AB Power Engineering Review Materials PDF
Document Details
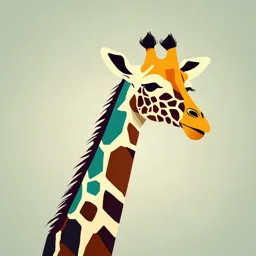
Uploaded by CaptivatingRational1067
Bulacan Agricultural State College
null
null
Tags
Summary
This document provides a review of internal combustion engines, discussing various types, classification, and operational characteristics. It covers key components, processes, and performance metrics like horsepower.
Full Transcript
REVIEW MATERIALS AB Power Engineering Internal combustion engines operate with heat generated inside, while external combustion engines rely on external heat sources. a. External combustion engines such as steam trains and boat...
REVIEW MATERIALS AB Power Engineering Internal combustion engines operate with heat generated inside, while external combustion engines rely on external heat sources. a. External combustion engines such as steam trains and boats, utilize external heat sources like coal to generate power. They have lower thermal efficiency compared to their internal counterparts. b. Internal combustion engines use fuels like gasoline and diesel, resulting in higher temperatures and pressures compared to external combustion engines. This highlights the efficiency of internal combustion engines. The classification of internal combustion engines can be based on fuel ignition methods, including spark ignition and compression ignition. This classification aids in understanding their operational mechanics. Diesel engines have a higher compression ratio of 1:16 compared to gasoline engines' 1:7 or 1:8. This higher compression allows for efficient fuel ignition without a spark. Engines can be classified based on their stroke cycles, such as two-stroke and four-stroke engines. Each type has distinct operational characteristics and power generation methods. Two-stroke engines often produce more smoke due to the burning of oil along with fuel. This pollution has led to restrictions on their manufacture in many regions. Vertical engines are stable but limit fuel usage, while horizontal engines offer flexibility in mounting options. This requires a specific orientation to prevent fuel spillage, impacting their usability in various applications. Unlike electric motors, they cannot be mounted in multiple positions. The arrangement of pistons in engines can be vertical or horizontal, significantly affecting their performance and application. Understanding these arrangements helps in selecting the right engine for specific needs. Different engine types, such as radial and V-type engines, are designed for specific applications, including aviation. This diversity allows for tailored solutions depending on the operational requirements. Understanding the operation of internal combustion engines is essential for recognizing how they function. The process includes various strokes: intake, compression, power, and exhaust, each playing a vital role. The internal combustion engine operates through a series of strokes, where air and fuel mixtures enter and are compressed for ignition. This process is crucial for generating power. The difference between gasoline and diesel engines lies in their ignition methods. Diesel engines utilize injectors instead of spark plugs, leading to different combustion processes. Various systems support engine operation, including the ignition system, fuel system, and starting system. Each system contributes to the engine's efficiency and performance. The relationship between piston displacement and clearance volume is essential for understanding total engine volume and compression ratios. These elements influence the engine's efficiency and power generation. Effective pressure is generated during the power stroke and is crucial for engine performance. This pressure directly affects how well the fuel combusts and powers the engine. Horsepower can be categorized into various types, including indicated power and brake horsepower. Each type measures different aspects of engine performance and efficiency, showing the power available for work. The carburetor is crucial for creating the correct air-fuel mixture, ensuring efficient combustion in the engine. Understanding its mechanisms can enhance engine tuning and performance. Choke operation is vital for cold starts, limiting air entry to enrich the fuel mixture. This adjustment helps engines start more readily in low temperatures. Key components include excess fuel devices and bleeding techniques for fuel systems. Excess fuel devices enhance starting by allowing more fuel to enter the engine, especially in cold conditions. Proper management of air-fuel ratios is crucial for efficient operation. Different chamber designs in diesel engines affect fuel injection and combustion. Understanding direct and indirect types helps in troubleshooting and optimizing engine performance. Bleeding techniques are essential for removing air from the fuel system when running low on fuel. This process prevents engine stalling and ensures smooth operation after refueling. Understanding clearance volume in engines is critical for calculating engine performance. It represents the volume remaining when the piston is at the top of its stroke. The clearance volume is essential for determining the total volume of the cylinder, which includes both the clearance and displacement volumes. This measurement is crucial for engine efficiency. Indicated horsepower is calculated using various parameters, including pressure, stroke length, and the number of cylinders. This