Analgesics PDF
Document Details
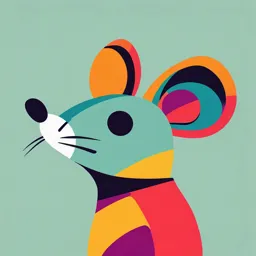
Uploaded by HealthyBromine
Tahir Muhammad
Tags
Summary
This document provides an overview of analgesics. It describes the different types of analgesics, including strong (opioid) and weak (NSAIDs) analgesics, and further explains their mechanisms of action. It also examines the types of pain, pain fibers, and the pain pathways involved. Finally, it discusses the various opioid receptors and the endogenous opioids within the body, presenting a strong emphasis on the pharmacology behind these.
Full Transcript
Tahir Muhammad ANALGESICS DEFINITION: “Drugs that relieve pain are called analgesic.” OR “A drug that selectively relieves pain by acting in the CNS or on p...
Tahir Muhammad ANALGESICS DEFINITION: “Drugs that relieve pain are called analgesic.” OR “A drug that selectively relieves pain by acting in the CNS or on peripheral pain mechanisms, without significantly altering consciousness.” The drugs that relieve pain by acting at some points in the body’s pain signaling mechanism (peripheral, spinal, supraspinal) are called analgesics. ALGESIA/PAIN: “Characteristic sensation arise from a noxious sensation” Pain is a warning signal, primarily protective in nature, but causes discomfort and suffering; may even be unbearable and incapacitating. It is the unpleasant sensory & emotional experience associated with actual tissue t damage. Pain is also considered as a complex body response to a noxious stimulus followed by emotional response. Excessive pain may produce other effects like sinking sensation, apprehension, sweating, nausea, palpitation, and rise or fall in BP, tac tachypnoea etc. TYPES OF PAIN: Chronic pain; Pain is transitory, lasting only until the noxious stimulus is removed, eg, rheumatoid arthritis, peripheral neuropathy, cancer, & idiopathic pain Lasts ↑ than 1 month → Having long duration Acute pain; The pain that resolve quickly is called acute pain. Lasts less than 30 days → ↓ duration PAIN FIBERS: Three main types of fiber, A-fiber A-alpha fiber 1 Tahir Muhammad A-beta fiber A-gamma fiber A-delta fiber B-fiber C-fiber → most imp Pain pathway: From periphery the pain through pain fibers is transmitted to the spinal cord and then higher centers of brain. Periphery → spinal cord → higher centers of brains. Neospinothalamic tract: Fast pain travels via type Aδ fibers to terminate in the dorsal horn of the spinal cord where they synapse on dendrites of the neospinothalamic tract. The axons of these neurons cross the midline (decussate) through the anterior and ascend contralaterally along the anterolateral columns. columns These fibers terminate on the ventrobasal complex of the thalamus and synapse with the dendrites of the somatosensory cortex. Fast pain is felt within a tenth of a secon secondd of application of the pain stimulus and is a sharp, acute, prickling pain,& felt in response to mechanical and thermal stimulation. It can be localized easily if Aδ fibers are stimulated together with tactile receptors. Paleospinothalamic tract 2 Tahir Muhammad Slow pain is transmitted via slower type C fibers to laminae II and III of the dorsal horns, together known as the substantia gelatinosa. Impulses are then transmitted to nerve fibers that terminate in lamina-V, also in the dorsal horn, synapsing with neurons that join fibers from the fast pathway, crossing to the opposite side via the anterior white commissure, and traveling upwards through the anterolateral pathway. These neurons terminate throughout the brain stem, with one tenth of fibers stopping in the thalamus and the rest stopping in the medulla, pons and periaqueductal grey of the midbrain tectum. Slow pain is stimulated by chemical stimulation, is poorly localized and is described as an aching, throbbing or burning pain. Putative sites of action of opioid analgesics (darker color). On the left, sites of action on the pain transmission pathway from the periphery to the higher centers are shown. A: Direct action of opioids on inflamed peripheral tissues. B: Inhibition occurs in the spinal cord. C: Possible site of action in the thalamus. Different thalamic regions project to somatosensory (SS) or limbic (L) cortex. Parabrachial nuclei (medulla/pons) projects to the amygdala. On the right, actions of opioids on pain-modulating neurons in the midbrain (D) and medulla (E) indirectly control pain transmission pathways. 3 Tahir Muhammad TYPES OF ANALGESICS: There are two main classes of analgesics which are; A) CLASS-1 / STRONG / OPOIDS / NARCOTIC ANALGESICS: Morphine is prototype drug of this class. These drugs acts centrally at spinal or supraspinal level (brain). Used for severe pain → such as pain arising from burns, neoplasm, etc Strong analgesics (these ) are used, where there other analgesics can’t works properly. Having sedative & analgesic properties. DISADVANTAGE The big disadvantage of this class is addiction, but still used as analgesic b/c some severe pain can’t be controlled without this drug such as cancer pain. B) CLASS-2 / WEAK ANALGESICS / NSAIDS: Here aspirin is prototype drug. Mostly used in musculoskeletal pain. They have also anti-inflammatory properties. Some have anti-pyretic activity also. The imp neurotransmitters such as adrenalin and 5-OH-tryptamine also relieve pain in CNS. Classification of Analgesics: Opioids (Type-1)→ narcotic, centrally acting & strong NSAIDS (Type-2) → non-narcotic peripherally acting, &weak OPIOIDS Definition: “The term opioids applied to any substance whether endogenous or synthetic that act like morphine, and its effects are antagonized by Naloxone.” Introduction: Morphine, the prototypical opioid agonist, has long been known to relieve severe pain with remarkable efficacy. The opium poppy is the source of crude opium from which Serturner in 1803 isolated morphine, the pure alkaloid and named it after Morpheus, the Greek god of dreams. It remains the standard against all the drugs that have strong analgesic action. Source: Obtained from the poppy → Papaver somniferum 1) Opioids Receptors: Opioids interact stereospecifically with protein receptors on the membranes of certain cells in the CNS, on nerve terminals in the periphery, and on cells of the gastrointestinal tract and other anatomic regions. 4 Tahir Muhammad The major effects of the opioids are mediated by three major receptor families. These are designated by the Greek letters µ-Receptor µ1 µ2 Delta Receptor Delta1 Delta2 Kappa Receptor K1 K2 K3 Each receptor family exhibits a different specificity for the drug(s) it binds. The analgesic properties of the opioids are primarily mediated by the μ receptors. However, other receptors also contribute. For example, butorphanol and nalbuphine primarily owe their analgesic effect to K-receptor activation. The enkephalins interact more selectively with the Delta receptors in the periphery. All three opioid receptors are members of the G protein coupled receptor family and inhibit adenylyl cyclase. They are also associated with ion channels, increasing postsynaptic K+ efflux (hyperpolarization) or reducing presynaptic Ca2+ influx, thus impeding neuronal firing and transmitter release 2) Endogenous Opioids: Discovered in 1975. These are pentapeptides involved in regulating nociception in the body. These are also called endogenous ligands or specifically endorphins. These are internally derived and binds to opioids receptors. Three families of endogenous opioid peptides have been described: Pro-enkephalin → The pentapeptides o Methionine-enkephalin (met-enkephalin) and o leucine-enkephalin (leu-enkephalin) Selctivity → Delta ↑ & µ receptors The endorphins → Selectivity → both Delta & µ receptors And the dynorphins → Selectivity → ↑ for Kappa receptor The three families of opioid receptors have overlapping affinities for these endogenous peptides derived from three precursor proteins: Prepro-opiomelanocortin (POMC) Preproenkephalin (proenkephalin A) And preprodynorphin (proenkephalin B) Selectivity of Endogenous towards opioids receptors: µ-receptor Delta-receptor kappa-receptor Enkephalin ++ +++ 5 Tahir Muhammad Endorphin +++ +++ Dynorphin A ++ +++ Dynorphin B + + +++ 3) Opioid classification: 1) STRONG AGONIST: Naturally occurring: Phenanthrene derivatives: Morphine → 9-17% Codeine → 0.3-4% Thebaine → 0.2-8 % Benzyl Iso-quinoline derivatives: Papaverine → 1% Noscapine → 6% Semisynthetic: Morphine derivatives: Hydromorphone Oxomorphone Diacetylmorphine (Heroin) Apomorphine Codeine derivatives: Hydrocodone Oxycodone Dihydrocodeine Pholcodeine Synthetic: Phenyl pepiredine derivatives: Fentanyl Alfentanil Sufentanil Remifentanil Pethidine (Meperidine) Diphenoxylate → weakly agonist Loperamide → weakly agonist Phenyl-heptyl-amines: Methadone Propoxyphene Morphinans: Dextromethorphan Levorphanol 6 Tahir Muhammad 2) PARTIAL AGONIST: Nalbuphine Buprenorphine Butorphanol Pentazocine Dezocine Tramadol 3) ANTAGONIST: Naloxone Naltrexone Nalorphine Levallorphan 4) P-K: Route: Parentral → S/C, I/M, P/O Nasal ABSORPTION: Most opioid analgesics are well absorbed However, because of the first-pass effect, the oral dose of the opioid (eg, morphine) may need to be much higher than the parenteral dose to elicit a therapeutic effect. Certain analgesics such as codeine and oxycodone are effective orally because they have reduced first-pass metabolism. Nasal insufflation of certain opioids can result in rapid therapeutic blood levels by avoiding first- pass metabolism. DISTRIBUTION: High Highest concentrations in tissues that are highly perfused such as the brain, lungs, liver, kidneys, and spleen. Drug concentrations in skeletal muscle may be much lower METABOLISM: The opioids are converted mostly to glucuronides. For example, morphine → morphine-3-glucuronide (M3G), a compound with neuroexcitatory properties. → 10% of morphine to morphine-6-glucuronide (M6G), an active metabolite with analgesic potency 4-6 six times that of its parent compound. EXCRETION: Polar metabolites & Small amounts of unchanged → urine. Glucuronide conjugates also found in the bile 5) P-D: 7 Tahir Muhammad ↓ Ca++ influx ↓ the release of neurotransmitters ↑ K+ efflux Opioids exert their major effects by interacting with opioid receptors in the CNS and in other anatomic structures, such as the gastrointestinal tract and the urinary bladder. Opioids cause hyperpolarization of nerve cells, inhibition of nerve firing, and presynaptic inhibition of transmitter release. Morphine acts at µ receptors in Lamina II and III of the dorsal horn of the spinal cord, and it decreases the release of substance P, which modulates pain perception in the spinal cord. Morphine also appears to inhibit the release of many excitatory transmitters from nerve terminals carrying nociceptive (painful) stimuli. 