Surfaces and Interfacial Chemistry Part 2 PDF
Document Details
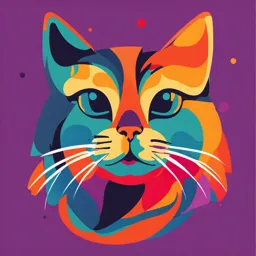
Uploaded by CheaperBlueLaceAgate
The University of Zambia
Tags
Summary
This document is an Advanced Physical Chemistry and Laboratory document from the University of Warwick that covers surfaces and interfacial chemistry. It includes details on topics like crystallisation, dissolution, and in-situ SPM techniques. Note, the document is not a past paper based on the provided text.
Full Transcript
lOMoARcPSD|6077384 Surfaces and interfacial chemistry part 2 Advanced Physical Chemistry and Laboratory (The University of Warwick) Studocu is not sponsored or endorsed by any college or university Downloaded by Anushaya Jeyabalan ([email protected]) lOMoARcPSD|6077384 Pat Notes Scope of interfac...
lOMoARcPSD|6077384 Surfaces and interfacial chemistry part 2 Advanced Physical Chemistry and Laboratory (The University of Warwick) Studocu is not sponsored or endorsed by any college or university Downloaded by Anushaya Jeyabalan ([email protected]) lOMoARcPSD|6077384 Pat Notes Scope of interfaces: Living systems and models Carbon sink and source processes in the environment I.e. carbon cycle Energy and electrocatalysis Probes and feedback: STM - probe : sharp and hard metal wire (Pt/Ir or W) feedback : electron tunnelling between to tip and substrate (both need to be made of conducting materials) AFM - probe : cantilever with a sharp end (Si or S3Ni4 (silicon nitride)) and known spring constant feedback : elastic force (Hook’s law: F=kx) which is measured by displacement of laser on photodiode. Tip or modified tip translated to and from a surface and the deflection monitored (via laser from back of tip). Piezos used for tip movement. Deflection converted to force using Hooke’s Law. Example below of force curves for a general surface. Can also measure colloidal forces and binding force, etc. SICM – probe : hollow glass pipette containing electrolyte and a quasi-reference counter electrode feedback : Ion migration limited by the resistance of the tip end and gap. Topic 1: In-situ SPM techniques Crystallisation and Dissolution Defects on surfaces: Kossel model o Surface defects are vital for crystallisation as they form traps for newly absorbed atoms/molecules o Restructuring of surfaces happens at defects first o Kink, Step and terrace atoms have large eqm concentrations on real surfaces – hard to get perfect surface In Situ EC STM o STM measurements can be made in electrolyte solutions simultaneously with electrochemical measurements/control o Can only be applied to SC or conducting materials to build up a topography image using the current that flows between tip and surface o Control potential of working electrode 1 with respect to reference electrode o Reference – stable, well defined potential – can apply a driving potential, and measure the current flowing. Opposite sign occurs at the other electrode, and there is a flow of electrons Downloaded by Anushaya Jeyabalan ([email protected]) lOMoARcPSD|6077384 o Bipotentiostat - allows to work with 2 electrodes, 2nd working electrode is the tunnelling tip o WE2 is the tunnelling tip – we don’t want an electrical current to flow, we want a tunnelling current. We therefore can’t use a conducting wire into solution otherwise it will act like a large electrode. Hence, we shield with an insulator and leave a small part exposed. o Also need to make sure the potential applied wont cause the tip to do an electrochemical current, and compete with our tunnelling current o The AFM cantilever is immersed in electrolyte solution. And a 3-electrode electrochemical set up will be used to control the potential at the substrate (WE) and measure the WE current, using a bipotentiostat. The potential is applied between the WE and RE and the current is measure between the WE and CE. o Advantages over in situ EC AFM: i) The topographical feedback on AFM is independent of electrochemical current from the substrate. (STM feedback can be convoluted with electrochemical processes at the surface of the substrate and the tip) ii) Study where the