNA ladder. Adenine pairs with thymine (A-T), and cytosine pairs with guanine (C-G). This complementary pairing is crucial for the replication of DNA and ensures that genetic information is accurately transmitted. Together, these components form the double helix structure of DNA, which is essential for storing and transmitting genetic information in living organisms. 15\. How does the DNA sequence get turned into a cell's phenotype? Transcription and Translation Central Dogma, nucleus and ribosome, DNA, RNA, and protein Answer: The process by which a DNA sequence is turned into a cell\'s phenotype involves two main steps: transcription and translation. This process is often referred to as the Central Dogma of molecular biology, which outlines the flow of genetic information. \- Transcription: This first step occurs in the nucleus of the cell. During transcription, the DNA sequence of a gene is copied into a messenger RNA (mRNA) molecule. The enzyme RNA polymerase binds to the DNA at the promoter region and unwinds the DNA strands. It then synthesizes the mRNA strand by pairing RNA nucleotides with the complementary DNA bases, following the base-pairing rules (A-U and C-G). Once the mRNA is synthesized, it undergoes processing, which includes the addition of a 5\' cap and a poly-A tail, and the removal of introns (non-coding regions). \- Translation: After transcription, the mature mRNA molecule is transported from the nucleus to the ribosome, which is the site of protein synthesis. During translation, the ribosome reads the mRNA sequence in sets of three nucleotides called codons. Each codon corresponds to a specific amino acid. Transfer RNA (tRNA) molecules bring the appropriate amino acids to the ribosome, where they are linked together in the order specified by the mRNA sequence. This process continues until a stop codon is reached, resulting in the formation of a polypeptide chain that folds into a functional protein. The proteins produced through this process ultimately determine the cell\'s phenotype, as they play crucial roles in the structure and function of the cell, influencing traits and characteristics. In summary, the flow of genetic information from DNA to RNA to protein is how the DNA sequence translates into a cell\'s phenotype. 16\. Transcription- in the nucleus (Figs. 10.1-10.2) Answer: Transcription is a critical process that occurs in the nucleus of a cell, where the genetic information encoded in DNA is converted into messenger RNA (mRNA). Here's how it works: \- Promoter and Terminator Sequences: Transcription begins at a specific region of the gene called the promoter. The promoter contains specific sequences that signal RNA polymerase where to start transcribing the DNA. Once RNA polymerase binds to the promoter, it unwinds the DNA strands. At the end of the gene, there are terminator sequences that signal RNA polymerase to stop transcription. \- RNA Polymerase: This enzyme plays a crucial role in the transcription process. After binding to the promoter, RNA polymerase moves along the DNA template strand, synthesizing a complementary mRNA strand. It adds RNA nucleotides one by one, matching them with the corresponding DNA bases (adenine pairs with uracil in RNA, and cytosine pairs with guanine). \- Production of mRNA in the Nucleus: As RNA polymerase continues to transcribe the DNA, it creates a single-stranded mRNA molecule that is a copy of the gene. Once the RNA polymerase reaches the terminator sequence, transcription ends, and the newly formed mRNA molecule is released. Following transcription, the mRNA undergoes further processing, including the addition of a 5\' cap and a poly-A tail, and the removal of non-coding sequences (introns). The mature mRNA then exits the nucleus and enters the cytoplasm, where it will be translated into a protein. In summary, transcription in the nucleus involves promoter and terminator sequences, the action of RNA polymerase, and the production of mRNA, which is essential for protein synthesis. 17\. Translation (Figs. 10.10-12) interactions between mRNA codon and tRNA molecules in the ribosome charged and uncharged tRNA molecules Answer: Translation is the process by which the information encoded in mRNA is used to synthesize proteins. This occurs in the ribosome and involves several key components, including mRNA codons and tRNA molecules. \- Interactions Between mRNA Codon and tRNA Molecules: The mRNA is read in sets of three nucleotides called codons. Each codon corresponds to a specific amino acid. Transfer RNA (tRNA) molecules carry the amino acids to the ribosome. Each tRNA has an anticodon region that is complementary to the mRNA codon. When the ribosome reads an mRNA codon, the corresponding tRNA with the matching anticodon binds to it, ensuring that the correct amino acid is added to the growing polypeptide chain. \- Charged and Uncharged tRNA Molecules: A charged tRNA molecule is one that is attached to its specific amino acid. This process is called \"charging\" and is catalyzed by enzymes known as aminoacyl-tRNA synthetases. An uncharged tRNA, on the other hand, does not have an amino acid attached. During translation, charged tRNA molecules enter the ribosome, donate their amino acids, and then exit as uncharged tRNA after the amino acid has been added to the polypeptide chain. In summary, translation involves the interaction between mRNA codons and charged tRNA molecules in the ribosome, which facilitates the assembly of amino acids into proteins. The process continues until a stop codon is reached, signaling the end of protein synthesis. 