Task 2 - Neurons PDF
Document Details
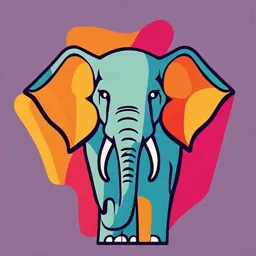
Uploaded by TemptingGrace7856
Maastricht University
Tags
Summary
This document provides an overview of neurons, their structure, types, and functions within the nervous system. It explains the functions of neurons and different interactions between them. It also describes the process of neural integration and synaptic transmission.
Full Transcript
Task 2 - Neurons Learning Goals What is the structure and function of neurons? What are the different types of neurons? What is the structure and function of the neuron's cell membrane. What is a receptor? What is an action potential? What are the different phases of an action potential and what ro...
Task 2 - Neurons Learning Goals What is the structure and function of neurons? What are the different types of neurons? What is the structure and function of the neuron's cell membrane. What is a receptor? What is an action potential? What are the different phases of an action potential and what roles do different ions play in each phase? How does synaptic transmission work? Cells in the nervous system The nervous system contains 2 types of cells: neurons and glial cells (glia = glue). A neuron is a cell that is specialized for the reception, conduction and transmission of electrochemical signals. There are 80-90 billion neurons in the adult human brain. Glia provide various forms of support and also contribute to information processing. In the human brain, there are roughly equal numbers of neurons and glia. The neuron doctrine - a perspective that proposes that information is transmitted from one neuron to the next across tiny gaps (synapses). The neuron doctrine is most likely an oversimplification because it excludes glia. Anatomy of neurons External features of neurons Almost all neurons share some distinctive structures that are directly related to information processing: Dendrites - cellular extensions that receive information from other neurons across synapses. They can accommodate synapses from many other neurons. Cell body (soma) - contains the cell nucleus and combines the information that the neuron has received to determine whether or not to send a signal of its own. Axon - a long thin tube that leaves the cell body and serves as a conduction zone. It is covered by a myelin sheath - a layer of insulating substance called myelin. Axons carry the electrical signals produced by the neuron away from the cell body towards the axon terminals (or terminal buttons). Most axons divide & branch many times. At the end of the branches are the axon terminals (or synaptic buttons). They transmit the neuron's activity across synapses to other cells. Nodes of Ranvier are the gaps between sections of myelin in the axon. Classifications of neurons Neurons can be classified on the basis of processes (projections) coming out of their cell bodies: Multipolar neuron - a neuron with many sets of dendrites and a single axon. 99% of all neurons are multipolar. Unipolar neuron - a neuron with only 1 process extending from its cell body. The process branches in 2 directions after leaving the cell body. One of the ends is an input zone, the other - an output zone. Such cells transmit touch information from the body into the spinal cord. Bipolar neuron - a neuron with 1 dendrite at the one end of the cell and a 1 axon on the other end. Very common in sensory systems (e.g. vision) Multipolar interneuron - a neuron with a short axon or no axon at all. They integrate neural activity within a single brain structure, instead of Body and Behavior Page 1 They integrate neural activity within a single brain structure, instead of conducting signals to other neurons. In multipolar and bipolar cells, the cell body also receives synapses and so is also part of the input zone. Neurons can also be classified in terms of their function: Sensory neurons - in the PNS, they gather information in the form of light, sound waves, odors, tastes, contact with objects etc. Motor neurons - control the contraction of muscles, facilitating movements. Interneurons - neurons that lie entirely within the CNS. Local interneurons form circuits with nearby neurons and analyze small pieces of information. Relay interneurons connect circuits of local interneurons in one region of the brain with those in other regions. Example on the left: a person sees the glass of water and sensory nerves relay the sensory information from the eyes towards the CNS. Then, the motor output from the CNS allows the person to lift the glass. Synapses A synapse consists of 3 primary components: Presynaptic membrane of the axon terminal of the presynaptic neuron. Synaptic cleft - a gap of 20-40 