8 Tahir Muhammad 6) Site of Action: Direct action the inflamed peripheral tissue Act at spinal cord level and causes inhibition Effects at supra-spinal level ( Thalamus is involved) Spinal sites of opioid action. 7) Organ system effects: o CNS effects o Peripheral effects A) CNS EFFECTS: The principal effects of opioid analgesics with affinity for µ-receptors are on the CNS; the more important ones include analgesia, euphoria, sedation, and respiratory depression. With repeated use, a high degree of tolerance occurs to all of these effects. 1) Analgesia: Pain consists of both sensory and affective (emotional) components. Opioid analgesics are unique in that they can reduce both aspects of the pain experience, especially the affective aspect. 2) Euphoria: Patients or drug users → i/v morphine → experience a pleasant Oloating sensation with lessened anxiety and distress. 3) Dysphoria: 9 Tahir Muhammad An unpleasant state characterized by restlessness and malaise, may sometimes occur. 4) Sedation: Drowsiness and clouding of mentation are common concomitants of opioid action. There is little or no amnesia. Sleep is induced by opioids more frequently in the elderly than in young, healthy individuals. 5) Respiratory depression: All of the opioid analgesics can produce significant respiratory depression by inhibiting brainstem respiratory mechanisms. Alveolar CO2 may increase, but the most reliable indicator of this depression is a depressed response to a carbon dioxide challenge. The respiratory depression is dose-related. 6) Cough suppression: Suppression of the cough reflex is a well-recognized action of opioids. Codeine in particular has been used in persons suffering from pathologic cough. However, cough suppression by opioids may allow accumulation of secretions and thus lead to airway obstruction and atelectasis. 7) Miosis: Constriction of the pupils is seen with virtually all opioid agonists. Miosis is a pharmacologic action to which little or no tolerance develops, thus, it is valuable in the diagnosis of opioid overdose. Even in highly tolerant addicts, miosis is seen. This action, which can be blocked by opioid antagonists, is mediated by parasympathetic pathways, which, in turn, can be blocked by atropine. 8) Truncal rigidity: An intensification of tone in the large trunk muscles has been noted with a number of opioids. It results from an action at supraspinal levels. Truncal rigidity reduces thoracic compliance and thus interferes with ventilation. The effect is most apparent when high doses of the highly lipid-soluble opioids (eg, fentanyl, Sufentanil, Alfentanil, Remifentanil) are rapidly administered intravenously. 9) Nausea and vomiting: The opioid analgesics can activate the brainstem CTZ to produce nausea and vomiting. 10) Temperature: µ-opioids such as Morphine administered to the anterior hypothalamus produces hyperthermia, whereas administration of k-agonists induce hypothermia. B) PERIPHERAL EFFECTS: 1) Cardiovascular system: Most opioids have no significant direct effects on the heart and, other than bradycardia, no major effects on cardiac rhythm. Meperidine is an exception → antimuscarinic action → result in tachycardia. Blood pressure is usually well maintained in subjects receiving opioids unless the cardiovascular system is stressed, in which case hypotension may occur. This hypotensive effect is probably due to peripheral arterial and venous dilation, which is b/c central depression of vasomotor and release of histamine. No consistent effect on cardiac output is seen, caution should be exercised in patients with decreased blood volume, because will lead to → hypotension. 10 Tahir Muhammad Increased CO2 level leads to cerebral vasodilation associated with a decrease in cerebral vascular resistance, an increase in cerebral blood flow, and an increase in intracranial pressure. 2) Gastrointestinal tract: Constipation has long been recognized as an effect of opioids, an effect that does not diminish with continued use; that is, tolerance does not develop to opioid-induced constipation. Opioid receptors exist in high density in the gastrointestinal tract, and the constipating effects of the opioids are mediated through an action on the enteric nervous system as well as the CNS. Stomach Motility (rhythmic contraction and relaxation) may decrease but tone (persistent contraction) may increase particularly in the central portion; gastric secretion of hydrochloric acid is decreased. Small intestine resting tone is increased, with periodic spasms, but the amplitude of nonpropulsive contractions is markedly decreased. Large intestine propulsive peristaltic waves are diminished and tone is increased; this delays passage of the fecal mass and allows increased absorption of water, which leads to constipation. The large bowel actions are the basis for the use of opioids in the management of diarrhea. 3) Biliary tract: The opioids contract biliary smooth muscle, which can result in biliary colic. The sphincter of Oddi may constrict, resulting in reflux of biliary and pancreatic secretions and elevated plasma amylase and lipase levels. 4) Renal: Renal function is depressed by opioids. It is believed that in humans this is chiefly due to decreased renal plasma flow. In addition, u-agonist opioids have been found to have an antidiuretic effect in humans. Mechanisms may involve both the CNS and peripheral sites. Opioids also enhance renal tubular sodium reabsorption. The role of opioid-induced changes in antidiuretic hormone (ADH) release is controversial. Ureteral and bladder tone ↑ by therapeutic doses of the opioid analgesics. Increased sphincter tone may precipitate urinary retention, especially in postoperative patients. 5) Uterus: The opioid analgesics may prolong labor. The mechanism for this action is unclear, but both peripheral and central actions of the opioids can reduce uterine tone. 6) Endocrine: Opioid analgesics stimulate the release of ADH, prolactin, and somatotropin but inhibit the release of luteinizing hormone. These effects suggest that endogenous opioid peptides, through effects in the hypothalamus, regulate these systems. 7) Pruritis: Therapeutic doses of the opioid analgesics produce flushing and warming of the skin accompanied sometimes by sweating and itching; CNS effects and peripheral histamine release may be responsible for these reactions. 8) Immune system: 11 Tahir Muhammad The opioids modulate the immune system by effects on lymphocyte proliferation, antibody production, and chemotaxis. Natural killer cell cytolytic activity and lymphocyte proliferative responses to mitogens are usually inhibited by opioids. 8) Uses: A) ANALGESIA Severe, constant pain is usually relieved with opioid analgesics with high intrinsic activity whereas sharp, intermittent pain does not appear to be as effectively controlled. Another type of pain control called patient-controlled analgesia (PCA) is now in widespread use for the management of breakthrough pain. With PCA, the patient controls a parenteral (usually intravenous) infusion device by depressing a button to deliver a preprogrammed dose of the desired opioid analgesic. B) ACUTE PULMONARY EDEMA The relief produced by intravenous morphine in dyspnea from pulmonary edema associated with left ventricular failure is remarkable. Proposed mechanisms include reduced anxiety (perception of shortness of breath), and reduced cardiac preload (reduced venous tone) and afterload (decreased peripheral resistance). C) COUGH Suppression of cough can be obtained at doses lower than those needed for analgesia. But now-a- days synthetic cough suppressants are used. D) DIARRHEA Diarrhea from almost any cause can be controlled with the opioid analgesics, but if diarrhea is associated with infection such use must not substitute for appropriate chemotherapy. Crude opium preparations (eg, paregoric) were used in the past to control diarrhea, but now synthetic surrogates with more selective gastrointestinal effects and few or no CNS effects, eg, diphenoxylate, are used. Several preparations are available specifically for this purpose. E) SHIVERING Although all opioid agonists have some propensity to reduce shivering, meperidine is reported to have the most pronounced anti-shivering properties. It is interesting that meperidine apparently blocks shivering through its action on subtypes of the a2 adrenoceptor. F) APPLICATIONS IN ANESTHESIA The opioids are frequently used as premedicant drugs before anesthesia and surgery because of their sedative, anxiolytic, and analgesic properties. They are also used intraoperatively both as adjuncts to other anesthetic agents and, in high doses (eg, 0.02-0.075 mg/kg of fentanyl), as a primary component of the anesthetic regimen. Opioids are most commonly used in cardiovascular surgery and other types of high-risk surgery in which a primary goal is to minimize cardiovascular depression. In such situations, mechanical respiratory assistance must be provided. 9) Toxicities: Behavior restlessness Dysphoria 12 Tahir Muhammad Neuroexcitatory effects Seizures Constipation Postural hypotension Nausea & vomiting Itching around nose Urticaria Tolerance Dependence → Physical → Psychological 10) Contra-indications: 1) Use of pure agonists with weak partial agonists: When a weak partial agonist such as pentazocine is given to a patient also receiving a full agonist (eg, morphine), there is a risk of diminishing analgesia or even inducing a state of withdrawal; combining full agonist with partial agonist opioids should be avoided. 