electrochemical processes changes the surface with non-conducting material ( e.g. polymerisation and metal passivation with oxide layers) iii) Provides information of other surface properties (adhesion, elasticity, hydrophobicity etc) Video rate imaging o Move tip as quickly as possible (milliseconds) and capture images of dynamic process o Using a scan pattern and in constant height imaging mode o Therefore, atoms will be up close when tip is on the top terrace, but lower terrace sees a larger gap and smaller tunnelling current – so the resolution is lower o Dynamics: Reach equilibrium. One atom mobile o Applications: Cu/Cu+ Cu deposition kinetics – fuzziness around step edges mean that there is a lot of dynamics going on at that site. -movement L-cystine crytsl growth/dissolution Growth and Dissolution Processes – questions to ask yourself: o Mass transport – must be controlled, as we need to know how things are arriving at the surface o Relationship between microscopic surface structure, reactivity, and time dependence o Are the rates at individual crystals the same? – defects/bulk Dissolution o Laser spot on the back of A, tip Figure 1: Growth, opposite arrows for dissolution Downloaded by Anushaya Jeyabalan ([email protected]) lOMoARcPSD|6077384 o Building models for mass transport o Crystal is dissolving, cysteine moves out of solution o Slowest step will limit rate: if this is cysteine moving out then the crystal will be saturated with cysteine and a concentration marked at Csat. The experimental shows that this is not the case and that actually both steps are relevant. – extra detail o General overview: With STM we can look at dynamics of surfaces spirals/screw dislocations AFM Force Curves AFM force curve 1 - Probe-substrate interaction: attraction and adhesion o Approach tip to surface or visa versa in the z axis and monitor the force as we do this, relative to the displacement of the tip. o Plot this onto the graph to the right adhesion force, jump to contact, hookes law, force, distance o Force of attraction begins to pull the tip to the surface, bringing the tip closer and the force gets stronger o ‘Jump to contact’ occurs -C o At D, the force to pull apart is greater than jump to contact and the tip retracts – adhesion force AFM Force curves 2: soft substrate o Some compliance of the surface as it pushes against the tip and will deform the surface, causing a deviation in the curve. – use to analyse how soft the surface is AFM Force curves 2: Replusion o Pushes tip away -no jump to contact Probing double layer with AFM o Attraction vs repulsion forces – negative charge screened, depending on electronic strength and attraction increases as closer together o Colloidal stability? Which force wins o As ionic strength goes up, electrolyte conc goes up, debeye length goes down Example 1: o AFM tip replaced with a glass microsphere o Surface negatively charged o High electrolyte concentration/low ionic strength repulsion felt at bigger distances, charge screened more efficiently o In low electrolyte concentrations, repulsion felt at closer distances Example 2: o Purple membrane o Various approach curves at different concentrations o Increase electrolyte concentrations, repulsions felt at closer distances Downloaded by Anushaya Jeyabalan ([email protected]) lOMoARcPSD|6077384 Topic 2: Chemically and electrochemically sensitive SPM techniques AFM: o A tip is moved towards a surface while detecting the deflection (force), with a laser reflected from the back. Deflection is related to force via Hooke’s Law, for which the spring constant of the tip/cantilever needs to be known. The tip is brought into contact with the surface to a predefined set-point force (deflection) and then retracted Chemical Force Microscopy (CFM) o Chemically functionalised AFM probe – specific properties o Strong affinity of sulphur to gold so an alkanethiols might be a good surface to use with a tip coated in gold (yellow bit in the diagram), as the sulphurs would interact with the gold surface – making gold thiolate and self-assembled monomer around the whole of the AFM tip o Gold is