nanometers that separates the presynaptic and postsynaptic neurons. Postsynaptic membrane on the surface of the dendrite or the cell body of the postsynaptic neuron. Presynaptic axon terminals contain many tiny hollow spheres, called synaptic vesicles. Each vesicle contains neurotransmitters - a molecule which the neuron uses to communicate with the postsynaptic neurons. In response to electrical activity in the axon, these vesicles fuse with the presynaptic membrane and rupture, releasing the neurotransmitter molecules into the synaptic cleft (exocytosis). The released neurotransmitters interact with postsynaptic receptors: protein molecules that capture and react to the neurotransmitter. Neurotransmitters do not enter the postsynaptic neuron; they simply bind to the receptors momentarily, and then diffuse in the extracellular fluid in the synaptic cleft. Each synapse occupies a tiny patch of the postsynaptic neuron. Some neurons receive as many as 100,000 synaptic contacts, although a more common number is 5,000-10,000. Neural plasticity - the configuration of synapses on a neuron's dendrites and cell body is constantly changing - synapses come and go and dendrites change their shape. Some dendritic spines (tiny protrusions from the surface of some dendrites) can change from minute to minute, while others may be stable for a lifetime. Axon hillock - a cone-shaped projection of the cell body where the axon starts. It is the neuron's integration zone. Axoplasmic transport The terminal buttons need items that can be produced only in the cell body. Axoplasmic transport is a process that propels Body and Behavior Page 2 the cell body. Axoplasmic transport is a process that propels substances along microtubules that run inside the length of the axon. Anterograde axoplasmic transport - movement from the cell body to the terminal buttons. Accomplished by a protein called kinesin, which resembles a pair of legs and feet. A kinesin molecule attaches to the item being transported down the axon and carries the cargo to the terminal buttons. Retrograde axoplasmic transport - movement of substances from terminal buttons to the cell body. Performed by a protein called dynein. Other internal features of neuron Cell membrane defines the boundary of a neuron. It is composed of a lipid bilayer - 2 layers of lipid (fatlike) molecules. Many proteins are embedded in the lipid bilayer: Channel proteins - certain molecules can pass through them. Signal proteins - they transfer a signal to the inside of the neuron when specific molecules bind to them on the outside of the membrane (e.g. hormones). Cytoskeleton - a matrix of strands of protein that gives the neuron its shape. Microtubules - the thickest of these strands Cytoplasm - a jellylike substance that fills the space inside the membrane. It contains the cell's organelles. Cell nucleus - an oval structure in the soma. The nucleolus and chromosomes are inside the nucleus. Nucleolus - responsible for production of ribosomes - small structures involved in protein synthesis. Chromosomes - consist of long DNA strands and contain the organism's genetic information. Proteins are produced in a 2-step process: 1. Information from DNA (which cannot leave the nucleus) is transcribed into a portable form mRNA (messenger RNA). 2. mRNA takes this information to the ribosomes, where translation occurs: information from the mRNA is used to create proteins. Endomembrane system - comprised of endoplasmic reticulum, Golgi apparatus and lysosomes. The endoplasmic reticulum and Golgi apparatus work together with the rest of the organelles in the nucleus to produce and transport protein to the various locations inside and outside cell. Lysosomes, produced by the Golgi apparatus are small sacs that contain enzymes that break down substances no longer needed by the cell. Mitochondria - cells provide mitochondria with nutrients, and mitochondria provide cells with a special molecule - adenosine triphosphate (ATP) - that cells use as their immediate source of energy. Body and Behavior Page 3 Glial cells Astrocytes (star cell) are the largest glial cells. Some astrocytes' extensions cover blood vessels in the brain, but they also make contact with neurons. The following functions of astrocytes have been discovered. They: ○ Exchange chemical signals with neurons and other astrocytes. They form three-way synapses with neighboring neurons and directly participate in the transmission of information between neurons. ○ Control the establishment and maintenance and pruning of synapses between neurons. ○ Form functional networks with neurons and other astrocytes. ○ Control the blood-brain barrier. Relax/contract blood vessels based on blood