2) Use in patients with head injuries: Carbon dioxide retention caused by respiratory depression results in cerebral vasodilation. In patients with elevated intracranial pressure, this may lead to lethal alterations in brain function. 3) Use during pregnancy: In pregnant women who are chronically using opioids, the fetus may become physically dependent in uterus and manifest withdrawal symptoms in the early postpartum period. A daily dose as small as 6 mg of heroin (or equivalent) taken by the mother can result in a mild withdrawal syndrome in the infant, and twice that much may result in severe signs and symptoms, including irritability, shrill crying, or even seizures. 4) Use in patients with impaired pulmonary function: In patients with borderline respiratory reserve, the depressant properties of the opioid analgesics may lead to acute respiratory failure. 5) Use in patients with impaired hepatic or renal function: Because morphine and its congeners are metabolized primarily in the liver, their use in patients in prehepatic coma may be questioned. Half-life is prolonged in patients with impaired renal function, and morphine and its active glucuronide metabolite may accumulate; dosage can often be reduced in such patients. 6) Use in patients with endocrine disease: Patients with adrenal insufficiency (Addison's disease) and those with hypothyroidism (myxedema) may have prolonged and exaggerated responses to opioids. 11) Drug-interactions: 13 Tahir Muhammad Drug Group Interaction with Opioids Increased central nervous system depression, particularly respiratory Sedative-hypnotics depression. Increased sedation. Variable effects on respiratory depression. Antipsychotic tranquilizers Accentuation of cardiovascular effects (antimuscarinic and a-blocking actions). Relative contraindication to all opioid analgesics because of the high MAO inhibitors incidence of hyperpyrexic coma; hypertension has also been reported. NSAIDS Properties: Antipyretic Analgesic Anti-inflammatory All of the NSAIDs act by inhibiting the synthesis of prostaglandins. These properties of NSAIDS depends upon the inhibition of cyclo-oxygenase enzyme (COX) There are 3 types of COX enzymes COX-1 (Cyclo-oxygenase-1) COX-2 (Cyclo-oxygenase-2) COX-3 (Cyclo-oxygenase-3) These are also called prostaglandins synthetase 1, 2 & 3. Synthesis of prostaglandins: Arachidonic acid, a 20-carbon fatty acid, is the primary precursor of the prostaglandins and related compounds. Arachidonic acid is present as a component of the phospholipids of cell membranes. Cyclooxygenase pathway: i. COX-1: Cyclooxygenase-1 (COX-1) is responsible for the physiologic production of prostanoids, COX-1 is described as a housekeeping enzyme that regulates normal cellular processes, such as gastric cytoprotection, vascular homeostasis, platelet aggregation, and kidney function. ii. COX-2: Cyclooxygenase-2 (COX-2) causes the elevated production of prostanoids that occurs in sites of disease and inflammation. 14 Tahir Muhammad COX-2 is continuously expressed in tissues such as the brain, kidney, and bone. Its expression at other sites is increased during states of inflammation. Lipoxygenase pathway: Alternatively, several lipoxygenases can act on arachidonic acid to form 5-HPETE, 12-HPETE, and 15-HPETE, which are unstable peroxidated derivatives that are converted to the corresponding hydroxylated derivatives (the HETEs) or to leukotrienes or lipoxins, depending on the tissues. (Hydroperoxyeicosatetraenoic and hydroxyeicosatetraenoic acids → HPETEs and HETEs, respectively) 15 Tahir Muhammad Classification of NSAIDS: 1) Classification based on selectivity for COX enzyme 2) Chemical classification: i. Salicylates: o Salicylic acid o Acetyl salicylic acid (Aspirin) o Methyl salicylic acid o Diflunisal ii. Propionic acid (phenyl alkanoic acid)derivatives: o Flurbiprofen o Ibuprofen o Fenoprofen o Ketoprofen o Naproxen 16 Tahir Muhammad o Oxaprozin iii. Indole derivative: o Indomethacin iv. Pyrazolone derivatives: o Phenyl beutazone v. Phenyl acetic acid: o Diclofenac vi. Femanates derivatives: o Mefenamic acid o Flufenamic acid o Meclofenamate vii. Oxicam/ Enolic acid derivatives: o Piroxicam o Meloxicam o Nabumetone viii. Para-aminophenol derivatives: o Acetaminophen ASPIRIN Introduction: Properties: o Analgesic o Anti-pyretic o Anti-inflammatory: Aspirin is the prototype of traditional NSAIDs Officially approved by the FDA in 1939. It is the most commonly used and is the drug to which all other anti-inflammatory agents are compared. It has been replaced by ibuprofen and naproxen, since o they are effective, o available over the counter, o And have good-excellent safety records. P-K: Absorption: After oral administration, the un-ionized salicylates are passively absorbed well & rapidly from stomach & upper part of GIT. Rectal absorption of the salicylates is slow and unreliable, but it is a useful route for administration to vomiting children. Antacids → All types ↓ its absorption b/c they ↓ acidity of the stomach while aspirin is absorbed in acidic environment. 17 Tahir Muhammad Distribution: Well Salicylates (except for Diflunisal) cross both the BBB and the placenta and are absorbed through intact skin (especially methyl salicylate). PPC → 1-2 hours T1/2 → 3-5 hours Displace so many dugs from binding site of protein i.e. Tolbutamide, etc Effect of dose on the half-life of aspirin. Metabolism: Aspirin is metabolized to salicylate and acetic acid by esterases in tissues and blood. Then followed by Oxidation & Conjugation. Excretion: 18 Tahir Muhammad Being an organic acid, salicylate is excreted into the urine and can affect uric acid excretion At low doses of aspirin, uric acid secretion is decreased, whereas at high doses, uric acid secretion is increased. Both hepatic and renal function should be monitored periodically in those receiving long- term, high-dose aspirin therapy. Dosage: Analgesic or antipyretic dose → less than the 0.6-0.65 gram oral dose. The anti-inflammatory dose → for adults is 45 mg/kg/d in divided doses (For children is 50- 75 mg/kg/d in divided doses) For those with RA or osteoarthritis → 3 grams/day Acute myocardial infarction, the dose is 162 to 325 mg of nonenteric coated Pharmalogical Actions: 1) ANTI-INFLAMMATORY EFFECTS Because aspirin inhibits cyclooxygenase activity, it diminishes the formation of prostaglandins and, thus, modulates those aspects of inflammation in which prostaglandins act as mediators. Aspirin inhibits inflammation in arthritis, but it neither arrests the progress of the disease nor induces remission. 2) ANALGESIC EFFECTS: Prostaglandin E2 (PGE2) is thought to sensitize nerve endings to the action of bradykinin, histamine, and other chemical mediators released locally by the inflammatory process. Thus, by decreasing PGE2 synthesis, aspirin and other NSAIDs repress the sensation of pain. The salicylates are used mainly for the management of pain of low to moderate intensity arising from musculoskeletal disorders rather than that arising from the viscera. Combinations of opioids and NSAIDs are effective in treating pain caused by malignancy. Diflunisal is three- to four-fold more potent than aspirin as an analgesic and an anti-inflammatory agent, but it has no antipyretic properties. 3) ANTIPYRETIC EFFECTS: Fever occurs when the set-point of the anterior hypothalamic thermoregulatory center is elevated. This can be caused by PGE2 synthesis, which is stimulated when an endogenous fever-producing agent (pyrogen), such as a cytokine, is released from white cells that are activated by infection, hypersensitivity, malignancy, or inflammation. The salicylates lower body temperature in patients with fever by impeding PGE2 synthesis and release. Aspirin resets the thermostat toward normal, and it rapidly lowers the body temperature of febrile patients by increasing heat dissipation as a result of peripheral vasodilation and sweating. Aspirin has no effect on normal body temperature. Diflunisal does not reduce fever, because it does not cross the blood-brain barrier. 4) ANTI-PLATELET EFFECT: Aspirin irreversibly inhibits platelet COX, so that aspirin's antiplatelet effect lasts 8-10 days. Arachidonic acid 19 Tahir Muhammad Endoperoxides Aspirin Thromboxane-A2 Prostacyclin Aggregation of platelets De-aggregation of platelets & vasoconstriction 5) URICOSURIC EFFECT: In ↓ doses → ↓ uric acid secretion & ↑ tubular absorption → leads to ↑ uric acid in the body. This is mainly due to interference with uric acid secretory enzymes. In ↑ doses (3-4gm/day) → ↑ uric acid secretion ↓ tubular absorption → leads to ↓ uric acid in the body → used in gout. Even small doses of salicylate can block the effects of probenecid and other uricosuric agents that decrease tubular reabsorption of uric acid. Organ system effects: 1) Respiration: Salicylates increase oxygen consumption and CO2 production (especially in skeletal muscle) at full therapeutic doses; these effects are a result of uncoupling oxidative phosphorylation. The increased production of CO2 stimulates respiration (mainly by an increase in depth of respiration with only a slight increase in rate. The increased alveolar ventilation balances → the increased CO2 production, and thus plasma CO2 tension (PCO2) does not change or may decrease slightly 2) Cardiovascular Effects: Low doses of aspirin (3 g daily), as might be given for acute rheumatic fever, salt and water retention can lead to an increase (up to 20%) in circulating plasma volume and decreased hematocrit (via a dilutional effect). There is a tendency for the peripheral vessels to dilate because of a direct effect on vascular smooth muscle. Cardiac output and work are increased. Those with carditis or compromised cardiac function may not have sufficient cardiac reserve to meet the increased demands, and congestive cardiac failure and pulmonary edema can occur. 