hydrophilic Force curves for CFM: in ethanol o Different functional groups come into contact with each other on nanoscale o Lots of measurements and histograms made on a surface o COOH-COOH has jump to contact, where the horizontal line dips slightly down. We then see a very strong adhesion force, (vertical line coming from the horizontal line) and whereby the diagonal line shows the level of force needed to pull apart the surface and tip o CH3-CH3 has a smaller adhesion force o COOH-CH3 we see a repulsion rather than a jump to contact (the line sort of bends upwards) o We can see how the COOH-COOH interactions are stronger than the C-C interactions Friction force/ lateral force o Apply a particular load force and then monitor the torsional force as the tip moves across the surface o Obtain a friction force – higher for stronger interactions Phase shift in tapping mode o Tip into contact with a surface, if surface holds onto the tip, there will be a lag before the tip can come off the surface, and hence a longer phase shift/out of phase with the driving oscillation. o Like groups hang on to each other and unlike groups slide over each other Past question: Made up of CH3 side groups some jump to contact seen Reduced attraction force Scanning Electrochemical probe microscopes (SEPMs) Downloaded by Anushaya Jeyabalan ([email protected]) lOMoARcPSD|6077384 Scanning Ion Conductance Microscopy (SICM) o Classic conductivity cell to measure i.e., mobility of ions o 2 Electrode set up o Measure conductivity via resistivity and cell constant o K (conductivity) = (1/Rsol)/(I/A) where A= cell constant which requires calibration to determine value and is variable o Total resistance = Rp + RAc Principles of SICM o Well defined potential and inject current o Current restricted by taper – very fine, usually glass or quartz on the scale of 10>nm o Bias between 2 electrodes and then measure the current that forms - same way as STM does o How will the probe position effect current? As probe gets closer to surface, current will go down as the flow of ions is restricted as resistance goes up o RAC is the thing that moves, and is proportional to 1/d o Total resistance = RP + RAC o No physical contact between probe and surface – particularly favourable for biological cells. This is a main fact of this as all modes will prevent the tip touching the surface in some way. The system will settle where distance is stable Approach curves for different modes o DC mode Electrodes need stable potentials Apply a bias Measure the current and scan electrode at a certain distance Not very successful as the intrinsic electric potential charges changes as composition changes and therefore the applied potential drifts Flow of ions restricted close to the surface, ion current goes up past a set point and the pipette will raise/ or visa versa o AC mode More robust Oscillate probe position between 2 values relative to the surface at a certain frequency Far from the surface, ion current is steady like in DC Current changes massively over a short range of distance/ basically at the surface and goes up Raise or lower pipette/tip to adjust Ac amplitude Tip follows contours at a constant mean distance o Hopping mode Downloaded by Anushaya Jeyabalan ([email protected]) lOMoARcPSD|6077384 Measure DC current between surface and bulk, so any drift in bulk current is tracked at every signal Vertical approach until ion current changes, which then pipette pulls away. Move sideway only after tip is clear and the target is therefore never disturbed Can do this now very quickly, at a high frequency High speed SICM for topography imaging o Dynamics of an image can be analysed to find the motion of the surface o Used in thrombin-induced cytoskeleton dynamics in human platelets o Detection of single ion channels Track topography like normal and then you can see ion channels too Only the SICM pipette contains K+ o Functional localisalisation of KATP Channels Difference between SICM and AFM: Both techniques use a piezo-positioning system to move a probe near a surface to gather images and other information on substrate