is essential for evaluating the engine's power output effectively. Conversion factors play a significant role in calculations, such as converting horsepower to foot-pounds per minute. Understanding these conversions is necessary for accurate engineering assessments. The formula for compression ratio is essential for determining engine efficiency. It is calculated using piston displacement and clearance volume, impacting overall performance. Indicated power is always higher than brake horsepower, which is crucial in engine analysis. This relationship helps identify potential issues in engine performance metrics. Specific fuel consumption can be calculated using fuel consumed and output power. This measurement indicates how efficiently an engine uses fuel relative to its power output. The calculation of power using torque and RPM, emphasizing the importance of understanding and applying the correct formulas for accurate results. It highlights the conversion of measurements, and the nuances involved in calculations. The formula for calculating power is derived from torque and RPM. This relationship is crucial for accurately determining engine performance based on the given measurements. Conversion between different units, such as kilogram meters to horsepower, is necessary to ensure proper understanding of engine specifications. Small differences in decimals can affect the results significantly. Fluid power devices and their functions are essential in mechanical systems. Understanding these devices, like actuators and carburetors, is crucial for efficient engine performance. Actuators are devices that utilize fluid power to create mechanical force and motion, playing a vital role in controlling engine operations. They are essential for various applications in engineering. Atomization is the process of breaking fuel into fine droplets using high-velocity air, crucial for efficient combustion in engines. This process enhances fuel mixing and energy output. Carburetors mix fuel and air in the correct ratio before entering the combustion chamber, significantly affecting engine performance. Proper understanding of carburetors is essential for maintaining engine efficiency. The importance of spark plugs in diesel engines is highlighted, emphasizing that they are not present in such engines. Understanding this can clarify engine mechanics for learners. Piston displacement is explained as the volume displaced by a piston during its movement. This concept is vital for calculating engine performance and efficiency. Engine Terminology Bore - size of the opening of the cylinder almost equal to the the diameter of the piston plus the rings Stroke - distance traveled by a piston from the TDC to the BDC Top Dead Center (TDC) - the uppermost position of the piston during the compression and exhaust stroke Bottom Dead Center (BDC) - the lowest most position of the piston during the intake and power stroke Engine Displacement (PD) - volume displaced by a piston in one stroke Clearance Volume (CV) - volume in the combustion cylinder when the piston is at the TDC position Compression Ratio (CR) - ratio of the total volume to the clearance volume Mean Effective Pressure (MEP) - amount of pressure generated during the power stroke of the engine Revolution - one complete rotation of the crankshaft Cycle - series of event occurring one after the other in a definite order and repeats the event after the last one has occurred Firing Order - The sequence of piston power in multiple cylinders in delivering the power stroke Indicated Horsepower - the power generated at the combustion chamber Brake Horsepower - power available at the drive shaft or the crankshaft of an engine Friction Horsepower - power loss due to friction of piston and other component parts of the engine Rated Horsepower - power as specified by the manufacturers Mechanical Efficiency - ratio of the brake horsepower and the indicated horsepower Thermal Efficiency - ratio of the brake horsepower to the power available at the fuel Firing Order - sequence of delivering of power stroke by the piston in multi-cylinder engine Specific fuel consumption - amount of fuel consumed by brake horsepower of the engine Cycle of Events Intake Stroke - fuel and air or air alone is suck in the combustion chamber as the piston moves in downward direction Compression Stroke - fuel and air or air is compressed by the piston as it moves in upward direction Diesel Engine Starting Aids For cold climate starting of diesel engines methods are used to aid starting various 1. Glow-Plugs These are located in the pre-combustion chambers, one plug per cylinder. These glow- plugs are heated by the tractor batteries and glow continuously while the switch is being operated. A ballast resistor indicator is usually on the dash panel to warn the operator that the plugs are glowing. Immediately the indicator glows the starter should be operated and starting is usually achieved quickly if everything is in good order. 2. Thermo start This is a single heater unit fitted in the inlet manifold to heat the ingoing air. A key type of switch operates the heater element; 15-20 seconds is the usual amount of time needed to glow the element and no indicator is fitted. The glowing elements activate a bi-metal strip which then uncovers an orifice to allow fuel to flow on to the glowing element, this sets fire and can be heard in the inlet manifold. On hearing this noise immediately operate the starter, drawing the flame into the engine, which should give immediately starting. 