3) Gastrointestinal Effects: The ingestion of salicylates may result in epigastric distress, nausea, and vomiting. Salicylates also may cause gastric ulceration, exacerbation of peptic ulcer symptoms (heartburn, dyspepsia), gastrointestinal hemorrhage, and erosive gastritis. These effects occur primarily with acetylated salicylates (i.e., aspirin). Because nonacetylated salicylates lack the ability to acetylate cyclooxygenase and thereby irreversibly inhibit its activity, they are weaker inhibitors than aspirin 4) Effects on the Blood: Ingestion of aspirin by healthy individuals prolongs the bleeding time 20 Tahir Muhammad 5) Hepatic Effects: Salicylates can cause hepatic injury, usually in patients treated with high doses of salicylates that result in plasma concentrations of more than 150 µg/ml. The injury is not an acute effect; rather, the onset characteristically occurs after several months of treatment. 6) Actions on the kidney: Cyclooxygenase inhibitors prevent the synthesis of PGE2 and PGI2 prostaglandins that are responsible for maintaining renal blood flow, particularly in the presence of circulating vasoconstrictors. Decreased synthesis of prostaglandins can result in retention of sodium and water and may cause edema and hyperkalemia in some patients. Interstitial nephritis can also occur with all NSAIDs except aspirin Uses: Anti-inflammatory Antipyretic analgesic uses RA → in combo with other drugs. Cardiovascular applications: Aspirin is used to inhibit platelet aggregation. Low doses are used prophylactically to Reduce the risk of ischemic attacks and stroke or death Reduce the risk of death in those having an acute myocardial infarction or unstable angina pectoris. Reduce the cardiovascular risk in patients undergoing certain revascularization procedures. Side effects: Gastrointestinal: Epigastric distress, nausea, and vomiting. Microscopic GI bleeding is almost universal in patients treated with salicylates. Blood: The irreversible acetylation of platelet cyclooxygenase reduces the level of platelet TXA2, resulting in inhibition of platelet aggregation and a prolonged bleeding time. For this reason, aspirin should not be taken for at least 1 week prior to surgery. When salicylates are administered, anticoagulants may have to be given in reduced dosage Respiration: In toxic doses, salicylates cause respiratory depression and a combination of uncompensated respiratory and metabolic acidosis. Metabolic processes: Large doses of salicylates uncouple oxidative phosphorylation. The energy normally used for the production of adenosine triphosphate is dissipated as heat, which explains the hyperthermia caused by salicylates when taken in toxic quantities. Hypersensitivity: Approximately 15 percent of patients taking aspirin experience hypersensitivity reactions. Symptoms of true allergy include urticaria, bronchoconstriction, or angioedema. Fatal anaphylactic shock is rare. 21 Tahir Muhammad Reye's syndrome: Aspirin and other salicylates given during viral infections has been associated with an increased incidence of Reye's syndrome, which is an often fatal, fulminating hepatitis with cerebral edema. This is especially encountered in children, who therefore should be given acetaminophen instead of aspirin when such medication is required to reduce fever. Ibuprofen is also appropriate. Toxicity: Salicylate intoxication is called Salicylism → may be mild or severe Mild cases: Characterized by nausea, vomiting, marked hyperventilation, headache, mental confusion, dizziness, and tinnitus (ringing or roaring in the ears). Treatment → measurement of serum salicylate concentrations and of pH to determine the best form of therapy. In mild cases, symptomatic treatment is usually sufficient. Increasing the urinary pH enhances the elimination of salicylate. In serious cases: When large doses of salicylate are administered, severe salicylate intoxication may result. The symptoms listed above are followed by restlessness, delirium, hallucinations, convulsions, coma, respiratory and metabolic acidosis, and death from respiratory failure Treatment → Mandatory measures include the intravenous administration of fluid, dialysis (hemodialysis or peritoneal dialysis), and the frequent assessment and correction of acid-base and electrolyte balances. [Note: Diflunisal does not cause salicylism.] Dose dependent effects of salicylate. Drug Interactions: The plasma concentration of salicylates generally is little affected by other drugs, but concurrent administration of aspirin lowers the concentrations of indomethacin, naproxen, Ketoprofen, and Fenoprofen, at least in part by displacement from plasma proteins. 22 Tahir Muhammad Aspirin could displace other highly protein-bound drugs, such as warfarin, phenytoin, or valproic acid, resulting in higher free concentrations of the other agent → Lead to toxicity of these agents. Other interactions of aspirin include the antagonism of spironolactone-induced natriuresis. Blockade of the active transport of penicillin from CSF to blood. Contra-Indications: Aspirin is classified as FDA pregnancy category C risk during Trimesters 1 and 2 and category D during Trimester 3. Because salicylates are excreted in breast milk, aspirin should be avoided during pregnancy and while breast-feeding Aspirin Resistance All forms of treatment failure with aspirin have been collectively called "aspirin resistance." Genetic variants of COX-1 that cosegregate with resistance have been described, but the relation to clinical outcome is not clear. PARACETAMOL Introduction: Also called Acetaminophen/N-acetyl-p-aminophenol. It is the active metabolite of phenacetin / also called coal tar analgesic. Acetaminophen is an effective alternative to aspirin as an analgesic-antipyretic agent. however, its anti-inflammatory effects are much weaker Acetaminophen is well tolerated and has a low incidence of gastrointestinal side effects. It is available without a prescription and is used as a common household analgesic like aspirin. It was introduced into market in 1886 under the name antifebrin by Cahn and Hepp, who had discovered its antipyretic action accidentally. P-K: Absorption: Acetaminophen is administered orally. Rapidly & well. Absorption is related to the rate of gastric emptying. Distribution: Uniformly distributed throughout most body fluids PPC → 30-60 mins 23 Tahir Muhammad T1/2 → 2 hours Acetaminophen is slightly bound to plasma proteins → less than other NSAIDs. Metabolism: Partially metabolized by hepatic microsomal enzymes. Hepatic conjugation with glucuronic acid (about 60%), sulfuric acid (about 35%), or cysteine (about 3%). Small proportion of acetaminophen undergoes N-hydroxylation to form N-acetyl-p- benzoquinoneimine (NAPQI), a highly reactive intermediate. This metabolite normally reacts with sulfhydryl groups in glutathione (GSH) and thereby is rendered harmless. However, after ingestion of large doses of acetaminophen, the metabolite is formed in amounts sufficient to deplete hepatic GSH and contributes significantly to the toxic effects of overdose. Excretion: Renal pathway Pharmalogical Actions: Acetaminophen has analgesic and antipyretic effects similar to those of aspirin. However, it has only weak anti-inflammatory effects and has been thought to have a generally poor ability to inhibit COX in the presence of high concentrations of peroxides as are found at sites of inflammation. Daily dose, 1000 mg, results in roughly 50% inhibition of both COX-1 and COX-2 in whole blood in healthy volunteers. Recent evidence suggests that acetaminophen may inhibit a third enzyme, COX-3, in the central nervous system. COX-3 appears to be a splice variant product of the COX-1 gene. No CNS, platelets, & uricosuric effects. No effects on acid-base balance ↓ GIT upset Uses: Although equivalent to aspirin as an effective analgesic and antipyretic agent, acetaminophen differs in that it lacks anti-inflammatory properties. The drug is useful in mild to moderate pain such as headache, myalgia, postpartum pain, and other circumstances in which aspirin is an effective analgesic. For mild analgesia, acetaminophen is the preferred drug in patients allergic to aspirin or when salicylates are poorly tolerated. It is preferable to aspirin in patients with hemophilia or a history of peptic ulcer and in those in whom bronchospasm is precipitated by aspirin. 24 Tahir Muhammad Unlike aspirin, acetaminophen does not antagonize the effects of uricosuric agents; it may be used concomitantly with probenecid in the treatment of gout. It is preferred to aspirin in children with viral infections Dosage: Acute pain and fever may be effectively treated with 325-500 mg four times daily and proportionately less for children. Side effects: Mild doses: In therapeutic doses, a mild increase in hepatic enzymes may occasionally occur in the absence of jaundice; this is reversible when the drug is withdrawn. Larger doses: CNS → dizziness, excitement, and disorientation are seen. Hepatotoxicity → Ingestion of 15 g of acetaminophen may be fatal, death being caused by severe hepatotoxicity with centrilobular necrosis. Early symptoms of hepatic damage include nausea, vomiting, diarrhea, and abdominal pain. Nephrotoxicity → acute renal tubular necrosis → Cases of renal damage without hepatic damage have occurred, even after usual doses of acetaminophen. Blood → Hemolytic anemia and methemoglobinemia are very rare. Contra-Indications: Alcoholism → Given with Cautions Any type of liver disease. Overdose treatment: Antidote → N-acetylcysteine (NAC) which functions by detoxifying NAPQI. It is administered orally within 8-10 hours after overdosing. NAC form complex with NAPQI (Contains sulfhydryl group to which the toxic metabolite can bind) → excreted. Can also be removed from the body by hemodialysis or peritoneal dialysis. When damage occurred to the liver → Liver