flow demands of certain brain regions. ○ Respond to brain injury Neurons have a very high rate of metabolism but have no means of storing nutrients. They receive most of their nutrients from astrocytes. Microglia are smaller than other glial cells. They respond to sites of damage by forming a spherical containment zone around the injury. They migrate to sites of injury or disease in the nervous system to remove debris from injured or dead cells. They also play a role in synapse formation and pruning. Oligodendrocytes are glial cells with extensions that wrap around the axons of some neurons of the CNS. They perform myelination (formation of myelin sheaths, which increase speed and efficiency of axonal conduction). It also insulates axons from one another. Schwann cells are glia in the PNS, which perform myelination there. Each segment of myelin is a single Schwann cell wrapped many times around the axon. Each Schwann cell constitutes only 1 myelin segment, contrary to oligodendrocytes, which provide several myelin segments, often on more than 1 axon. Schwann cells can guide axonal regeneration after damage, but oligodendrocytes cannot, due to lack of ability to penetrate a barrier of scar tissue produced by the astrocytes. This is why effective axonal regeneration in the mammalian nervous system is restricted to the PNS. Multiple sclerosis (MS) is a disease where the immune system attacks myelin sheaths , leaving behind hard patches of debris. It causes a wide variety of neurological symptoms. An interesting fact is that the myelin differs in composition between the CNS and PNS and the immune system of someone with MS attacks only the myelin protein produced by oligodendrocytes (thus the myelin of the PNS is spared). Changes in glia, especially loss of oligodendrocytes and their associated myelin, are implicated in the onset and symptoms of schizophrenia. Body and Behavior Page 4 Action potentials Membrane potential - any difference in charge across the membrane (positive or negative). When a neuron is at rest and not involved in communicating with any other neurons, the membrane potential remains at approximately -70 millivolts (mV). This is called the neuron's resting potential. A neuron at resting state is polarized. When neurotransmitters bind to postsynaptic receptors, they can: a) Depolarize the receptive membrane (decrease the resting potential, from -70 to -67 mV for example). Postsynaptic depolarizations are called excitatory postsynaptic depolarizations (EPSPs), because they increase the likelihood that the neuron will fire. b) Hyperpolarize the receptive membrane (increasing the resting potential from -70 to -72mV for example). Postsynaptic hyperpolarizations are called inhibitory postsynaptic depolarizations (IPSPs). Action potential - a burst of rapid depolarization followed by hyperpolarization. It begins at the axon initial segment (a compartment at the base of an axon) and propagates like a wave till the end of the terminal buttons, informing them to release neurotransmitters into the synapse. Each neuron has a threshold of excitation - a point after which depolarization triggers an action potential. The membrane potential The membrane potential is the result of 2 opposing forces: diffusion & electrostatic pressure. Diffusion - the process whereby molecules distribute themselves evenly throughout the medium in which they are dissolved. Molecules are constantly in motion, and their rate of movement is proportional to the temperature. Only at absolute zero [0 K = –273.15° C], they stop their random movement. At all other temperatures, they constantly move in different directions and collide. When there are no forces/barriers to prevent them from doing so, molecules will naturally diffuse from regions of high concentration to regions of low concentration. Electrostatic pressure - the force exerted by the attraction/repulsion of ions. When some substances are dissolved in water, they split into 2 parts with opposing electrical charge (ions). Substances that have this property are called electrolytes. ○ Cations - positively charged ions (e.g. Na+ - sodium cation). ○ Anions - negatively charged ions (e.g. Cl- chloride anion). Electrostatic pressure pushes cations away from regions with an excess of cations, and anions away from regions with an excess of anions. The intracellular and extracellular fluids (within and surrounding cells) contain different ions: Organic anions (A-) - negatively charged proteins and intermediate products of the cell's metabolic processes. Only in the intercellular fluid. Chloride (Cl-) and sodium (Na+) ions - predominantly in the extracellular fluid. Potassium ions (K+) - predominantly in the intracellular