3. Excess-fuel device On some tractor fuel pumps there is a small button to depress or a small T-bar to turn clockwise which allows extra fuel to spray into the engine to aid starting. As the engine starts the small button flies out automatically to reduce the amount of fuel, but the T-bar type must be released manually, or the engine will run very erratically and rich. 4. Ether start An ether-based spray is available in aerosol cans which aids starting by spraying a very small amount into the inlet manifold of the engine as the starter is turning the engine over. This gives instant starting to virtually any engine, however poor the engine's condition may be. Before using this starting method, a few simple rules must be read on the can or the engine may be permanently damaged, or worse still human injury may occur. Four-Stroke Diesel Engine Combustion Chamber Designs Two main types of diesel engine combustion chamber design are employed on tractor engines: a. The direct type This is where the injector is fitted to spray directly on to the piston crown, which may be shaped to form a combustion chamber. Usually, a multi-hole injector is used which spreads the spray evenly over the chamber to give good fuel air mixing. The type of engine usually starts easily and quickly without any special aids, such as heaters or excess fuel devices. b. The indirect type This is where the injector sprays through a single-hole or double-hole injector at slightly lower pressure into a pre-combustion chamber coupled to the main chamber by a tangential passage. Ignition or burning first occurs in the pre-combustion chamber then passes through into the main chamber. Fuel Injection A fuel feeding method for most diesel and newer models of gasoline engines where high- pressure electric pump mixes precisely measured amount of filtered fuel and air. The mixture of fuel and air is then sprayed into the cylinders by fuel injectors. Piston Displacement 𝜋𝐷 2 𝑃𝐷 = (𝐿𝑛) 4 PD – piston displacement, cm³ D – piston diameter, cm L – length of stroke, cm Compression Ratio 𝑃𝐷 + 𝐶𝑉 𝐶𝑅 = 𝐶𝑉 CR – compression ratio PD – piston displacement, cm³ CV – clearance volume, cm³ Indicated Horsepower 𝑃𝐿𝐴𝑁𝑛 𝐼𝐻𝑃 = 33000 HP - indicated horsepower, hpP - mean effective pressure, psi L - length of stroke, ft. A - area of bore, in² N - crankshaft speed, rpm N - number of cylinders 2 for four-stroke engine and 1 for two-stroke engine Brake Horsepower 𝐵𝐻𝑃 = (𝐼𝐻𝑃)(𝑛𝑚) 𝐵𝐻𝑃 = 𝐼𝐻𝑃 − 𝑃𝐻𝑃 BHP - brake horsepower, hp IHP - indicated horsepower, hp ηm - engine mechanical efficiency, decimal PHP - friction horsepower, hp Example: 1. Suppose a 4-cylinder engine has a bore of 3.5 in. and a stroke of 4.0 in., what is the piston displacement of the engine? Given: No. of cylinders = 4 Piston diameter = 3.5 in. Stroke = 4.0 in. Required: Piston displacement Solution: 𝜋(3.5𝑖𝑛)2 𝑃𝐷 = (4.0)(4) 4 = 153.9 in³ Example: 2. Suppose that an engine has a piston displacement of 38.4 in³. If the clearance volume of the cylinder in 2.5 in³, what is its compression ratio? Given: PD = 38.4 in³ CV = 2.5 in³ Required: Compression ratio Solution: (38.4𝑖𝑛3 ) + (2.5𝑖𝑛3 ) 𝐶𝑅 = 2.5𝑖𝑛3 = 16.4 Soil and Water Conservation Engineering Hydrometeorology refers to the study of the relationship between water and weather, focusing on the processes that govern the distribution and movement of water in the atmosphere. Watershed is a horizontal projection of an area containing a natural waterway bounded by an arbitrarily selected outlet and by ridges, summits and hydraulic boundaries such that precipitation falling onto this area is trapped within and consequently discharged through the outlet. Components of the hydrologic cycle Precipitation – all forms of water derived from atmospheric vapor and deposited back on the earth's surface, e.g., rain, hail, mist and snow. Infiltration – process of downward movement of water through the soil surface. The passage of water into the soil surface. Runoff – the portion of precipitation that makes its ways towards streams, lakes or oceans or groundwater flow. Surface runoff includes water that reaches the stream without first percolating into the soil. However, runoff generally means surface runoff. Streamflow includes both surface and subsurface flows. Evaporation – process by which liquid water changes into gas or water vapor. Transpiration – the process by which water vapor leaves the plant body to the atmosphere Evapotranspiration – also, consumptive use, is the quantity of water transpired by the plant including the water evaporated from the soil and water surfaces. Estimation of Runoff Useful definitions referring to runoff components include: Direct runoff – same as surface runoff Subsurface runoff – same as interflow (Lateral movement of infiltrated water when it encounters impervious layer. Such water usually reappears on the surface of the soil at a lower elevation.) Base flow – same as groundwater runoff Estimates of rate of surface runoff depend on two processes: 1. Estimation of rate of rainfall 2. Estimation of how much rainfall becomes runoff Empirical Methods for Estimating Peak Runoff Rate 1. Rational Method Convert everything to get a m³/s value. 