topography and other properties. The techniques differ in that SICM uses a nanopipette filled with electrolyte and a QRCE as a probe and senses the surface via an ion current flow, whereas AFM uses a tip on a cantilever to sense the surface via a force. Both techniques allow imaging under liquid which is critical for biological applications, eg of living cells. A key advantage of SICM over AFM is that it is non-contact and topographical images obtainable of living cells are better resolved than from tapping mode AFM. Additionally, SICM opens up other possibilities for functional imaging. Notably, individual ion channels can be detected and analysed (diagram from lectures and explanation useful here). Further, one can visualise cell topography dynamics, such as thrombin-induced cytoskeleton dynamics in human platelets Scanning Electrochemical Microscopy (SECM) Current dependent on: o Electron transfer kinetics at the electrode o Ion migration in solution – SICM o Diffusion of reactant to/from electrode – SECM SECM 2 electrode system to the right o Apply potential between probe and reference electrode Measure the current o Sample = insulator/metal (purple) not connected to circuit, as otherwise you would need a 4-electrode system rather than 1 o 4 electrode system: Bipotentiostat, 4 electrodes: ref elec, counter elec working elec (sample), tip = 2nd working elec. == STM system o Spatial resolution governed by size of the electrode not a nanoscale probe Downloaded by Anushaya Jeyabalan ([email protected]) lOMoARcPSD|6077384 o Nanoscale probes No positional feedback could we combine one with something that does have positional feedback? Constant height mode: o How do we separate topography and electrochemical activity? o Positive feedback the circular thing underneath the active site o Is it topography or active site? o Small tips need to be close to the surface But we don’t want ‘tip crash’ topography o Position of nanoelectrodes? o Some restricted movement (flat line) o No feedback? What interfacial properties could we measure? o Use an electrode to detect interesting phenomena i.e. permeability o AFM to identify topography Combine SECM and AFM to do function as an AFM tip and also detect various surface properties Scanning electrochemical cell microscopy (SECCM) imaging modes o Dual barrel probe: Ion conductance microscopy Ion migration dependent on the geometry of the conductivity cell droplet falls measure the change detect by the current flowing between 2 electrodes Surface that is both an insulator and conductor Dynamic electrochemical measurements too o Single barrel probe Probe into meniscus contact with surface droplet onto surface, form a local electrochemical cell o Constant distance scanning regime (AC mode) o Hopping scanning regime (DC/AC mode) Scan through a range of potentials make local electrochemical measurements creating a current/voltage profile o SECCM Examples Nano-probe for electrochemistry + other forms of microscopy i..e TEM to measure properties of the surface + correlation with other analysis Correlative electrochemical multi-microscopy Combination of electrochemical microscopy with various complementary techniques o Electrochemical movie Determine key active sites engineer surfaces with these Reconstruct data: present a series of images as a function of the potential current = activity Kinks can show topography with SEPM Identify where the electrochemistry is taking place bigger the step edges more activity electrocatalyst has Downloaded by Anushaya Jeyabalan ([email protected]) lOMoARcPSD|6077384 Topic 3: Adsorption on solids and chemically functionalised surfaces Adsorption in electrocatalysis Hydrogen oxidation reaction CO2 reduction reaction Types of adsorption Physisorption o Van der waals o -15 – 20 kjmol-1 Chemisorption o Chemical bond between surface and adsorbate Adsorption enthalpy< 0 very exothermic Delta Had = -456kjmol-1 for CO2 on W -703kjmol-1 on Ta Fractional coverage (feta) feta = no.surface sites occupied (Ns) / total no.surface sites (N) For gas phase adsorption = volume of adsorbate adsorbed on surface (V) / volume