fluid Easy to remember by remembering that the extracellular fluid resembles seawater, which is mostly a solution of salt, NaCl. Why is every ion wherever it is Body and Behavior Page 5 The A- ion is unable to pass through the membrane. K+ is concentrated inside the axon. Diffusion tends to push it outside. However, the outside of the cell is positively charged relative to the inside, so electrostatic pressure tends to force the cation inside. The 2 opposing forces balance each other. Cl- is concentrated outside the axon. Diffusion tends to push it inward. Electrostatic pressure pushes it outward. The 2 opposing forces balance each other. Na+ is also concentrated outside the axon. Diffusion tends to push it inward. Electrostatic pressure also tends to push it inward. Na+ ions are attracted by the inside by both forces. How do they stay outside? By being constantly pumped out the axon by sodium- potassium pumps. A sodium-potassium pump consists of many proteins (called sodium-potassium transporters) embedded in the membrane. The sodium-potassium transporters exchange Na+ for K+, pushing 3 sodium ions out for every 2 potassium ions they push in. This way they also slightly increase the intercellular concentration of K+ ions. Another reason why extracellular Na+ concentration is higher is that the membrane is not very permeable to Na+ ions. On the other hand, it is moderately permeable to K+ ions. The transporters that make up the sodium- potassium pump use up to 40% of a neuron's metabolic resources. Most cells of the body (including neurons, muscle cells and glia) have sodium-potassium transporters in their membrane. Ion movements during the generation of an action potential Ion channels - structures of proteins that permit ions to enter or leave the cells. Ion channels contain passages (pores) that can open or close. When an ion channel is open, specific ions can flow through the pore. Neural membranes contain thousands of ion channels. The permeability of a membrane to a particular ion at a given moment is determined by the number of ion channels that are open. Some ion channels are called voltage-dependent ion channels because they are only opened by changes in the membrane potential. The action potential consists of a series of changes in opening and closing of ion channels and the resulting distribution of ions: 1. The membrane potential must reach a threshold of excitation. As soon as that happens, the sodium channels open, and Na+ rushes in, propelled by the forces of diffusion and electrostatic pressure. The influx of positively charged Na+ ions produced a rapid change in the membrane potential, from -70mV to +40mV. 2. The membrane of the axon contains voltage-dependent potassium Body and Behavior Page 6 2. The membrane of the axon contains voltage-dependent potassium channels, which are less sensitive than the voltage-dependent sodium channels (they require a greater level of depolarization before they begin to open). Therefore, they begin to open later than the sodium channels. 3. When the action potential reaches its peak (~1 millisecond), the sodium channels become refractory (blocked) and cannot open again until the membrane reaches the resting potential again. When this point is reached, no more Na+ can enter the cell. ○ Absolute refractory period - the period after the initiation of an action potential, during which it is impossible to elicit a second one (due to blockage of the Na+ channels). 4. At this point the voltage-dependent potassium channels are already open, letting K+ ions flow out of the membrane (because the inside of the cell is positively charged so K+ are driven out by diffusion and electrostatic pressure). This outflow of K+ ions causes the membrane potential to return to its normal value, causing the potassium channels to close again. 5. Once the membrane potential returns to normal, sodium channels reset (so that another depolarization can cause them to open again). ○ Relative refractory period - after the absolute refractory period; it is possible to fire the neuron again but only by applying higher-than- normal levels of stimulation (because the neuron is hyperpolarized). 