𝑄 = 𝐶𝐼𝐴 Q – runoff rate (cfs or cms) C – dimensionless runoff coefficient I – rainfall intensity for the design - assumes that the frequencies of rainfall and runoff are similar - a great oversimplification of complicated process - sufficiently accurate for design of relatively inexpensive structures where the consequences of failure are limited - limited to watersheds of less than 800 ha. Runoff Coefficient (C) - ratio of the rate of runoff to the rate of rainfall Example: If half of the rainfall is "lost" by infiltration, etc, and the other half appears as runoff, then the coefficient, C, therefore is 0.50. Rational Method Time of Concentration, Tc 𝑇𝑛 = 0.0195 𝐿0.77 𝑆 −0.385 - also called as gathering time - the storm duration which will correspond with the maximum rate of run off - time required for water to flow from the remotest point of the area to the outlet 𝑇𝑐 = time of concentration in minutes L = maximum length of flow in meters S = average stream gradient in meters per meter - the difference in elevation between the outlet and the remotest point divide by the length, L 2. Hydrometeorology - the study of the relationship between water and weather, focusing on the processes that govern the distribution and movement of water in the atmosphere. Design of grassed waterway Grassed waterways are open channels protected with suitable grasses constructed along the slope and act as outlet for terraces and graded bunds. They are also used to safely convey runoff from contour furrows, diversion channels and serve as emergency spillways in farm ponds. The three common types of shapes used for grassed waterways are the trapezoidal, triangular, and parabolic. 3. Watershed Hydrology Importance of Hydrology in Soil and Water Conservation Precipitation – information on precipitation is needed in estimating runoff, planning erosion control measures, planning for irrigation and drainage, and water conservation in low rainfall regions. Runoff – needed in designing structures and channels that will handle natural flows of water. Infiltration, Evaporation and transpiration – are required in planning irrigation and drainage systems, moisture conservation practices, etc. EROSION Soil erosion is the detachment & transport of soil particle from one place to the other (David, 1975). Soil erosion is the removal of soil from the land surface by running water or by wind (Schwab et al., 1955) Effects of Soil Erosion - Loss of soil fertility - Raising the beds of streams and rivers, thus reducing their capacity - Silting of reservoirs, thus reducing their capacity and useful life - Damage to agricultural lands due to soil deposition Types of soil erosion 1. Water erosion Sheet erosion - a thin film of soil layer detached and transported by water flowing on the land surface. Rill erosion - finger-like rills appear on the soil surface. Gully erosion - advanced form of rill that cannot be obliterated by plowing. Rills when neglected develop in size and become gullies. Stream bank erosion - erosion of stream banks by flowing water. Coastal erosion - erosion caused by wave action on the seashore. - caused by high velocity winds moving over barren land surfaces. 2. Wind erosion Slip erosion - Landslides and slips due to saturation of slopes, hills, and cliffs. Factors Affecting Soil Erosion Climatic factors – precipitation, wind, temperature, humidity, and solar radiation Soil physical properties influence the extent to which soil can be dispersed and transported. Soil structure, texture, organic matter content, moisture content, and bulk density. Vegetation acts in the following ways: - Interception of falling rain by the foliage helps in absorbing the energy of raindrops. - Transpiration of moisture thus decreasing soil moisture and increasing storage capacity. - Binding effect of root system helps retard erosion. - Decayed roots: provides cavities and pores which promote infiltration. - Increase surface friction reduces runoff velocity. - Topography - Slope - Slope length - Size and shape of watershed Purposes of Soil and Water Conservation - To control runoff and thus prevent loss of soil-by-soil erosion, to reduce soil compaction. - To maintain or to improve soil fertility. - To conserve or drain water. - To harvest (excess) water. Classification of Erosion Control Methods 1. Agronomic or Biological Method - Utilize the role of vegetation in helping to minimize erosion. - Soil management is concerned with ways of preparing the soil to promote dense vegetation growth and improve its structure so that it is more resistant to erosion. - Usually less expensive and deal directly with reducing raindrop impact, increasing infiltration, reducing runoff volumes, and decreasing water velocities. 2. Mechanical or Engineering Method - Depend upon manipulating the surface topography, for example, by installing terraces to control the flow of water. - Largely ineffective on their own because they cannot prevent detachment of soil particles. - Main role is in supplementing agronomic measures, being used to control the flow of any excess water that arises. - In general, mechanical measures are effective soil conservation technologies as they reduce soil loss. Agronomic or Biological Methods 1. Mulching - Mulch is a layer of dissimilar material placed between the soil surface and the atmosphere. - Reduce the splash effect of the rain, decreasing the velocity of runoff and hence reducing the amount of soil loss. 2. Cover Crops - Cover crops such as the legumes or the grasses are plants that grow rapidly and close. - Their dense canopy prevents raindrops from detaching soil particles and this keeps soil loss to tolerable limits. - Cover crops also positively influence physical soil properties such as the infiltration rate, moisture content, and bulk density, increase the organic matter content, nitrogen (N) levels by the use of N2-fixing legumes. 