Spectroscopy PDF
Document Details
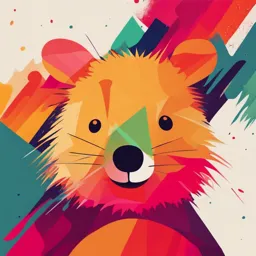
Uploaded by RespectableRegionalism
Dr. Fadzlie Wong Faizal Wong
Tags
Related
- Fluorescence Spectroscopy PDF
- Lecture 1 - BMS2043 - Analytical and Clinical Biochemistry Week 1 PDF
- Lecture 4 -BMS2043 - Spectroscopy final PDF
- BCMB 214 Principles of Biochemical Techniques PDF
- Fluorescence Spectroscopy - BIOC3570 Notes PDF
- Practical Part of Spectral Analysis and Applied Spectroscopy (CP508) 2024-2025 PDF
Summary
This document presents an overview of spectroscopy, covering various techniques like UV-Vis, atomic, NMR, and IR spectroscopy. It explains the basic principles behind these methods and their applications. The document also contains example questions at the end.
Full Transcript
Spectroscopy Dr. Fadzlie Wong Faizal Wong Department of Bioprocess Technology Faculty of Biotechnology & Biomolecular Sciences Content Introduction Atomic spectroscopy UV-Vis spectroscopy Colorimetry Fluorimetry Nuclear...
Spectroscopy Dr. Fadzlie Wong Faizal Wong Department of Bioprocess Technology Faculty of Biotechnology & Biomolecular Sciences Content Introduction Atomic spectroscopy UV-Vis spectroscopy Colorimetry Fluorimetry Nuclear Magnetic Resonance (NMR) Mass spectrometry (MS) Infrared spectroscopy (IR) Introduction - Spectroscopy ➔ study of the interaction between matter and electromagnetic radiation (a form of energy that propagates as both electrical and magnetic waves traveling in packets of energy called photons) ➔ method of analysis used in experimental biochemistry, natural product chemistry and for feed and food analyses - Spectroscopy basis: 1. Interaction between electromagnetic radiation and atoms or chromophoric groups in the molecules. 2. Involves the measurement of the absorption and emission of radiation by atoms and molecules. - Interpretation of the absorption and emission of radiation can gain information about the structure or concentration of the species interacting with the radiation. - Radiation has characteristics that are consistent with both an electromagnetic wave-form and a beam of particles (photons). - Radiation induces transitions between discrete energy levels in an atom or molecule, if the wavelength () of the radiation is related to the energy difference between 2 energy levels, E E = E2 - E1 (Fig.5.1) = hv1 - hv2 = h (c/1) − h (c/2) = quantum of energy, J/mol Where: h = Planck constant c = velocity of light in a vacuum v = frequency (Hz) = wavelength Figure 5.1 Principles of absorption spectroscopy, illustrating quantified energy levels; E = E2 - E1 = hv = hc/ for excitations between electronic, vibrational, and rotational levels; h = Planck constant, v = frequency, = wavelength, and c = velocity of light (electromagnetic radiation, which for light in a vacuum is 2.9979 x 1010 cms-1 ~ 300,000 kms-1) Insert figure 5.1 - Molecules have discrete nuclear spin, electron spin, rotational, vibrational and electronic energy levels. - Radiation will be absorbed and cause transitions between appropriate energy levels depending on its frequency. - Excitation is a fast process, occurring in about 10-15 s, and the excited state corresponds to unstable conditions with lifetimes of about 10-9 to 10-7 s, which are utilised in relaxation methods. Figure 5.2 The electromagnetic spectrum of continuous radiation, spectral characteristics, and associated spectroscopy - Spectroscopic methods based on determination of energy required for excitation ➔ absorption spectroscopy. - Measure the energy released on the return to the ground state ➔ emission spectroscopy. - Nuclear magnetic resonance (NMR), IR and UV- Vis spectroscopy are the major procedures for studying molecular adsorption of radiation in the appropriate regions of electromagnetic spectrum. - Fluorescence and phosphorescence involves studies of the emission of radiation after initial absorption of radiation (Fig 5.1). - Atomic absorption and atomic emission spectroscopy ➔ to study electronic transitions in atoms. Figure 5.1 Principles of absorption spectroscopy, illustrating quantified energy levels; E = E2 - E1 = hv = hc/ for excitations between electronic, vibrational, and rotational levels; h = Planck constant, v = frequency, = wavelength, and c = velocity of light (electromagnetic radiation, which for