of adsorbate to complete monolayer coverage (Vm) When feta = 1, then total monolayer coverage Rate of adsorption = dθ/dt Langmuir adsorption isotherm Coverage of surface with adsorbate as a function of the concentration of adsorbate in solution/gas phase Assumptions made: o Adsorption cannot go past the monolayer coverage o All surfaces are equivalent and at most, a site can hold one atom or molecule o Ability of the molecule to adsorb onto the surface is not dependent on occupation of neighbouring sites Adsorption rate = kapN(1-θ) where o ka= adsorption rate constant o p = pressure in gas phase or concentration in solution o N= surface density of adsorption sites (molcm-2) Desorption = kdNθ o kd = desorption rate constant At eqm: dθ/dt = 0 adsorption rate= desorption rate so that kapN(1-θ) = kdNθ Downloaded by Anushaya Jeyabalan ([email protected]) lOMoARcPSD|6077384 Where K = ka/kd so o Kp(1-θ) = 0 o and θ = Kp/(1+Kp) o when Kp1 θ=1 (monolayer coverage limit) Graphical representation of Langmuir isotherm: How do we determine K and Vm? Traditionally, θ was measured as a change in gas volume or concentration i.e. How true are Langmuir assumptions? DeltaHad should be independent of θ if Langmuir assumptions hold true Example 1: Adsorption of CO onto Pd o Large deltaad probably chemisorption o Can see a discontinuity of deltaad and therefore Langmuir doesn’t hold true (at least after 0.5, and therefore not the full range) o Bridging at lower site coverage up to 0.5 most active sites filled/energetic Example 2: Adsorption of N2 on graphite at 77k o Much smaller deltaad most likely to be physisorption o Decrease in enthalpy most active sites filled first o Interaction in first layer (θ up to 1) different to 2nd layer can see a drop off o As we add more N2 (up to 1) enthalpy goes up quadropolar interactions/herringbone N2 sitting differently because it is now layering on top of N2 different interactions condensing N2 down onto the surface Example 3: H2 on polycrystalline Ni o Big decrease in enthalpy after sites filled energetic sites first, leaving behind less favourable sites creating a nickel hydride type surface unfavourable dipole interactions doesn’t follow Langmuir o Dissociative adsorption goes against Langmuir that prefers molecular adsorption Example 4: Self-assembled monolayers (SAM) o Adsorbing species is thiolate o Proton reduced to H2 o Surface bond strength = 200kjmol-1 chemisorption Preparation of SAMS on Au o SAMS are formed spontaneously by exposure of solid substrate to a solution of surface active molecules only requires a beaker SAMS from thiols Exercise 5: o Downloaded by Anushaya Jeyabalan ([email protected]) lOMoARcPSD|6077384 Fractal surfaces Euclidian geometry The further you look into something, the more patterns you find Generated by a positive feedback loop Object operation modified object (positive feedback loop) back to object With ruler length r1, object length = 2r1 Ruler r2, object length = 8r2 or 8r1/3 Fractal dimension = N(R) (no.rulers) = Cr-D D= fractal dimension (unknown), C=constant, r= length of ruler For r1, what is D? o N(r1) = Cr1-D = 2 o N(r2) = Cr2-D = C(r1/3)-D = 8 o ln2 = lnC – Dlnr1 o ln8 = lnC – Dlnr1/3 o (2) – (1) = ln8 – ln2 = -Dlnr1 -Dlnr1/3 o ln8/2 = D (-lnr1 – lnr1/3) = -ln(r1 x 3/ r1) o So that ln4 = Dln3 D= 1.26 Measured length = N(r) x r = Cr-D x r = Cr(1-D) So for a perfect line, D=1 and so length = C, independent of ruler size For a non-perfect line D>1 and so that the measured length goes up as the ruler size goes down Can also be applied to a plane surface surface roughened by the successive application of a formula to create a fractal or self similar surface: o N(sigma) = C sigma-D/2 o where N=no. objects with area sigma o Sigma = surface area of object/ cross sectional area = area o Surface area = A2g-1 = molg-1 x 6.02x1023mol-1 x A2 Can then plot ln[N(sigma)] = LnC – (d/2) ln(sigma) Examples in real life: Beetle can drink in the desert from having a waxy back, apart from one wax free area which allows for beetle to drink High fractal dimension amplifies the effect of surface properties larges surface