transplantation should be done. GENERAL ANESTHESIA Anesthesia is of two types; General Local GENERAL ANESTHESIA: “Induction of a state of unconsciousness with the absence of pain sensation over the entire body”. So generally anesthesia having two main effects which are; Unconsciousness Inhibition of pain sensation The physiologic states of general anesthesia typically includes analgesia, amnesia, loss of consciousness, inhibition of sensory and autonomic reflexes, and skeletal muscle relaxation. The extent to which any individual anesthetic drug can exert these effects depends upon, 25 Tahir Muhammad the drug the dosage & the clinical situation. PURPOSE OF G.ANESTHESIA: To produce unconsciousness To relief pain → Analgesia Blocking of memory of the procedure → called amnesia. Inhibiting of the normal reflexes → to make the surgery safe. Relaxing the skeletal muscles. PROPERTIES OF A GOOD ANESTHETIC: An ideal anesthetic drug would induce anesthesia smoothly and rapidly. Prompt/Quick recovery after its administration is discontinued. The drug would also possess a wide margin of safety and be devoid of adverse effects. Should produce complete relaxation of the skeletal muscles. Should be stable and non-inflammable. No single anesthetic agent is capable of achieving all of these desirable effects without some disadvantages when used alone, so thas why combo of inhaled & i/v anesthetics are used to obtain these desirable properties. STAGES OF ANESTHESIA: There are mainly 4 stages of anesthesia, all of which can’t be seen because of proper selection and combo of anesthetics. Stage-1: Stage of analgesia Patient feels initially analgesia which is followed by amnesia. Starts from the beginning of anesthesia and lasts Upto the loss of consciousness. Normal reflexes are there Patient can conversant with the fellow and can hear. Analgesic effects are produced b/c of inhibition sensory transmission in the spinothalamic tract in the dorsal horn of spinal cord. Stage-2: Stage of excitement and delirium Onset of unconsciousness. During this stage, the patient often appears to be delirious and excited but definitely is amnesic. Respiration is irregular both in volume and rate, and retching and vomiting may occur. For these reasons, efforts are made to limit the duration and severity of this stage, which ends with their establishment of regular breathing. Cortical inhibitory centers are depressed before than the excitatory center i.e; o Blockade of inhibitory o Release of excitatory neurotransmitters o The results will be; 26 Tahir Muhammad High reflexes of tone High autonomic activities More reflex vomiting High respiratory pneumonia High cardiac arrhythmia (vagal inhibition) So thas why in modern anesthesia this stage is bypassed by the use short acting barbiturates. Stage-3: Stage of surgical anesthesia This stage begins with the recurrence of regular respiration and extends to complete cessation of spontaneous respiration. Four planes of stage III have been described in terms of changes in ocular movements, eye reflexes, and pupil size,& respiration, which under specified conditions may represent signs of increasing depth of anesthesia. o Plane-1: Normal respiration Constricted pupil Roving/Pendular movement of the eyeball (b/c of effects on medulla) Reflexes are abolished except corneal reflex o Plane-2: Respiration become shallow Pupil size begin to dilate Eyeballs become fixed Loss of corneal reflex No ocular movement Skeletal muscles are relaxed o Plane-3: Respiration further depressed Pupil size further dilated Skeletal muscles are further relaxed B.P decreased All reflexes are absent o Plane-4: Medullary center begins to depress Intercostal muscles paralyzed Respiration shifted to abdominal muscles i.e further shallowed B.P falls & H.R increase (reflex T.Cardia) Pupil widely dilated All reflexes are absent Dangerous plane. Stage-4: Stage of medullary depression. This stage is never achieved otherwise will lead to death b/c of cessation of breathing and failure of circulation. 27 Tahir Muhammad Medullary neurons are depressed b/c of high dose of anesthetic. This stage occurs when center for breathing and other functions i.e Medulla cease to function. So this stage should never be reached and careful amount of anesthesia should be administered to prevent this stage. CLASSIFICATION OF GENERAL ANESTHETICS Three types of drugs are used in general anesthesia which are; Pre-anesthetic drugs For induction For maintenance A) PRE-ANESTHETIC DRUGS: 1. Anti-cholinergic & H2 antagonists: For the prevention of secretions. 2. Benzodiazepines: For sedation and anxiolytic purpose. 3. Opioids: For pain. 4. Muscle relaxants: For muscle relaxation e.g Suxamethonium. B) DRUGS FOR THE INDUCTION OF ANESTHESIA: Thiopental-Na Propofol paraldehyde C) DRUGS FOR THE MAINTAINANCE OF ANESTHESIA: For this purpose combo of inhalant & i/v anesthetics are used b/c none of this alone can maintain well anesthesia. 1. Inhaled anesthetics: a) Gases: These are gases at room temp Nitrous oxide cyclopropane b) Volatile liquids: Halogenated agents; Halothane Isoflurane Enflurane Sevaflurane Desflurane Methoxyflurane Chloroform 28 Tahir Muhammad Trichloroethylene Ethers; Diethyl ether Divinyl ether 2. I/v anesthetics: a) Barbiturates: Thiopental-Na Methohexital b) BDZ: Diazepam Midazolam Lorazepam c) Opioids: Opium Fentanyl Sofentanil Alfentanil Remifentanil Propofol d) Miscellaneous: Ketamine ANESTHETICS: HOW THEY WORK Type Name(s) Administered Affect General Halothane, Intravenously, Produces total Enflurane Inhalation unconsciousness Isoflurane, affecting the entire Ketamine, body Nitrous Oxide, Thiopental Regional Mepivacaine, Intravenously Temporarily inter- Chloroprocaine, rupts transmission Lidocaine of nerve impulses (temperature, touch, pain) and motor functions in a large area to be treated; does not 29 Tahir Muhammad produce unconsciousness Local Procaine, Intravenously Temporarily blocks Lidocaine, transmission of Tetracaine, nerve impulses and Bupivacaine motor functions in a specific area; does not produce unconsciousness Topical Benzocaine Demal Temporarily blocks Lidocaine (Sprays, nerve endings in Tetracaine Drope, skin and mucous Ointments, membranes; does Creams, Gels) not produce unconsciousness P.k PROPERTIES OF INHALED ANESTHETICS: These properties depend upon the following factors; Solubility or partition coefficient of anesthetic. Pulmonary ventilation rate. Pulmonary blood flow. Its concentration in the inspired air. 30 Tahir Muhammad Tensions of three anesthetic gases in arterial blood as a function of time after beginning inhalation. Nitrous oxide is relatively insoluble (blood: gas partition coefficient = 0.47); methoxyflurane is much more soluble (coefficient = 12); and halothane is intermediate (2.3). 1) Solubility of anesthetic: The blood-gas partition coefficient is a useful index of solubility and defines the relative affinity of an anesthetic for the blood compared to air. “Partition coefficient is the ratio of concentration of anesthetics in blood to the concentration in gas”. Partition coefficient = Anesthetic conc. in blood Anesthetic conc. in gas NO2 ↓ P.coefOicient ↓ solubility in blood ↑ P.Pressure ↑ induction. Halothane ↑ P.coefOicient ↑ solubility in blood ↓ P.Pressure ↓ induction. Methoxyflurane ↑↑ P.coefOicient ↑↑ solubility in blood ↓↓ P.Pressure ↓↓ induction. The partition coefficients for desflurane and nitrous oxide, which are relatively insoluble in blood, are extremely low. When an anesthetic with low blood solubility diffuses from the lung into the arterial blood, relatively few molecules are required to raise its partial pressure, and therefore the arterial tension rises rapidly. There is inverse relation b/w Solubility(P.Coefficient) and P.Pressure And Direct relation b/w P.Pressure & Induction. Why induction of anesthesia is slower with more soluble anesthetic gases. In this schematic diagram, solubility in blood is represented by the relative size of the blood compartment (the more soluble, the larger the compartment). Relative partial pressures of the agents in the compartments are indicated by the degree of filling of each compartment. For a given concentration or partial pressure of the two anesthetic gases in the inspired air, it will take much longer for the blood partial pressure of the more soluble gas (halothane) to rise to the same partial pressure as in the alveoli. Since the concentration of 31 Tahir Muhammad the anesthetic agent in the brain can rise no faster than the concentration in the blood, the onset of anesthesia will be slower with halothane than with nitrous oxide. 2) Pulmonary ventilation rate: The rate of rise of anesthetic gas tension in arterial blood is directly dependent on both the rate and depth of ventilation (i.e, minute ventilation). The magnitude of the effect varies according to the blood: gas partition coefficient. An increase in pulmonary ventilation is accompanied by only a slight increase in arterial tension of an anesthetic with low blood solubility or low coefficient but can significantly increase tension of agents with moderate or high blood solubility (Figure below). For example, a fourfold increase in ventilation rate almost doubles the arterial tension of halothane during the first 10 minutes of anesthesia but increases the arterial tension of nitrous oxide by only 15%. Therefore, hyperventilation increases the speed of induction of anesthesia with inhaled anesthetics that would normally have a slow onset. Depression of respiration by opioid analgesics will slow the onset of anesthesia of some inhaled anesthetics if ventilation is not assisted. Ventilation rate and arterial anesthetic tensions. Increased ventilation (8 versus 2 L/min) has a much greater effect on equilibration of halothane than nitrous oxide. 3) Pulmonary blood flow: Changes in blood flow to and from the lungs influence transfer processes of the anesthetic gases. 