Resp Physiology Past Paper PDF - DMU 2022
Document Details
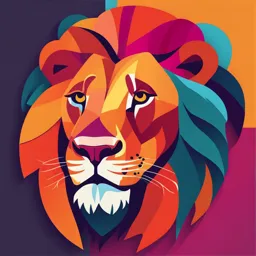
Uploaded by ManeuverableCreativity
DMU
Prof S Ghosh
Tags
Summary
This document details the respiratory physiology lecture notes from DMU. It covers topics such as respiration, ventilation, gas exchange, and pulmonary function.
Full Transcript
Respiratory Physiology Prof S Ghosh DMU Respiration The term respiration includes 3 separate functions: ▫ Ventilation: Breathing. ▫ Gas exchange: Between air and capillaries in the lungs. Between systemic capillaries and tissues of the body. ▫ 02utilization:...
Respiratory Physiology Prof S Ghosh DMU Respiration The term respiration includes 3 separate functions: ▫ Ventilation: Breathing. ▫ Gas exchange: Between air and capillaries in the lungs. Between systemic capillaries and tissues of the body. ▫ 02utilization: Cellular respiration. Ventilation Mechanical process that moves air in and out of the lungs. [O2]of air is higher in the lungs than in the blood, O2 diffuses from air to the blood. C02 moves from the blood to the air by diffusing down its concentration gradient. Gas exchange occurs entirely by diffusion: ▫ Diffusion is rapid because of the large surface area and the small diffusion distance. Alveoli Polyhedral in shape and clustered like units of honeycomb. ~ 300 million air sacs (alveoli). ▫ Large surface area (60–80 m2). ▫ Each alveolus is 1 cell layer thick. Total air barrier is 2 cells across (2 ∝m). 2 types of cells: ▫ Alveolar type I: Structural cells. ▫ Alveolar type II: Secrete surfactant. Respiratory Zone Region of gas exchange between air and blood. Includes respiratory bronchioles and alveolar sacs. Must contain alveoli. Conducting Zone All the structures air passes through before Insert fig. 16.5 reaching the respiratory zone. Warms and humidifies inspired air. Filters and cleans: ▫ Mucus secreted to trap particles in the inspired air. ▫ Mucus moved by cilia to be expectorated. Thoracic Cavity Diaphragm: ▫ Sheets of striated muscle divides anterior body cavity into 2 parts. Above diaphragm: thoracic cavity: ▫ Contains heart, large blood vessels, trachea, esophagus, thymus, and lungs. Below diaphragm: abdominopelvic cavity: ▫ Contains liver, pancreas, GI tract, spleen, and genitourinary tract. Intrapleural space: ▫ Space between visceral and parietal pleurae. Intrapulmonary and Intrapleural Pressures Visceral and parietal pleurae are flush against each other. ▫ The intrapleural space contains only a film of fluid secreted by the membranes. Lungs normally remain in contact with the chest walls. Lungs expand and contract along with the thoracic cavity. Intrapulmonary pressure: ▫ Intra-alveolar pressure (pressure in the alveoli). Intrapleural pressure: ▫ Pressure in the intrapleural space. ▫ Pressure is negative, due to lack of air in the intrapleural space. Transpulmonary Pressure Pressure difference across the wall of the lung. Intrapulmonary pressure – intrapleural pressure. ▫ Keeps the lungs against the chest wall. Intrapulmonary and Intrapleural Pressures (continued) During inspiration: ▫ Atmospheric pressure is > intrapulmonary pressure (-3 mm Hg). During expiration: ▫ Intrapulmonary pressure (+3 mm Hg) is > atmospheric pressure. Boyle’s Law Changes in intrapulmonary pressure occur as a result of changes in lung volume. ▫ Pressure of gas is inversely proportional to its volume. Increase in lung volume decreases intrapulmonary pressure. ▫ Air goes in. Decrease in lung volume, raises intrapulmonary pressure above atmosphere. ▫ Air goes out. Physical Properties of the Lungs Ventilation occurs as a result of pressure differences induced by changes in lung volume. Physical properties that affect lung function: ▫ Compliance. ▫ Elasticity. ▫ Surface tension. Compliance Distensibility (stretchability): ▫ Ease with which the lungs can expand. Change in lung volume per change in transpulmonary pressure. ∆V/∆P 100 x more distensible than a balloon. ▫ Compliance is reduced by factors that produce resistance to distension. Elasticity Tendency to return to initial size after distension. High content of elastin proteins. ▫ Very elastic and resist distension. Recoil ability. Elastic tension increases during inspiration and is reduced by recoil