area more hydrophobic provide more surface by roughening it Surface tension, paint Topic 5: Liquid surfaces Surface tension Droplet with spherical shape shape has minimum surface area to volume ratio wants to minimise surface area bulk interactions can be maximised Downloaded by Anushaya Jeyabalan ([email protected]) lOMoARcPSD|6077384 Molecules in a liquid: o Bulk liquid Molecules face equal forces of attraction in all directions o Interface more random forces, net inward pull and contraction at the surface as surface area is to be minimised Comparing the two systems (bulk and interface), we can divide surface energy (J) by area (m2) o Work of forming a surface o dw = γdA γ=surface tension o work = force (N) x distance (m) o So that γ = force (N) / unit length of interface (m-1) Surface tension can be considered in 2 ways: o energy per unit area or force per unit length of boundary Intermolecular forces responsible for surface tension o Van der waals o h-bonding o metal bonding o ionic interactions Interfacial tension is the sum of all contributions = γd (dispersion) + γh (h-bonding) + γm (metallic bonds) + γi (ionic) = γd (always present to some degree) + γsp (specific to system) Exercise 8: order of increasing γ magnitude at room temp: mercury metal bonding (485 mNm-1) water hydrogen bonding (72.8 mNm-1) see later how this is modified with additives n-octanol hydrogen bonding (27.5) n-octane van der waals (21.8) n-hexane van der waals (18.4) Soap bubbles + Young-Laplace equation Soap bubbles Thin film of liquid with gas in it Thin film has lots of layers of mols inner part is like the bulk liquid Shrinking bubble would allow more mols to move into inner part (less surface area) Why does it not shrink to reduce surface area? o Bubble surface area = 4πr2 x 2 o Bubble surface area after shrinking = 4π(r-dr)2 x 2 o Energy gain from shrinking the bubble + reducing the surface area is balanced by the rise in pressure that would result, causing the work to be done in compressing air inside Downloaded by Anushaya Jeyabalan ([email protected]) lOMoARcPSD|6077384 Work done due to rise in pressure= (pin - pout) x A x dr = force x distance for a stable bubble, forces balance so that dw = γdA So: 8r2 - 8(r-dr)2 = (pin - pout) x 4r2 dr o 16r dr = (pin - pout) x 4r2 dr ignore any dr2 values o change in p = 4/r For a liquid drop or a cavity where there is only one surface o Change in p = 2/r young-laplace equation Electrochemical bubble formation: producing gaseous products sticks to the electrodes and then impairs process Current voltage curves for H+ + e- ½ H2 Want to make reduction more negative to drive reaction Exponential dependence on current initially increases Current abruptly decreases Hydrogen forms at electrode and diffuses away Hydrogen soluble in solution concentration at surface hits a max eventually become insoluble as reaction goes forward bubble forms blocks protons to come onto electrode Bubble nucleated, fed by hydrogen that has been converted to proton and diffuses away into bulk of solution Did reaction with various pressures of hydrogen: nA Were then able to analyse the experiment with the following equations -------------------------------- Can be described in terms of the proton come in producing hydrogen escaping from the surface is what we measure KH – Henry’s Law constant (ideal dilute solution) a – electrode radius (disc) RNB – radius of nanobubble F – Faraday’s constant n – no. of electrons in process G(θ,d) – geometric factor o Can then predict the local hydrogen concentration o Limiting current dependent on the hydrogen escaping o Henrys law describes conc. in aqueous solution as a function of hydrogen in the gas phase Surfactants and surface pressure Negatively surface adsorbed: for ions, concentration is lower at the interface than in the bulk To be at the interface, ion loses part of the stabilising hydration shell unfavourable Downloaded by Anushaya Jeyabalan ([email protected]) lOMoARcPSD|6077384 In contrast, solute-solvent interactions< solvent-solvent interactions, then the solute migrates to the surface, lowering surface tension