32 Tahir Muhammad An increase in pulmonary blood flow (increased cardiac output) slows the rate of rise in arterial tension, particularly for those anesthetics with moderate to high blood solubility. This is because increased pulmonary blood flow exposes a larger volume of blood to the anesthetic; thus, blood "capacity" increases and the anesthetic tension rises slowly. A decrease in pulmonary blood flow has the opposite effect and increases the rate of rise of arterial tension of inhaled anesthetics in a patient with circulatory shock, the combined effects of decreased cardiac output (resulting in decreased pulmonary flow) and increased ventilation will accelerate the induction of anesthesia with halothane and isoflurane. This is not likely to occur with nitrous oxide, desflurane, or sevoflurane because of their low blood solubility. 4) Conc. in inspired air: Increases in the inspired anesthetic concentration will increase the rate of induction of anesthesia by increasing the rate of transfer into the blood according to Fick's law. That is, simply if the concentration in the inspired air is more/ high then rapid will be the onset of anesthesia and vice versa. The concentration of an individual gas in a mixture of gases is proportionate to its partial pressure or tension. These terms are often used interchangeably in discussing the various transfer processes of anesthetic gases in the body. Achievement of a brain concentration of an inhaled anesthetic adequate to cause anesthesia requires transfer of that anesthetic from the alveolar air to blood and then to brain. The rate at which a given concentration of anesthetic in the brain is reached depends on the solubility properties of the anesthetic, its concentration in the inspired air, pulmonary ventilation rate, pulmonary blood flow, and the partial pressure gradient of the anesthetic between arterial and mixed venous blood. Similarly Cerebral blood flow ↑ → Quick onset & recovery. Alveolar exchange ↑ → Quick onset & recovery, and vice versa. 5) Recovery: The time to recovery from inhalation anesthesia depends on the rate of elimination of anesthetics from the brain after the inspired concentration of anesthetic has been decreased. Many of the processes of anesthetic transfer during recovery are similar to those that occur during induction of anesthesia. The blood: gas partition coefficient/ solubility of the anesthetic agent. The pulmonary blood flow, the magnitude of ventilation. Duration of administration of anesthesia also prolong the anesthesia. Accumulation of anesthetics in skeletal muscles, brain, adipose tissues etc If exposure is low, rapid will be the recovery and vice versa. Alveolar exchange etc. 6) Elimination: Inhaled anesthetics that are relatively insoluble in blood (low blood: gas partition coefficient) and brain are eliminated at faster rates than more soluble anesthetics. Clearance of inhaled anesthetics by the lungs into the expired air is the major route of their elimination from the body. However, metabolism by enzymes of the liver and other tissues ma also contribute to the elimination of volatile anesthetics. For example, the elimination of halothane 33 Tahir Muhammad during recovery is more rapid than that of enflurane, which would not be predicted from their respective solubilities. MECHANISM OF ACTION OF G.ANESTHETICS: The inhaled anesthetics, and most of the intravenous agents depress spontaneous and evoked activity of neurons in many regions of the brain. There are two types of mechanisms: Cellular mechanism: Molecular mechanism: A) CELLULAR MECHANISM Older concepts of the mechanism of anesthesia, evoked by nonspecific interactions of these agents with the lipid matrix of the nerve membrane (the Meyer-Overton principle) interactions that were thought to lead to secondary changes in ion flux. Recently, evidence has accumulated, suggesting that the modification of ion currents by anesthetics results from more specific interactions with nerve membrane components. The ionic mechanisms involved for different anesthetics may vary, but at clinically relevant concentrations they appear to involve interactions with members of the ligand-gated ion channel family. General anesthetics produce two important physiologic effects at the cellular level. First, the inhalational anesthetics can hyperpolarize neurons. Second, at anesthetizing concentrations, both have substantial effects on synaptic transmission and much smaller effects on action-potential generation or propagation. B) MOLECULAR MECHANISM: It is the more recent concept. There is strong evidence that ligand-gated ion channels are important targets for anesthetic action. Chloride channels gated by the inhibitory GABAA receptors (GABAA receptor-chloride channel) are sensitive to clinical concentrations of a wide variety of anesthetics. Halogenated inhalational agents and many intravenous agents such a Propofol, barbiturates, etomidate, and neurosteroids acts through this mechanism. Anesthetic molecules do not interact directly with the GABA binding site but with specific sites in the transmembrane domains of both α and β subunits. This causes a prolongation of the inhibitory chloride ion current after a pulse of GABA release. Postsynaptic neuronal excitability is thus diminished. C) OTHER MECHANISMS: 34 Tahir Muhammad Inhaled anesthetics have been reported to cause membrane hyperpolarization (an inhibitory action) via their activation of ligand-gated potassium channels. These channels are ubiquitous in the central nervous system and are linked to several neurotransmitters, including acetylcholine, dopamine, norepinephrine, and serotonin. Ketamine does not produce its effects via facilitation of GABAA receptor functions, but it may function via antagonism of the action of the excitatory neurotransmitter, glutamic acid on the NMDA receptor. ORGAN SYSTEM EFFECTS: 1) CVS: The most prominent physiological effect of anesthesia induction is a decrease in systemic arterial blood pressure. The causes includes, Direct vasodilatation, Myocardial depression, A blunting of baroreceptor control, And a generalized decrease in central sympathetic tone. Heart effects: ↓ H.R ↓ CO this is due to alteration of sinus node activity and blunting of baroreceptor control. With halothane and enflurane, the reduced arterial pressure appears to be caused by a reduction in cardiac output…… Peripheral resistance: This is due to shift of the autonomic balance, i.e ↑ p. sympathetic activity through vagal tone shifting and ↓ sympathetic activity. Isoflurane, desflurane, and sevoflurane have a depressant effect on arterial pressure as a result of a decrease in systemic vascular resistance with minimal effect on cardiac output. So in general Halothane and enflurane has got ↑ CVS depressant activity than isoflurane, desflurane and sevoflurane etc. Nitrous oxide also depresses CVS activity but upto a limited extent. Certain factors influence the CVS effects are; → surgical stimulus → volume status → duration status → ventilating status With the use of anesthetic CO2 level ↑ (called Hypercapnia) → causes ↑ release of the catecholamines which in turn ↑ B.P. Halothane upto a lesser extent sensitize the myocardial tissues towards catecholamine → ↑ effect will there on the heart → ↑ B.P. 2) RESPIRATORY SYSTEM: With the exception of nitrous oxide, all inhaled anesthetics in current use cause a dose dependent decrease in tidal volume and an increase in respiratory rate. However, the increase in rate is insufficient to compensate for the decrease in volume, resulting, a decrease in minute ventilation. 35 Tahir Muhammad All inhaled anesthetics are respiratory depressants, as indicated by a reduced response to increased levels of carbon dioxide. Inhaled anesthetics increase the apneic level for the CO2 below which apnea occurs (through lack of CO2-driven respiratory stimulation) and decrease the ventilatory response to hypoxia. Depression is vary depending on the volatile nature of the anesthetic. To prevent respiratory depression, mechanical ventilation is used. Inhaled anesthetics also depress mucociliary function in the airway. Thus, prolonged anesthesia may lead to pooling of mucus and then result in atelectasis and postoperative respiratory infections. However, inhaled anesthetics tend to be bronchodilators, an effect of value in the treatment of status asthmaticus. The bronchodilating action of halothane and sevoflurane makes them the induction agents of choice in patients with underlying airway problems. Airway irritation, which may provoke coughing or breath holding, is rarely a problem with most inhaled anesthetics (especially with desflurane). 3) KIDNEY: Inhaled anesthetics decrease glomerular filtration rate and effective renal plasma flow and increase filtration fraction. All anesthetics tend to increase renal vascular resistance. 4) LIVER: All volatile anesthetics cause a decrease in hepatic blood flow, ranging from 15% to 45% of the preanesthetic flow rate. Halothane causes hepatotoxicity 5) BRAIN: Inhaled anesthetics decrease the metabolic rate of the brain. Nevertheless, most volatile agents increase cerebral blood flow because they decrease cerebral vascular resistance. The increase in cerebral blood flow is often clinically undesirable. For example, in patients who have an increased intracranial pressure because of a brain tumor or head injury, administration of a volatile anesthetic may increase cerebral blood flow, which in turn will increase cerebral blood volume and further increase intracranial pressure. Of the inhaled anesthetics, nitrous oxide increases cerebral blood flow the least. 6) REPRODUCTIVE SYSTEM: Causes abortion. The risk of abortion is clearly higher following this experience. It is not obvious whether the underlying disease, surgery, anesthesia, or a combination of these factors is the cause of the increased risk TOXICITY: 1) HEPATOTOXICITY: Hepatotoxicity mainly occurs with halothane. Postoperative hepatic dysfunction is usually associated with factors such as blood transfusions, hypovolemic shock, and other surgical stresses rather than anesthetic toxicity. 