light in a vacuum is 2.9979 x 1010 cms-1 ~ 300000 kms-1) Insert figure 5.1 Atomic spectroscopy - Collectively known as flame spectroscopy, based on the ability of flames to produce free atoms and other atomic species from inorganic analytes in solution. - Analysis basis ➔ placing atoms in a flame or a heat generated by the electrothermal energy - Absorption of light corresponding to specific wavelengths determined by the type of atom being analysed. - Allow both identification of the atoms and their quantification, as the energy absorbed is proportional to the number of atoms present in the optical path. - Two techniques :- (a) emission flame spectroscopy (b) atomic absorption spectroscopy - Both techniques measure the absorption of a beam of monochromatic light by atoms in the flame (Fig. 5.3) and different lamps are used for different element (Table 5.1). - The first stage ➔ nebulization * Analyte solution is drawn into the burner via a narrow capillary as a result of the high-velocity gas stream across the tip of the capillary. * The droplet-size distribution of the aerosol, which is important for the subsequent states, is affected by the physical properties of the solution, e.g. viscosity, density and surface tension, flow rates of the nebulizer gas and sample solution. * The aerosol will be evaporated to solid particle before vaporisation to gaseous molecules. * Gaseous molecules will then be atomised and excited. Insert Figure 11.2 UV-Vis Spectroscopy a) Instruments - Various types of instruments are available. - Example ➔ * single – beam * double – beam * multibeam - Double beam UV-spectrophotometers contain a beam splitting arrangement, which enables a simultaneous comparison of the absorption by both the sample and reference cuvettes (Fig. 5.4) - The data are commonly recorded on a paper chart, shown on a display unit and/or transferred to a computer for additional data treatment. - Relatively simple single-beam spectrophotometer is cheaper and of more limited performance. b) Solvents and cuvettes - UV-Vis spectroscopy is performed with the analytes in solution, which may be limited by the availability of suitable solvents. - The organic solvents required to dissolve various low molecular weight biomolecules, in solution most often have simple structures, and they only tend to absorb light toward the short wavelength end of the UV spectrum. - This type of absorption for the solvent is often said to have a cut-off value, which is the wavelength below which the solvent absorption is greater than 0.3 in a cuvette with a 1 cm path- length (Table 5.2) - The material used for the cuvettes must be of special optical transparent material. - Plastic cuvettes are acceptable in the visible spectrum, where high precision is not required, otherwise they should be made of glass, and for the UV spectrum, quartz cuvettes are required. - Typical cuvettes for routine work are 4 cm in height with a 1 cm x 1 cm base area (1 cm path- length), but various types of special cuvettes with other path lengths are also available. c) Absorbance and transmittance - Quantitative analysis in photometry (science of the measurement of light) absorption spectroscopy is based on the Beer-Lambert law, which is a combination of: i. Lambert’s law ➔ relates the radiation absorbed to the cell pathlength (diameter of cuvette). ii. Beer’s law ➔ relates the radiation absorbed to the solute concentration. - Lambert’s law states that the change in radiation intensity, dIx, as it passes through a thin section of a transparent medium is proportional to the incident radiation intensity, Ix and the distance traversed by the beam dx (Fig. 5.3). - dIx = kIxdx (Eq 1) k = constant of proportionality - Beer’s law states that each molecule of solute absorbs the same fraction of radiation incident upon it, regardless of concentration, in a non- absorbing medium. - k is proportional to the number of molecules, or the molar concentration of solute, C. k = aC (Eq 2) a = constant of proportionality - Eq. 1 and 2 can be combined into the Beer- Lambert Eq. ➔ -d Ix = a C Ix dx (Eq 3) - Can be integrated between x = 0 and x = l, l = cell pathlength (Fig. 5.3). * When x = 0, Ix = Io = intensity of the incident radiation. * When x = l, Ix = I = intensity of transmitted radiation at the required