3. Intercropping - Including different kinds of annual crops planted in alternating rows also reduce soil erosion risk by providing better canopy cover than sole crops. 4. Contouring - Farming practice of plowing across a slope following its elevation contour lines. - Rows formed have the effect of slowing water runoff during rainstorms so that the soil is not washed away and allows the water to percolate into the soil. 5. Strip Cropping - Method of farming used when a slope is too steep or too long, or when other types of farming may not prevent soil erosion. - Strips of row crops and closely growing crops are planted on the contour alternately. - Erosion is largely limited to the row-crop strips and soil removed from these is trapped in the next strip down slope which is generally planted with a leguminous or grass crop. Mechanical or Engineering Methods 1. Contour Bunds - Contour bunds are made of earth or stones or terraces that consist of an excavated channel and a bank or ridge on the downhill side for cultivating crops. - Contour bunds are earth banks, 1.5 to 2 m wide, thrown across the slope to act as a barrier to runoff, to form a water storage area on their upslope side and to break up a slope into segments shorter in length than is required to generate overland flow. 2. Waterways - Permanent structures that aim to collect and guide excess runoff to suitable disposal points. - They are constructed along the slope, often covered with grass to prevent destruction, and primarily installed in areas with high rainfall rates. - The purpose is to convey runoff at non-erosive velocity to a suitable disposal point. 3. Tillage Conventional Tillage - involves inversion of soil, usually with a moldboard or disc plow as the primary tillage operation, followed by a secondary tillage with a disc harrow. Primary tillage objective is weed control through burying. Secondary tillage objectives are to breakdown the aggregates and to prepare a seedbed. Control of diseases and insects by burying crop residues. Conservation Tillage - describes the method of seedbed preparation that includes the presence of residue mulch and an increase in surface roughness as key criteria. Normally refers to a tillage system which does not invert the soil, and which retains crop residues on the surface. Minimum Tillage - describes a practice where soil preparation is reduced to the minimum necessary for crop production and where 15% to 25% of residues remain on the soil surface. Less frequency and intensity of cultivation. No Till or Zero Tillage - characterized by the elimination of all mechanical seed bed preparation wherein the soil surface is covered by crop residue mulch. 4. Terraces - A terrace is a levelled section of a hill cultivated area, designed as a method of soil conservation to slow or prevent the rapid surface runoff of irrigation water. - Often such land is formed into multiple terraces, giving a stepped appearance. Design of Bench Terraces Case 1: when cut is vertical - Where S is the land slope in percent - D/2 is the depth of cut - W is the width of the terrace Case 2: When the batter slope is 1:1 𝐷 2 𝑆 𝐷 𝐷= = 𝑜𝑟 = 𝑊𝑆 𝑊 𝐷 100 (100 − 𝑆) 2 +2 - Length of terrace per hectare can be computed by Lha = 1000W - Volume of earthwork is computed by the cross section of the cut portion multiplied by the length of terrace per hectare. - Percent Width lost due to terracing can be computed by the following formula: Contour Bunding - Consists of building earthen embankments across the slope of the land, following the contour as closely as possible. - A series of such bunds divide the area into strips and act as barriers to the flow of water, thus reducing the amount and velocity of the runoff. Types of Bunds - Contour bunds - Narrow base bunds - Broad base bunds - Graded bunds - Staggered trenches Design Considerations 1. Height of Dam and Freeboard Height of Dam – depends on storage capacity required (check elevation area curve). Freeboard – depends on: a) Wind Setup, wave height and wave run up. Wind Setup – tilting of reservoir water surface due to water movement towards the leeward side caused by wind. Wave Runup – height that a deep-water wave runs up the slope. Wave height for moderate-size reservoir areas can be determined by: 1 ℎ = 0.014𝐷𝑓 2 Where: h= height of wave from trough to crest under maximum wind velocity in m 𝐷𝑓 = fetch or exposure in m 2. Top Width - Top widths of dams vary with height and purpose. - Minimum top width for dams is up to 3.5 m, in height it should be 2.4 m. - Top width for dam exceeding 3.5 m in height may be designed by the empirical formula: 𝑊 = 0.4𝐻 + 1 Where: W = top width in meters H = max height of embankment in meters 3. Compaction and Settlement 𝑉 = 𝑉𝑠 + 𝑉𝑒 Where: V = total in-place volume (L³) 𝑉𝑠 = volume of solid particles (L³) 𝑉𝑒 = volume of voids, either air or water (L³) - The void ratio, e is expressed as: 𝑉𝑒 𝑒= 𝑉𝑠 4. Slope Stability - Side slope of an earth dam or levee is dependent on the height of the structure. - On structures less than 15 m high, side slopes should be no steeper than 3:1 on the upstream face and 2:1 on the downstream face. Classification of Dams A. According to Use 1. Storage Dam 2. Diversion Dam - more of river type irrigation system 3. Detention Dam - for flood control B. According to Hydraulic Design 1. Overflow Dam 2. Non-Overflow Dam C. According