36 Tahir Muhammad The mechanisms underlying hepatotoxicity from halothane remain unclear, but studies in animals have implicated the formation of reactive metabolites that either cause direct hepatocellular damage (e.g, free radical intermediates) or initiate immune-mediated responses. 2) NEPHROTOXICITY: Metabolism of enflurane and sevoflurane leads to the formation of fluoride ions which causes the Nephrotoxicity. 3) MALIGNANT HYPERTHERMIA: Malignant hyperthermia is an autosomal dominant genetic disorder of skeletal muscle that occurs in susceptible individuals undergoing general anesthesia with inhaled agents and muscle relaxants characterized by, o Rapid onset of tachycardia and hypertension, o Severe muscle rigidity, o Hyperthermia, o Hyperkalemia, o And acid-base imbalance with acidosis, following exposure to a triggering agent. Malignant hyperthermia is a rare but important cause of anesthetic morbidity and mortality. The specific biochemical abnormality is an increase in free calcium concentration in skeletal muscle cells. Treatment includes administration of dantrolene (which prevents calcium release from the sarcoplasmic reticulum) and appropriate measures to reduce body temperature and restore electrolyte and acid-base balance. 4) HEMATOTOXICITY: Prolonged exposure to nitrous oxide decreases methionine synthase activity and causes megaloblastic anemia. Under normal conditions, most modern and many older inhaled anesthetics are neither mutagens nor carcinogens. MAC-Measurement Of Anesthetic Potency (Min Alveolar Conc.) For inhalational anesthetics, anesthetic potency is measured in MAC units, with 1 MAC defined as the minimum alveolar concentration that prevents movement in response to surgical stimulation in 50% of subjects. The potency of intravenous agents is defined as the free plasma concentration (at equilibrium) that produces loss of response to surgical incision (or other end points) in 50% of subjects HALOTHANE 1) INTRODUCTION: 37 Tahir Muhammad This agent is the prototype Halothane (FLUOTHANE) is a volatile liquid at room temperature. Because halothane is light-sensitive and subject to spontaneous breakdown, it is marketed in amber bottles with thymol added as a preservative. When halothane was introduced, its ability to induce the anesthetic state rapidly and to allow quick recovery and the fact that it was nonexplosive made it an anesthetic of choice. 2) P.K: Halothane has a relatively high blood:gas partition coefficient and high fat:blood partition coefficient. Induction with halothane therefore is relatively slow. Because halothane is soluble in fat and other body tissues, it will accumulate during prolonged administration. Therefore, the speed of recovery from halothane is lengthened as a function of duration of administration. Trifluoroacetic acid, bromine, and chlorine all can be detected in the urine. Trifluoroacetylchloride, an intermediate in oxidative metabolism of halothane, can trifluoroacetylate several proteins in the liver. An immune reaction to these altered proteins may be responsible for the rare cases of fulminant halothane-induced hepatic necrosis Approximately 60% to 80% of halothane taken up by the body is eliminated unchanged via the lungs in the first 24 hours after its administration → approximately 1% of halothane metabolized. 3) CLINICAL USE: Halothane, introduced in 1956, was the first of the modern, halogenated inhalational anesthetics used in clinical practice used for maintenance of anesthesia. It is not pungent and is therefore well tolerated for inhalation induction of anesthesia. This is most commonly done in children, in whom preoperative placement of an intravenous catheter can be difficult. Replaced by newer agents → newer inhalational agents with better pharmacokinetic and side- effect profiles. 4) SIDE EFFEDTS: Cardiovascular System. Respiratory System. Malignant hyperthermia: Hepatotoxicity. Isoflurane Low volatility Can’t be used for induction. This halogenated anesthetic is widely used. 38 Tahir Muhammad It is a very stable molecule that undergoes little metabolism; as a result, little fluoride is produced. Isoflurane is not tissue toxic. Unlike the other halogenated anesthetic gases, isoflurane does not induce cardiac arrhythmias and does not sensitize the heart to the action of catecholamines. However, it produces concentration-dependent hypotension due to peripheral vasodilation. It also dilates the coronary vasculature, increasing coronary blood flow and oxygen consumption by the myocardium. This property may make it beneficial in patients with ischemic heart disease. Sevoflurane Sevoflurane has low pungency, allowing rapid uptake without irritating the airway during induction, thus making it suitable for induction in children. It has replaced halothane for this purpose. Smooth induction. The drug has low solubility in blood and is rapidly taken up and excreted. Recovery is faster than with other anesthetics. It is metabolized by the liver, releasing fluoride ions; thus, like enflurane, it may prove to be nephrotoxic. LOCAL ANESTHETICS DEFINITION: “Local anesthetic blocks reversibly the conduction of sodium along the nerve axons or other excitable membrane, utilizing the sodium channel as a primary mean of potential generation (impulse generation).” Local anesthetics bind reversibly to a specific receptor site within the pore of the Na+ channels in nerves and block ion movement through this pore. HISTORY: Cocaine, the first such agent, was isolated by Niemann in 1860. It was introduced into clinical use by Koller in 1884 as an ophthalmic anesthetic. Cocaine was soon found to be strongly addicting but was widely used, for 30 years, since it was the only local anesthetic drug available. In an attempt to improve the properties of cocaine, Einhorn in 1905 synthesized procaine, which became the dominant local anesthetic for the next 50 years. Since 1905, many local anesthetic agents have been synthesized. The goals of these efforts were Reduction of local irritation and tissue damage, Minimization of systemic toxicity, Faster onset of action, And longer duration of action. Lidocaine, still a popular agent, was synthesized in 1943 by Löfgren and may be considered the prototype local anesthetic agent. PROPERTIES OF A GOOD L.ANESTHETIC: None of the currently available local anesthetics are ideal, and development of newer agents is still continued. 39 Tahir Muhammad However, while it is relatively easy to synthesize a chemical with local anesthetic effects, it is very difficult to reduce the toxicity significantly below that of the current agents. The major reason for this difficulty is the fact that the much of the serious toxicity of local anesthetics represents extensions of the therapeutic effect on the brain and the circulatory system. Less/ No irritation. Long duration of action. Rapid onset of action. Should have no systemic toxicity. CHEMICAL STRUCTURE: Most local anesthetic agents consist of a, Aromatic ring → lipophilic Ester or amide linkage (connects lipophilic & hydrophilic parts) An ionizable group( usually tertiary amine) → hydrophilic Lipophilic group Ester/amide Hydrophilic group In addition to the general physical properties of the molecules, specific stereochemical configurations are associated with differences in the potency of stereoisomers for a few compounds, e.g, bupivacaine, ropivacaine. Since ester links (as in procaine) are more prone to hydrolysis than amide links, esters usually have a shorter duration of action. CLASSIFICATION: A) BASED ON CHEMISTRY: I. Ester derivatives: Cocaine Procaine Tetracaine Benzocaine II. Amide derivatives: Lidocaine Prilocaine Bupivacaine Mepivacaine Etidocaine B) BASED ON DURATION/ONSET OF ACTION: I. Short acting: Procaine → 60-90mins (duration) → slow (onset) Chlorprocaine → 30-60 mins → rapid II. Intermediate acting: Mepivacaine → 120-240mins → fast Prilocaine → 120-240mins → fast Lidocaine → 90-200mins → fast 40 Tahir Muhammad III. Long acting: Tetracaine → 180-600mins → slow Bupivacaine → 180-600mins → intermediate Etidocaine → 180-600mins → fast The onset of local anesthesia can be accelerated by the use of solutions saturated with carbon dioxide ("carbonated"). The high tissue level of CO2 results in intracellular acidosis (CO2 crosses membranes readily), which in turn results in intracellular accumulation of the cationic form of the local anesthetic. The anesthetic effect of the agents with short and intermediate durations of action can be prolonged by increasing the dose or by adding a vasoconstrictor agent (eg, epinephrine or phenylephrine). MECHANISM: All local anesthetics are membrane stabilizer; they reversibly decrease the depolarization and repolarization of excitable membranes. Block the initiation & propagation of action potential by preventing the voltage dependent increase in Na+ conductance. Local anesthetic activity is also highly effected by pH, b/c of basic nature & non-ionize form (local anesthetic high pH ie basic pH) → ↑ lipid soluble → cross axonal membrane → block the sodium channel by combining to the inner side of membrane. Local anesthetics may also block nicotinic channels in the spinal cord. Acidosis such as caused by inflammation partly reduces action of local anesthetic. This is because most anesthetics are ionized & therefore unable to cross the membrane to reach to its site of action. FACTORS EFFECTING LOCAL ANESTHETICS: Hydrophobicity increases both the potency and the duration of action of the local anesthetics because association of the drug at hydrophobic sites enhances the partitioning of the drug to its sites of action and decreases the rate of metabolism by plasma esterases and hepatic enzymes. Hydrophobicity also increases toxicity, so that the therapeutic index is decreased for more hydrophobic drugs. Molecular size influences the rate of dissociation of local anesthetics from their receptor sites. Smaller drug molecules can escape from the receptor site more rapidly. This characteristic is important in rapidly firing cells, in which local anesthetics bind during action potentials and dissociate during the period of membrane repolarization. Rapid binding of local anesthetics during action potentials causes the frequency- and voltage-dependence of their action. ADVERSE EFFECTS: 1. CNS: Following absorption, local anesthetics may cause, CNS) stimulation → due to suppression of inhibitory neurons, Is followed by depression; death usually is caused by respiratory failure. Airway control and ventilatory support are essential features of treatment in the late stage of intoxication. Producing restlessness and tremor → that may progress to clonic convulsions. In general ↑ potent the anesthetic → ↑ convulsions may be produced. 