positively surface adsorbed lower pull seen commonly in alcohols Can see as we increase carbon chain greater effect on surface tension realised at lower concentrations strong H-bonding in water causes the surface tension Surfactants Substances that lower surface tension – surfactants Strongly adsorb at air-water interface with polar and non-polar parts 4 types: o Anionic - e.g. CH3 (CH2 )16 COO-Na+ [sodium stearate] o Cationic -e.g. CH3 (CH2 )15N(CH3 )3 +Br- [hexadecyltrimethylammonium bromide] o Non-ionic – e.g. CH3 (CH2 )m (OCH2CH2 )n OH [polyethylene oxides] o Ampolytic (zwitterionic) – e.g. C12H 25N+ (CH3 )2CH2COO- [dodecylbetaine] Surfactants: surface tension and micelle formation Surfactants have to be added in small amounts Critical micelle concentration (CMC) = Discontinuity = surfactants begin to build up micellar structures in solution and compact monolayers at the interface Below the CMC, surfactants live as monomers Density profiles For positively adsorbed surfaces: action at water/air interface: At low concentrations, strongly adsorbing solutes or surfactants solute is very low in the solution pacts into interface and displaces water This is the opposite to negatively adsorbed surfaces If π = expanding pressure exerted by the adsorbed surfactant then: o π = γ0 – γ o where y0 = surface tension of pure solvent and y = surface tension of solvent/solute system I.E. in alveoli o y = 25mNm o after expiration == 0 o Dynamic changes in surface tension control expansion/contraction rates o Surface tension goes up as you breath in and then there is a limit reached Gibbs adsorption isotherm dγ/dcs = -RTΓS / cs dγ= -Γdμs Jm-2 = molm-2 x jmol-1 Downloaded by Anushaya Jeyabalan ([email protected]) lOMoARcPSD|6077384 If Γ is +ve then dγ goes down as dus goes up μs = μ0 + RT lnas for dilute solutions, activity coeffienct = 1 so a=c so that o dμs = RT dlncs o (Recall (dc/c) = ln c; so dc/c = dlnc) o d = -RTsdcs /cs o d/dcs = -RTs /cs Topic 6: Monolayer and Bilayer Films Langmuir film balance change in F = 2 ΔY (tp +wp) recall π = γ0 – γ Hence Δπ = -Δγ = -[ΔF / 2 (tp+wp )] = -ΔF/2wp , if wp >> tp F = ρpglp wp tp + 2γ(tp + wp )(cosθ) – ρlgtlwlhl Gravity Capillary force Buoyancy p =material, g=gravity, l=length, w=width, t=thickness, y=aurface tension l= liquid and p=plate (subscript) Amphiphile dissolved in volatile solvent i.e. petroleum ether added dropwise to the water surface wait few minutes for solvent to evaporate and the amphiplile to spread out across the surface film forms compressed by moving barrier Measure surface pressure exerted by film on mica film Plot surface pressure vs area to obtain isotherms shape of curve tells us intermolecular interactions in the monolayer For stearic acid Abrupt change in pressure seen Characterising monolayers and bilayers: fluorescence E=hv = hc/wavelength Longer rxy in excited state Stokes shift Stoke shift emission generally occurs at longer wavelengths than absorption due to internal conversion Consequence low numbers of fluorescent photons can be detected against high background of excited photons Dark areas high phospholid density (LC = liquid compressed) low fluorescence Light areas low phospholid density (LE = Liquid expanded) high fluorescence Fluorescence recovery after photobleaching (FRAP) Downloaded by Anushaya Jeyabalan ([email protected]) lOMoARcPSD|6077384 Want to measure the surface diffusion coefficient and mobile fraction Measure movement of fluorescently labelled mols in a defined area Some labelled mols bleached with laser monitor recovery of fluorescence by imaging with lower light intensity Non-invasive Green fluorescent protein (GFP) – tagged proteins/lipids often used Want to create a diffusion gradient SICM VS AFM Both use piezo-positioning to move a probe near to a surface to gather images and other info on substrate topography and other properties. SICM uses a nanopippete filled with electrolyte and QRCE as a probe and senses the surface via an ion current flow whereas AFM uses a tip on a cantilever on the surface 15% 3 points in the answer Downloaded by Anushaya Jeyabalan ([email protected])