to Construction Materials 1. Earthfill Dams 2. Rockfill Dams 3. Concrete Gravity Dams Irrigation and Drainage Engineering Water Potential Water is retained or moved through the soil depending on the dominant component of soil water potential Definition: the amount of work that a unit quantity of water in an equilibrium soil water system is capable of doing when it moves to a pool of water in the reference state at the same temperature. Energy State of Water The total energy state of soil water is defined by its equivalent potential energy, as determined by the various forces acting on the water per unit quantity. - In general, flow rates of water in soils is too small to consider kinetic energy. - Therefore, the energy state of soil water is defined by its equivalent potential energy, that is by virtue of its position in a force field. Forces Acting on Soil Water - Capillary forces - Adsorptive forces (adhesion of water to solid soil surfaces) Capillary and adsorptive forces together result in soil matric potential. - Gravitational forces - Drag or shear forces (at soil surface-water interface) Water in the soil flows from points with high soil-water potential energy to points of lower potential energy. Soil-water Potential Gradient - The driving force for flow is the change in potential energy with distance (soil-water potential gradient). These driving forces determine: - Direction and magnitude of water flow - Plant water extraction rate - Drainage volumes - Upward water movement (or capillary rise) - Soil temperature changes - Solute (contaminant) transport rates Soil water potential (ϕwϕw) is the difference in energy state between soil water and pure water, influencing the movement of water from areas of higher to lower potential energy. Components of Soil Water Potential ϕT=ϕm+ϕs+ϕz+ϕpϕT=ϕm+ϕs+ϕz+ϕp where: Matric Potential (ϕmϕm): Results from capillary and adsorptive forces in the soil matrix. It is a dynamic property, theoretically zero in saturated soil. Solute Potential (ϕsϕs): Arises from soluble materials in the soil solution and is influenced by semi-permeable membranes (e.g., plant root cell walls). It drives water movement through diffusion. Gravitational Potential (ϕzϕz): Determined by the elevation of water relative to a reference point, it plays a role in water infiltration and drainage. Pressure Potential (ϕpϕp): Hydrostatic pressure from unsupported water in the soil. It can be positive (saturated soil) or negative (unsaturated soil) and is measured relative to a specified point. Units of Measurement: Energy per unit mass: N-m/kg, J/kg Energy per unit volume: N/m², Pa Energy per unit weight: cm, mm, in, m, ft (Ig + P + GW) - (ETc + DP + SRO + SDL) = ΔSW Ig = ETc + DP + SRO - P - GW + SDL – ΔSW Ig = gross irrigation required during the period ETc = amount of crop evapotranspiration during the period DP = deep percolation from the crop root zone during the period SRO = surface runoff that leaves the field during the period P = total precipitation during the period GW = ground water contribution to the crop root zone during the period SDL = spray and drift losses from irrigation water in air and evaporation off of plant canopies DSW = change in soil water in the crop root zone during the period Water balance equation (Supply = Demand) I + Rn = ET + P + S + SD ± CWS I = Irrigation supply Rn = Rainfall ET = Evapotranspiration P = Deep percolation S = Net seepage SD = Surface drainage CWS = Change in water status Calculation of Irrigation Water Requirements 1. Determine total time and time interval for calculation of the water balance - Cropping season and interval 2. Estimate ETo - Daily or annual 3. Determine appropriate crop coefficient - Kc = f (Crop Growth) 4. Calculate crop evapotranspiration Evapotranspiration 1. Indirect methods 2. Lysimeters 3. Catchment water balance 4. Empirical equation 5. Eddy covariance technique Potential Evapotranspiration (PET) - introduced in the late 1940s and 50s by Penman - defined as “the water loss which will occur if at no time there is a deficiency of water in the soil for use by vegetation” - by Thornthwaite (1943) - defined as “the amount of water transpired in a given time by a short green crop, completely shading the ground, of uniform height and with adequate water status in the soil profile” Reference Crop ET (Eto) - introduced in 1970s to 80s by engineers and researchers - defined as the rate of ET from a hypothetical reference crop with an assumed crop height of 0.12 m (4.72 in), a fixed surface resistance of 70 sec/m and an albedo of 0.23, closely resembling the ET from an extensive surface of green grass of uniform height, actively growing, well-watered, and completely shading the ground - used to study the evaporative demand of the atmosphere independently of crop type, crop development and management practices - so far, the most theoretically sound equation for estimating reference crop potential evapotranspiration - sometimes referred to as the combination equation because it combines the effect of heat transfer and air mass movement in transporting water molecules from the evaporating or transpiring surfaces into the atmosphere Irrigation efficiency is a critical measure of irrigation performance in terms of the water required to irrigate a field, farm, basin, irrigation district, or an entire watershed. It is the ratio of the average depth of irrigation water beneficially used to the average depth applied, expressed as a percentage. “Irrigation