18\. Why do changes in the DNA sequence result in changes in phenotype? explain it in terms of protein structure (primary, secondary, tertiary & quaternary) Answer: Changes in the DNA sequence can lead to changes in phenotype because the DNA sequence encodes the instructions for synthesizing proteins, which play crucial roles in determining an organism\'s traits. \- Primary Structure: The primary structure of a protein is its unique sequence of amino acids. This sequence is directly determined by the DNA sequence of the gene. A mutation in the DNA can lead to a different amino acid being incorporated into the protein. For example, if the DNA sequence changes from coding for alanine to coding for valine, the primary structure of the protein is altered. \- Secondary Structure: The secondary structure refers to local folded structures that form within a protein due to hydrogen bonding. Changes in the primary structure can affect how the protein folds into its secondary structure. If an amino acid is changed to one with different properties (like size or charge), it may disrupt hydrogen bonds or other interactions, leading to altered secondary structures like alpha-helices or beta-sheets. \- Tertiary Structure: The tertiary structure is the overall three-dimensional shape of a protein, formed by interactions among various amino acids and their side chains. Changes in the primary and secondary structures can influence the tertiary structure. A misfolded protein can lose its functionality, potentially leading to diseases or altered phenotypic traits. \- Quaternary Structure: Some proteins consist of multiple polypeptide chains, forming a quaternary structure. Changes in the DNA sequence can affect the interactions between these chains. If one chain is altered, it may affect how the chains come together, ultimately impacting the protein\'s function and, consequently, the phenotype. In summary, changes in the DNA sequence can lead to changes in the amino acid sequence of proteins, which can affect their folding and structure at all levels. These structural changes can alter the protein\'s function, leading to observable changes in phenotype. 19\. Mutations, point mutations (substitutions, insertions & deletions) Missense and nonsense substitutions Frameshift mutations- insertions/deletions Why don't all mutations have equivalent effects on phenotypes? Answer: Mutations are changes in the DNA sequence that can have various effects on an organism\'s phenotype. Not all mutations have equivalent effects, and here\'s a breakdown of the different types of mutations and their potential impacts: 1\. Point Mutations: These are small changes in the DNA sequence that affect only one nucleotide. They can be categorized into three types: \- Substitutions: One nucleotide is replaced by another. This can be further divided into: \- Missense Mutations: These substitutions result in a different amino acid being incorporated into the protein. Depending on where this change occurs and the properties of the new amino acid, it can have a significant or negligible effect on the protein\'s function. \- Nonsense Mutations: These substitutions create a premature stop codon, leading to a truncated protein that is usually nonfunctional. This can have a severe impact on phenotype. 2\. Insertions and Deletions: These mutations involve the addition or loss of nucleotides in the DNA sequence. They can lead to: \- Frameshift Mutations: When nucleotides are inserted or deleted in numbers that are not multiples of three, it shifts the reading frame of the codons. This typically results in a completely altered protein sequence downstream of the mutation, often producing a nonfunctional protein and significantly affecting phenotype. Not all mutations affect phenotypes equally because: \- Location of Mutation: Mutations in non-coding regions or introns may not affect protein function and thus have no phenotypic effect. \- Redundancy in the Genetic Code: Some amino acids are encoded by multiple codons. A substitution that changes one codon to another that codes for the same amino acid may have no effect (silent mutation). \- Protein Functionality: Some changes in amino acids may not significantly alter the protein\'s overall structure or function, while others can drastically change its activity or stability. In conclusion, the effect of mutations on phenotype depends on the type of mutation, where it occurs in the gene, and how it impacts the protein\'s structure and function.