wavelength. I I − dIx / Ix = a C dx I0 0 ln I0/I = aCl I0/I = exp (-a C l) (Eq 4) - The left-hand side of Eq. 4 = fraction of radiation transmitted by the solution. - The absorbance of a sample is defined as ➔ A = log10 Io/I. (Eq 5) - For a uniform absorbing medium, the proportion of the radiation passing through the cuvette is called the transmittance, T, where ➔ T = I (Eq 6) Io - The percentage light transmittance , T % ➔ T % = I/ Io x 100 = 100T log10 T % = log10 100 + log10 T = 2 - A (Eq 7) - A graph of T (Io/I) plotted against the [ ] (C) in the cuvette will give a hyperbolic curve. - the extent of radiation absorption is more commonly treated as absorbance (A) or extinction (E), defined by Bouguer-Lambert-Beer’s law ➔ * A = E = log 1/T = log (Io/I) = C l (Eq 8) - is a constant and known as absorption coefficient, usually is written as ➔ c () l to specify the concentration unit, light path and wavelength - For example, for concentration unit = M, l = 1 cm and = 430 nm , 11cm M (430 nm) - When C is in mol/L (M), l is in cm and logarithms to the base 10 are used, the is known as the molar absorption coefficient or molar extinction coefficient or the molar absorbancy index (M-1 cm-1). Question 1 ➔ An aqueous solution of iridine-5'-triphosphate at a concentration of 57.8 mg/L (Mr = 586), gave an absorbance of 1.014 with a light path of 1 cm. Calculate the molar absorption coefficient. What would be the absorbance of a 10 M solution and what would be its percentage of light transmittance? Solution 1➔ A = log 1/T = log (Io/I) = Cl C = 57.8 x 10-3 / 586 = 9.86 x 10-5 M = 1.014/ (9.86 x 10-5 M x 1 cm) = 1.028 x 104 M-1 cm-1 - For a 10 M solution ➔ A = 1.028 x 104 M-1 cm-1 x 10-6 M x 1 cm = _________ - The percentage light transmittance T % ➔ log10 T % = 2 - A = ______ T% = ______% - For compounds with unknown the concentration (g/100 ml) can be used, and corresponding extinction of a 1% (w/v) solution in a cuvette with a light path of 1 cm will then give the reference value. - The is known as the specific absorption coefficient ➔ 1cm 1% () - The specific absorption coefficient can be v/v concentration unit. - 1% E 1cm ➔ often quoted for proteins. - This will then give the relation ➔ A = E = E 1% C' l (Eq 9) 1cm C’ = concentration in g/100 ml l = 1 cm C’ = E = g (Eq 10) E 1% 100 ml 1cm OR C = E (10) = g or mg (Eq11) E 1% L ml 1 cm 1% - The size of E 1cm is determined from experiments where A is measured at different C’, followed by plot of A against C’. d) Determination of protein [ ] by UV spectroscopy - Protein absorbs light in the UV region at 200-220 nm and at 270-290nm (Fig. 5.6 and Table 5.3). - The absorption in the first region is a function of all protein amino acids, especially the peptide groups and the aromatic side chain. - The absorption at 270-290 nm is mainly determined by the proteins content of Tyr and Trp ( 280nm 1400 M-1 cm-1 and 5600 M-1 cm-1, respectively). - The other aromatic amino acid, Phe, has max at 257.6 nm (257.6nm 195 M-1 cm-1), only gives a limited contribution to the protein absorbance at 280 nm. Colorimetry - Based on utilisation of the visible area of the electromagnetic spectrum (Box 5.1). - Important for experimental biochemistry both in qualitative and quantitative methods of analysis. - The colour seen in the visible area of the spectrum is complementary to the vectorial sum of the absorbing peaks in the visible area (Box 5.1). - Yellow samples e.g. carotenes have the absorbing peaks in the blue region, the blue-violet-red anthocyanins have absorbing peaks in the green- yellow area and the haemoproteins absorb in the blue-green area. - Detection of many biomolecules with only weak chromophore groups (Table 5.4) often requires derivatization of functional groups with chromophore reagents, which results in colours. - Such less specific staining can be utilized in qualitative detections, spot test or in quantitative methods of analyses based on Eq. 8 as for direct UV spectroscopy. - Table 5.6 shows a list of derivatization or staining procedures. Fluorimetry - Fluorescence (Fig. 5.1) occurs when chromophore groups lose only part of their excitation energy by transfer to an intermediate vibrational or rotational energy level. - The final transfer to the ground state will released less energy than required for