efficiency” - is a basic engineering term used in irrigation science to characterize irrigation performance, evaluate irrigation water use, and to promote better or improved use of water resources, particularly those used in agriculture and turf/landscape management. Irrigation efficiency affects the following: 1. economics of irrigation 2. amount of water needed to irrigate a specific land area 3. spatial uniformity of the crop and its yield 4. amount of water that might percolate beneath the crop root zone 5. amount of water that can return to surface sources for downstream uses or to groundwater aquifers that might supply other water uses 6. amount of water lost to unrecoverable sources (salt sink, saline aquifer, ocean, or unsaturated vadose zone). Irrigation Efficiencies - Water conveyance efficiency - Water application efficiency - Water distribution efficiency - Water use efficiency - Water storage efficiency - Consumptive use efficiency Water conveyance efficiency (Ec) The ratio between the water delivered to the farm and the water diverted from a river or reservoir expressed in percent Ec = (Wf / Wr) x 100 where: Wf = water delivered to the farm Wr = water diverted from the river or reservoir Some loss in conveyance is unavoidable. Losses may be greatly reduced by (1) lining earth ditches and canals or converting to pipelines and (2) by repairing and maintaining canals and pipelines, headgates, and other structures. Water application efficiency (Ea) The ratio between water stored in the soil root zone during irrigation and the water delivered to the farm expressed in percent Ea = (Ws / Wf) x 100 where: Ws = water stored in the soil root zone during irrigation Wf = water delivered to the farm Agricultural Buildings and Structures Basic structural elements 1. BEAMS - have longitudinal dimensions that are appreciably greater than their lateral dimensions - subjected to loads that are perpendicular to the longitudinal axis - usually in a horizontal position (i.e. floors, girders) - may also be in an inclined or vertical position (rafters in roof and studs) - BEAM cross-sections: rectangular, circular, or triangular OR it can be of what are referred to as STANDARD SECTIONS, such as Channel (C), Tee (T), Angle (L), and Wide flange (I). --- Types of Beams (a) Cantilever (b) Simply supported (c) Overhanging (d) Continuous (e) Fixed ended (f) Cantilever, simply supported 2. TENSION MEMBERS - slender members subjected to tensile stress 3. COMPRESSION MEMBERS - Vertical members that are subjected to axial compressive loads (i.e. columns) - Columns can be circular, square, or rectangular in their cross sections, and they can also be of Standard sections. 4. FRAMES - composed of vertical and horizontal members - Frames are classified as sway or non-sway. - A sway frame allows a lateral or sideward movement. The lateral movement of the sway frames are accounted for in their analysis. - A non-sway frame does not allow movement in the horizontal direction as it is sufficiently braced. Frames can also be classified as rigid or flexible. (1) Rigid frames have joints that are fixed (2) Flexible frames have moveable joints. 5. TRUSSES - are structural frameworks composed of straight members connected at the joints. - loads are applied at the joints. - straight members are connected at the joints using frictionless pins. Materials for construction 1. WOOD - one of the most common materials - QUALITIES: strong, durable, ease in fastening, lightweight, natural TIMBER - unprocessed wood LUMBER - wood that has been processed into uniform and useful sizes, including beams and planks or boards - is mainly used for construction framing, as well as finishing 2. CONCRETE - a solid, hard material produced by combining cement, fine aggregates (sand), coarse aggregates (gravel) and water - commonly used in farm buildings for footings, foundation walls, floors and pavements Uses of mortar - For bonding pieces of bricks/blocks together - Seals spaces between units - Ties steel reinforcements - Anchors bolts into the wall Proportioning of concrete Concrete Mixing ratio FULLER’s FORMULA Let: C = no. of bags of cement per m³ of concrete work S = volume of sand (m³) per m³ of concrete work G = volume of gravel (m³) per m³ of concrete work c, s, g = cement-sand-gravel ratio (relative amounts of solids by volume in a mixture) 55 𝐶= (𝑐 + 𝑠 + 𝑔) 𝑆 = 0.028 (𝐶)(𝑠) 𝐺 = 0.028 (𝐶)(𝑔) Example Find the quantity of Cement (C), Sand (S) and Gravel (G) needed for a slab that is 30 ft long, 30 ft wide and 0.5 ft thick. Use Class A mixture and Fuller's method. Req'd: C, S, G (Class A - 1:2:4) Sol'n: i) Calculate volume of concrete slab, VCW ii) Calculate C and Nbags iii) Calculate volume of sand iv) Calculate volume of gravel 3. CONCRETE HOLLOW BLOCKS (CHB) - most widely used masonry materials - main components for concrete wall laying which has a standard dimension (0.4m * 0.2m x CHB SIZE) rectangular block used in building construction - commonly used for walls, partitions, dividers, fences QUALITIES: - lightweight, fireproof, soundproof, heat-preserving - impermeable, earthquake-resistant, durable Typically, - A CHB has 3 whole cells and 2 one-half cells = 4 cells - Cell size vary depending on manufacturer - 1 m² = 12.5 pcs of CHB 4. GALVANIZED IRON (GI) SHEETS - most widely used roofing material QUALITIES: - Reasonable cost, readily available, durable - Ease of installation and repair Standard Commercial Forms 1. PLAIN – used for roofing, gutter, flashing, ridge, etc. [90 