6. The membrane overshoots its resting value (-70mV) and only gradually returns to normal as the potassium channels finally close. Eventually, the sodium-potassium pumps remove the Na+ ions that leaked in and retrieve the K+ ions that leaked out. Conduction of the action potential All-or-none law - the action potential either occurs or does not occur. Once triggered, it is transmitted down the axon to its end. It always remains the exact same size, without growing or diminishing (even when it reaches a point where the axon branches - it splits but does not diminish in size). The rate law - variable information (e.g. intensity of stimulus) is not represented by action potential size, but by rate of firing action potentials. For example, an axon might respond to dim light by firing 10 action potentials in a unit of time, but the same axon might respond to a bright light by firing 100 action potentials in the same unit of time. A myelinated axon is isolated from the extracellular fluid, except at the nodes of Ranvier. In the myelinated areas, there can be no inward flow of Na+, because there is not extracellular sodium. In myelinated axons, axonal sodium channels are concentrated at the nodes of Ranvier. The axon conducts the electrical signal from the action potential to the next node of Ranvier. The signal gets smaller as it passes down the axon (decremental conduction), but it is still large to trigger a new action potential at the next node of Ranvier. At each node of Ranvier, the action potential gets retriggered, and the electrical signal that results is conducted decrementally along the myelinated area to the next node of Ranvier. This way of transmission (hopping from node to node) is called saltatory conduction. Saltatory conduction is economic. Because Na+ enters axons only at the nodes of Ranvier, much less gets in, and consequently much less has to be pumped out again => myelinated axons expend much less energy to maintain their sodium balance. Conduction of an action potential is faster in a myelinated axon because the transmission between the nodes is very fast. This model of neural conduction is based on the Hodgkin-Huxley model, which is based on the study of squid motor neurons. Research shows that mammalian cerebral neurons have properties that are not included in this model: Many cerebral neurons fire continually even when they receive no input. The dendrites of some cerebral neurons can actively conduct action potentials. Some do not display action potentials at all. Axons of some cerebral neurons can actively conduct both graded signals and action potentials. Depending on the class of cerebral neuron, action potentials vary greatly in duration, amplitude and frequency. How does synaptic transmission work? Many terminal buttons contain 2 types of synaptic vesicles: large and small. Small synaptic vesicles (found in all terminal buttons) contain molecules of a neurotransmitter. They are produced in the Golgi apparatus in the soma and are carried by fast axoplasmic transport to the terminal button. Some are also produced from recycled material in the terminal button. Large synaptic vesicles contain 1 or more neuropeptides (short proteins composed of 3-36 amino acids). They are produced only in the soma and are transported through the axoplasm to the terminal buttons. Transport proteins fill the synaptic vesicles with the neurotransmitters, and trafficking proteins are involved in the release of neurotransmitters and recycling of Body and Behavior Page 7 Transport proteins fill the synaptic vesicles with the neurotransmitters, and trafficking proteins are involved in the release of neurotransmitters and recycling of the vesicles. Release of neurotransmitters Docking - a cluster of protein molecules attach to other protein molecules located in the presynaptic membrane, effectively "parking" (docking) the vesicle against the presynaptic membrane. Release zone - the region of the presynaptic membrane that faces the synaptic cleft, where neurotransmitters are released from. The release zone contains voltage-dependent calcium channels. When the action potential arrives, the Ca2+ ions (which like the Na+ ions are concentrated more in the extracellular fluid) flow into the cell propelled by electrostatic pressure and diffusion. Later, calcium transporters, similar in operation to the sodium-potassium pumps, remove the intracellular Ca2+. If neurons are placed into a solution that contains no Ca2+ ions, an action potential no longer causes release of neurotransmitters. Some of the Ca2+ ions that flow in bind with the clusters of protein molecules that join the membrane of the synaptic vesicles with the presynaptic membrane. This produces a fusion pore - a hole through both membranes that enables them to fuse together. There are 3 pools of synaptic vesicles: Release-ready vesicles - docked and ready to release contents when action potential occurs (less than 1% of the ones found in the terminal). Recycling pool - 10-15% of the total pool of vesicles. Reserve pool - the remaining 85-90%. If an axon fires at a low rate, only vesicles from the release- ready pool are used. As rate increases, the recycling pool and then the reserve pool are used. Kiss and run - many vesicles