41 Tahir Muhammad Drowsiness is the most frequent complaint that results from the CNS actions of local anesthetics, Lidocaine may produce dysphoria or euphoria and muscle twitching. Both Lidocaine + procaine → loss of consciousness that is preceded only by symptoms of sedation. Cocaine has a particularly prominent effect on mood and behavior. Benzodiazepines or rapidly acting barbiturates administered intravenously are the drugs of choice for both the prevention and arrest of convulsions. Alterations of CNS activity are thus predictable from the local anesthetic agent in question and the blood concentration achieved 2. CVS: Myocardial depression → Due to Na+ channel blockade → ↓ intracellular Ca+ level → ↓ myocardial muscles activity. Arteriolar dilation → o Direct vasodilator action on B.vessels. o ↓ Sympathetic system activity → vasodilation. Leading to severe ↓ in BP which is sudden & life threatening. Local anesthetics decrease electrical excitability, conduction rate, and force of contraction. Untoward cardiovascular effects usually are seen only after high systemic concentrations are attained and effects on the CNS are produced. Lower doses of some local anesthetics will cause cardiovascular collapse and death, probably due to either an action on the pacemaker or the sudden onset of ventricular fibrillation. Ventricular tachycardia and fibrillation are relatively uncommon. 3. NEUROTOXICITY: When applied at excessively high concentrations, all local anesthetics can be toxic to nerve tissue. Chlorprocaine and Lidocaine appear to be more neurotoxic than other local anesthetics when used for spinal anesthesia, producing so-called transient radicular irritation. It has been suggested that this toxicity results from pooling of high concentrations of the local anesthetic in the cauda equina. Although the mechanism of this neurotoxic action has not been established, both interference with axonal transport and disruption of calcium homeostasis have been shown to occur and could be responsible. Spinal neurotoxicity does not result from excessive sodium channel blockade. 4. HEMATOLOGIC EFFECTS: The administration of large doses (> 10 mg/kg) of Prilocaine during regional anesthesia may lead to, Accumulation of the metabolite o-toluidine, an oxidizing agent capable of converting hemoglobin to methemoglobin → methemoglobinemia. The treatment of methemoglobinemia involves the intravenous administration of reducing agents (eg, methylene blue or ascorbic acid), which rapidly convert methemoglobin to hemoglobin. 5. HYPERSENSITIVITY: Rare individuals are hypersensitive to local anesthetics, Displaying allergic dermatitis or A typical asthmatic attack. It is important to distinguish allergic reactions from toxic side effects and from effects of coadministered vasoconstrictors. 42 Tahir Muhammad Hypersensitivity seems to occur more frequently with local anesthetics of the ester type and frequently extends to chemically related compounds. Local anesthetic preparations containing a vasoconstrictor also may elicit allergic responses due to the sulfite added as an antioxidant. Sedative & Hypnotics (Anxiolytic & Hypnotic) Introduction: A sedative drug decreases activity, moderates excitement, and calms the recipient. An effective sedative (anxiolytic) agent should reduce anxiety and exert a calming effect A hypnotic drug should produce drowsiness and encourage the onset and maintenance of a state of sleep. Hypnotic effects involve more pronounced depression of the central nervous system than sedation, and this can be achieved with many drugs in this class simply by increasing the dose. Anxiety: Anxiety is a physiological & psychological state characterized by somatic, emotional, cognitive & behavioral commutative functions. Symptoms: Anxiety can create, Worry Fear Dread (Extreme fear) Uneasiness Clinical conditions: Clinical condition related to anxiety include, Phobic anxiety & Panic anxiety. Phobic anxiety → Triggered by social interactions e.g. by looking to something like spider, cockroach, looking in open space, travelling in train etc. While in panic disorder attacks of over-welling fear occur in association with somatic symptoms such as sweating, tachycardia, fear, tremors, etc → all these b/c of hormonal release. Sedation refers to decreased responsiveness to any level of stimulation; is associated with some decrease in motor activity and ideation. 43 Tahir Muhammad Graded dose-dependent depression of central nervous system function is a characteristic of most sedative-hypnotics. Dose-response curves for two hypothetical sedative-hypnotics. The linear slope for drug A is typical of many of the older sedative-hypnotics, including the barbiturates and alcohols. With such drugs, an increase in dose higher than that needed for hypnosis may lead to a state of general anesthesia. At still higher doses, these sedative-hypnotics may depress respiratory and vasomotor centers in the medulla, leading to coma and death. Deviations from a linear dose-response relationship, as shown for drug B, require proportionately greater dosage increments to achieve central nervous system depression more profound than hypnosis. This appears to be the case for benzodiazepines and for certain newer hypnotics that have a similar mechanism of action. Sedative-hypnotic drugs depress the CNS in a dose-dependent fashion, Producing calming or drowsiness (sedation) → sleep (pharmacological hypnosis) → unconsciousness → coma → surgical anesthesia →and fatal depression of respiration and cardiovascular regulation Hypnotic drug → at ↓ doses → produce sedation. Sedative Drug → at ↑ doses → produce hypnosis. There is no sharp difference b/w sedatives & hypnotics. → Sedative produce calmness in anxious & restless person along with sleep (no normal sleep) with no effect on the normal person. → Hypnotics can easily produce sleep which resembling the normal sleep. Classification: I. Barbiturates: Further divided on duration basis A) Long acting: Barbitone 44 Tahir Muhammad Phenobarbitone D-O-A → ↑ than 6 hours B) Medium acting: Amilobarbitone Butobarbitone Cyclobarbitone Secobarbitone Pentobarbitone D-O-A → ↑ than 3 & ↓ than 6 hours C) Short acting: Quinolbarbitone Aprobarbitone D-O-A → ↓ than 3 hours D) Ultra-short acting: Thiopental Methohexital Thiomylol D-O-A → 20-30 minutes Used as anesthetics (for induction) II. Benzodiazepines: A) Ultra short acting: Triazolam → as hypnotic Midazolam → as pre-anesthetic D-O-A → less than 6 hours B) Short acting: Lorazepam (Ativan®) Oxazepam Temazepam Lometazepam Bromazepam → commonly used D-O-A → 12-18hours C) Medium acting: Alprazolam Nitrazepam D-O-A → 24 hours Manly used as anxiolytic and hypnotic D) Long acting: Diazepam Chlordiazepoxide → used in alcohol withdrawal also Flurazepam Clonazepam → antidepressant & Anticonvulsant (no active metabolite) D-O-A → 24-48 hours 45 Tahir Muhammad Hypnotic Antianxiety Anticonvulsant Diazepam Diazepam Diazepam Flurazepam Chlordiazepoxide Lorazepam Nitrazepam Oxazepam Clonazepam Alprazolam Lorazepam Temazepam Alprazolam Triazolam III. 5-HT31A receptor agonist: Buspirone Anxiolytic & Non-sedative IV. Β-Blockers: Propranolol V. Miscellaneous: Antihistaminic drug → Diphenhydramine Meprobamate Paraldehyde Chloral hydrate Barbiturates Introduction: These are Barbituric acid derivatives. Today, they have been largely replaced by the benzodiazepines → primarily because barbiturates induce tolerance physical dependence, and are associated with very severe withdrawal symptoms, foremost is their ability to cause coma in toxic doses. Certain barbiturates, such as the very short-acting thiopental, are still used to induce anesthesia. History: In 1903 Barbitone introduced into the market & were used for sleep → sleeping pill. 1912 → phenobarbitone-Na for sleep inducing & Epilepsy. Now upto 2500 different derivatives are developed. Chemistry: Barbituric acid is 2, 4, 6-trioxohexahydropyrimidine. This compound lacks central depressant activity, but the presence of alkyl or aryl groups at position 5 confers sedative-hypnotic and sometimes other activities. P-K: Barbiturates are well absorbed from the GIT. They are widely distributed in the body. The rate of entry into CNS is dependent on lipid solubility. o Highly-lipid soluble thiopentone o Less lipid-soluble ones pentobarbitone 46 Tahir Muhammad o Very less lipid soluble Phenobarbitone Plasma protein binding varies with the compound, e.g. thiopentone 75%, phenobarbitone 20%. Barbiturates cross placenta and are secreted in milk can produce effects on the foetus and suckling infant. significantly excreted unchanged in urine. Sites & Mechanism Of Action: Barbiturates act throughout the CNS & has got no selectivity like BDZ. That’s why it develops anesthetic effects & coma. Non-anesthetic doses preferentially suppress polysynaptic responses. Facilitation is diminished, and inhibition usually is enhanced. Sites: Presynaptic → Higher centers of brain Postsynaptic → in spinal cord The sedative-hypnotic action of the barbiturates is due to their interaction with GABAA receptors, which enhances GABAergic transmission. The binding site is distinct from that of the benzodiazepines. Barbiturates potentiate GABA action on chloride entry into the neuron by prolonging the duration of the chloride cha