cm x 2.4 m] 2. CORRUGATED – for roofing and siding [80 cm x 1.5 m to 3.6 m] Lapping of Roof Sheet Side Lapping - 1 ½ corrugation – effective width covers 70 cm - 2 ½ corrugation – effective width covers 60 cm End lapping – ranges from 20 cm to 30 cm depending on the degree of slope and number of sheets in the longitudinal row AB Machinery Specifications, Testing, and Evaluation Engine Nomenclatures CLEARANCE VOLUME (Cᵥ)- volume of the cylinder when the piston is at top-dead- center (cm³ or cc) TOTAL VOLUME (V)- volume of cylinder when the piston is at the bottom-dead-center (cm³ or cc); sum of Pᵈ and Cᵥ. COMPRESSION RATIO- ratio of total volume with clearance volume 𝑃𝑑 + 𝐶𝑣 𝐶𝑟 = 𝐶𝑣 Where: Pᵈ = piston displacement (cc) Cᵥ = clearance volume (cc) Fuel Equivalent Power the equivalent power contained in fuel 𝑃𝑓𝑒 = 𝐻𝑉(𝑞)(𝑝) = 𝐻𝑉(𝑚) Where: HV = heating value (kJ/kg) q = consumption rate (L/h) ρ = density of fuel (kg/L) m = mass consumption rate (kg/h) Indicated Power Theoretical value of all the potential work due to the combustion of gases (𝑃)(𝑙)(𝐴)(𝑛)(𝑁) 𝑃𝑖 = (𝑘)(𝑐) Where: P = mean effective pressure l = stroke A = area of cylinder n = rotational speed (rpm) N = number of cylinders k = (2 for 4-stroke engines or 1 for 2-stroke engines) Mean Effective Pressure equivalent constant pressure developed at the top of the piston during the power stroke Brake Power Power available at the engine output shaft or flywheel for doing useful work 2𝜋(𝑇)(𝑛) 𝑃𝑏 = 𝑐 Where: T = torque developed at the crankshaft (N-m) n = rotational speed (rpm) c = conversion constant Horsepower (hp) - commonly used to indicate the work output of humans, draft animals, and mechanical prime movers - equals to 33000 lb-ft/min or 746 watts 1 horsepower = 746 W French hp (metric hp or PS) = 0.7355 kW = 0.9863 hp = 75 kg-m/s Prony Brake Dynamometer - The simplest form of torque meter - Consists of a band on one end of the lever that can be clamped to the rim of a pulley or flywheel - The other end of the lever is kept from turning by bearing on some kind of weight scale - As the pulley turns and as the clamp is tightened, the torque increases and the speed decreases - Power can be obtained by using the torque-speed formula Water Brake Dynamometer - the PTO shaft drives a pump which pumps water against a controlled restriction - In another type, the tractor PTO drives a rotating drum. - Hydraulically actuated brake shoes are applied inside the drum to produce torque. - A small hydraulic cylinder at the end of the arm transmits pressure to a large pressure gage, which is used to indicate the torque. INDICATED THERMAL EFFICIENCY fraction of fuel equivalent power that is converted to indicated power 𝑃𝑖 𝑒𝑖𝑡 = 𝑃𝑓𝑒 Where: 𝑃𝑖 = indicated power kW 𝑃𝑓𝑒 = fuel equivalent power in kW MECHANICAL EFFICIENCY fraction of indicated power that is delivered as useful power from the engine 𝑃𝑏 𝑒𝑚 = 𝑃𝑖 Where: 𝑃𝑏 = brake power in kW 𝑃𝑖 = indicated power kW Causes of Mechanical Loss 1. Frictional resistance between the piston and cylinder 2. Frictional resistance of crankshaft, camshaft, and other rotating parts 3. Power required for driving auxiliary parts such as oil pump, water pump, etc. Fuel Consumption The quantity of fuel consumed by the engine per unit time by volume or by mass Where: 𝑄𝑓 = fuel consumption rate by volume in L/h (gal/h) 𝑚𝑓 = fuel consumption rate by mass in kg/h (lb/h) 𝑃𝑓 = fuel density in kg/L (lb/gal) Engine Testing PAES 116:2001 Small Engine – Specifications PAES 117:2000 Small Engine – Methods of Test https://amtec.ceat.uplb.edu.ph/ PAES 116: 2001 Agricultural Machinery – Small Engine – Specifications This standard specifies the requirements for construction and performance of fully equipped internal combustion engines with one or two cylinders of up to 20 kW rating used for agricultural purposes. Performance Requirements - The engine shall be easily started as indicated in PAES 117. - At least 80% of the rated maximum output power shall be attained during the varying load test. - A performance curve, which shows output power, torque, fuel consumption and specific fuel consumption plotted against engine shaft speed at full throttle setting, shall be provided. - The rated continuous output power of the engine specified on the nameplate or 80% of the rated maximum power (if no rating on continuous power is indicated) shall be attained at rated engine speed. - Running-in - The engine to be tested shall be run-in prior to the test as recommended by the manufacturer. - Performance Tests - Test conditions - The test shall be conducted at full throttle for both spark-ignition and compression ignition engines. Performance Test - Field performance test - Size and field condition of test plot For upland field, the test plot shall be unplowed. For lowland field, the test plot shall be unplowed and soaked for at least 24 h with water level of 3 cm to 5 cm. Furthermore, the test plot shall be rectangular with sides in the ratio of 2:1 as much as possible. Its size shall not be less than 500 m². The total size of the prepared test plots shall be sufficient for the required number of test trials and running-in. If the test plots are not conforming to the recommended characteristics, the test shall be suspended. - Machine setting The machine’s depth of cut shall be set between 90 mm to 110 mm for plowing and rotary tilling. For plowing, the specifications of the disc plow to be used shall conform with PNS/PAES 167:2015 (Agricultural machinery – Disc plow).