release most/all of their neurotransmitter, the fusion pore closes, the vesicles leave the docking site and get filled with neurotransmitter again. Merge and recycle - some vesicles (mostly those in the recycling pool) merge with the cell membrane and lose their identity. Little buds of membrane later pinch off into the cytoplasm and become new synaptic vesicles. The proper proteins are inserted into the new vesicles' membranes, and the vesicles are filled with neurotransmitter. Bulk endocytosis (endocytosis is the process of entering a cell) is the process by which vesicles in the reserve pool are recycled: large pieces of terminal membrane fold inward, Time: nothing happens, because diffusion and electrostatic pressure balance the Cl - ion perfectly. ○ When already depolarized => Cl- enters the cell resulting in the restoration of resting potential. Therefore, the opening of chloride channels results into IPSPs. When calcium channels are opened, Ca2+ ions flow inside the cell, resulting in an EPSP (just like sodium channels). Furthermore, in the dendrites of the postsynaptic cell, Ca2+ binds with and activates special enzymes, which can produce biochemical and structural changes in the postsynaptic neuron. One of the ways in which learning affects the connections between neurons involves changes in dendritic spines initiated by t he opening of calcium channels. Body and Behavior Page 9 Neural integration Neural integration - the interaction of the effects of excitatory and inhibitory synapses on a particular neuron. IPSPs can counteract EPSPs, leading to action potential not being triggered even in the presence of PSPs. PSPs are graded responses: their amplitudes depend on the intensity of signals that elicit them. The transmission of PSPs is decremental: they decrease in amplitude as they travel towards the axon initial segment. If the sum of the depolarizations and hyperpolarizations reaching the axon initial segment is sufficient to surpass the threshold of excitation, an action potential is generated. It was believed that the location of a synapse on the postsynaptic neuron's membrane is a crucial factor in determining the influence of the PSPs on the neuron's firing. However, some neurons have a mechanism for amplifying dendritic signals that originate far from their axon initial segments. Make distinction between action potentials and postsynaptic potentials: the former are neither graded (they are all-or-none) nor decremental (they arrive at the terminal buttons at full intensity). Neural integration happens in 2 ways: over space and time. Spatial summation - simultaneous PSPs produced on different physical locations across the cell body are integrated, taking into account their intensity and type (inhibitory/excitatory). Temporal summation - PSPs produced in rapid succession sum to form a greater signal. Termination of postsynaptic potentials Reuptake - special transporter molecules force neurotransmitter molecules from the synaptic cleft directly into the cytoplasm (the same way sodium-potassium transporters move Na+ and K+ across the membrane). Enzymatic deactivation (degradation) - an enzyme destroys molecules of the neurotransmitter. PSPs are terminated by enzymatic deactivation for acetylcholine (ACh) and for neurotransmitters that consist of peptide molecules. Other types of synapses Axodendritic synapses - synapses of axon terminal buttons on dendrites or dendritic spines. Axosomatic synapses - synapses of axon terminal buttons on cell bodies. Axoaxonic synapses - synapses that occur between 2 terminal buttons. Axoaxonic synapses do not contribute directly to neural integration, but they alter the neurotransmitter amount released by the buttons of the postsynaptic axon (presynaptic modulation). Presynaptic inhibition - if the activity of an axoaxonic synapse decreases the release of neurotransmitter. Presynaptic facilitation - if the activity of an axoaxonic synapse increases the release of neurotransmitter. Dendrodendritic synapses - synapses between dendrites (formed by very small neurons which have extremely short processes and lack axons). Little is known about the function of these neurons. Some larger neurons also form dendrodendritic synapses. Some are chemical (i.e. there are synaptic vesicles in one of the dendrites and a postsynaptic thickening in the membrane of the other). Others are electrical: the membranes meet and almost touch, forming a gap junction. Both membranes contain channels (connexins) that permit ions to diffuse from one cell to another (thus changes in membrane potential of 1 neuron induces changes in the membrane of the other). The function gap junctions is not known. Body and Behavior Page 10