excitation ➔ resulting in a higher wavelength for the fluorescence spectrum compared with the absorption spectrum. - Fluorescence spectroscopy is thus limited to molecules with the fluoresce property. - Compounds that do not fluoresce can be labelled with a fluorescent molecular group before analysis/detection (Fig.5.16). - Fluorescamine ➔ amine groups that used for detection of proteins after electrophoresis and in amine and amino acid analysis. - Ethidium bromide ➔ sensitive detection of nucleic acids after electrophoretic separation. Insert figure 5.16 - In protein, Tyr and Trp are native major fluorescent groups. - The fluorescence spectrum for Tyr has its maximum around 300 nm which is about 20 nm higher than the corresponding absorption spectrum (Fig. 5.9). - NADH and NADPH are fluorescent compound ➔ allow many redox-enzymes that utilised these coenzymes to be analysed by spectrofluorimetry. - Flavoproteins also give fluorescence ➔ can be measured at 525 nm after excitation at 345 nm. - Compared with UV-Vis spectrophotometers, the main difference in a fluorimeter is the need for two monochromators, one for excitation and one for emission. Nuclear Magnetic Resonance (NMR) - NMR spectrometry is a special form of absorption spectrometry in which nuclei of certain isotopes in a magnetic field absorb electromagnetic radiation in the radio frequency region (Fig. 5.2). - NMR spectrum ➔ a plot of the frequencies of the absorption peaks versus peak intensities. - Minute magnet property of nuclei is an essential characteristic for NMR spectroscopy studies, e.g.1H, 3H, 13C, 15N and 31P (1H and 13C are widely used species). - Analysis basis ➔ * When a sample is placed in a strong external magnetic field, the different orientations of the nuclear spin will have different energies. * The energy difference between two energy stages corresponds to microwave radiation, which will sufficient to flip the nuclei from one energy state to another. - NMR spectroscopy is a very powerful technique for structural identification of biomolecules, e.g ➔ (Fig. 5.18 and Fig. 5.19) (a) one-dimensional 13C NMR ➔ determine the chemical structure. (b) 1H NMR ➔ provide additional information on fine structures, including configuration and conformations. Mass spectrometry (MS) - Mass spectroscopy (MS) is not a spectroscopic ➔ as it does not involve electromagnetic radiation and quantum principles. - MS depends upon thermodynamic stability of the compounds or ions produced in the commonly used electron-impact (EI) mode, where molecules in the vapour phase are bombarded with a high- energy electron beam. - + Ve ions produced are separated in a magnetic field on the basis of their mass-to-charge (m/z) ratio and the mass spectrum recorded is presented as computer-plotted bar graph of abundance(vertical peak intensity) versus m/z (Fig 5.20). - Recently, MS (MALDI-TOF) has been employed to study protein mass➔ new technique. - MALDI-TOF ➔ Matrix – Assisted – Laser - Desorption of Ions, electrically accelerated into a Time Of Flight mass analyser. Infrared spectroscopy (IR) - IR spectroscopy covers the region of the electromagnetic spectrum between the red end of the visible range and the microwave region. - IR radiation causes transitions between vibrational energy levels and also between rotational energy levels. - The frequency of absorption due to transitions between vibrational energy levels allows the identification of functional groups within the molecule. - When IR radiation impinges on the molecule, energy is absorbed and the amplitude of the appropriate vibration is increased. - When the molecules reverts from the excited state to the original ground state, the absorbed energy is released as heat. - In order for the radiation to be absorbed, the vibration must involve a change in the dipole moment of the molecule. - The frequencies associated with chemical groupings ➔ characteristic group frequencies (Fig. 8.2). - The IR spectra of biological molecules are very complex, e.g the spectrum of a. a. Met. (Fig. 8.3) ➔ many IR absorption bands cannot be interpreted with confidence. - A spectrum in the mid-IR region generally allows the identification of some of the functional groups present in a molecule, e.g alkene, amino, aromatic, carbonyl, carboxy and hydroxy groups (Fig. 8.4).