during expiration. Surface Tension Force exerted by fluid in alveoli to resist distension. Lungs secrete and absorb fluid, leaving a very thin film of fluid. ▫ This film of fluid causes surface tension. ▫ Fluid absorption is driven (osmosis) by Na+active transport. ▫ Fluid secretion is driven by the active transport of Cl- out of the alveolar epithelial cells. H20 molecules at the surface are attracted to other H20 molecules by attractive forces. ▫ Force is directed inward, raising pressure in alveoli. Surface Tension (continued) Law of Laplace: ▫ Pressure in alveoli is Insert fig. 16.11 directly proportional to surface tension; and inversely proportional to radius of alveoli. ▫ Pressure in smaller alveolus would be greater than in larger alveolus, if surface tension were the same in both. Surfactant Phospholipid produced by alveolar type II cells. Insert fig. 16.12 Lowers surface tension. ▫ Reduces attractive forces of hydrogen bonding by becoming interspersed between H20 molecules. Surface tension in alveoli is reduced. As alveoli radius decreases, surfactant’s ability to lower surface tension increases. Disorders: ▫ RDS. ▫ ARDS. Quiet Inspiration Active process: ▫ Contraction of diaphragm, increases thoracic volume vertically. Parasternal and external intercostals contract, raising the ribs; increasing thoracic volume laterally. Pressure changes: ▫ Alveolar changes from 0 to –3 mm Hg. ▫ Intrapleural changes from –4 to –6 mm Hg. ▫ Transpulmonary pressure = +3 mm Hg. Expiration Quiet expiration is a passive process. ▫ After being stretched by contractions of the diaphragm and thoracic muscles; the diaphragm, thoracic muscles, thorax, and lungs recoil. ▫ Decrease in lung volume raises the pressure within alveoli above atmosphere, and pushes air out. Pressure changes: ▫ Intrapulmonary pressure changes from –3 to +3 mm Hg. ▫ Intrapleural pressure changes from –6 to –3 mm Hg. ▫ Transpulmonary pressure = +6 mm Hg. Pulmonary Ventilation Insert fig. 16.15 Pulmonary Function Tests Assessed by spirometry. Subject breathes into a closed system in which air is trapped within a bell floating in H20. The bell moves up when the subject exhales and down when the subject inhales. Insert fig. 16.16 Terms Used to Describe Lung Volumes and Capacities Anatomical Dead Space Not all of the inspired air reached the alveoli. As fresh air is inhaled it is mixed with air in anatomical dead space. ▫ Conducting zone and alveoli where is lower than normal and [C02] is higher than normal. Alveolar ventilation = F x (TV- DS). ▫ F = frequency (breaths/min.). ▫ TV = tidal volume. ▫ DS = dead space. Restrictive and Obstructive Disorders Restrictive disorder: ▫ Vital capacity is reduced. Insert fig. 16.17 ▫ FVC is normal. Obstructive disorder: ▫ Diagnosed by tests that measure the rate of expiration. VC is normal. FEV1 is < 80%. Pulmonary Disorders Dyspnea: ▫ Shortness of breath. COPD (chronic obstructive pulmonary disease): ▫ Asthma: Obstructive air flow through bronchioles. Caused by inflammation and mucus secretion. ▫ Inflammation contributes to increased airway responsiveness to agents that promote bronchial constriction. ▫ IgE, exercise. Pulmonary Disorders (continued) ▫ Emphysema: Alveolar tissue is destroyed. Chronic progressive condition that reduces surface area for gas exchange. Decreases ability of bronchioles to remain open during expiration. ▫ Cigarette smoking stimulates macrophages and leukocytes to secrete protein digesting enzymes that destroy tissue. Pulmonary fibrosis: ▫ Normal structure of lungs disrupted by accumulation of fibrous connective tissue proteins. Anthracosis. Gas Exchange in the Lungs Dalton’s Law: ▫ Total pressure of a gas mixture is = to the sum of the pressures that each gas in the mixture would exert independently. Partial pressure: ▫ The pressure that an particular gas exerts independently. PATM= PN + P0 + PC0 + PH 0= 760 mm Hg. 2 2 2 2 ▫ 02 is humidified = 105 mm Hg. H20 contributes to partial pressure (47 mm Hg). P0 (sea level) = 150 mm Hg. 2 ▫ PC0 = 40 mm Hg. 2 Partial Pressures of Gases in Inspired Air and Alveolar Air Insert fig. 16.20 Partial Pressures of Gases in Blood When a liquid or gas (blood and alveolar air) are at equilibrium: ▫ The amount of gas dissolved in fluid reaches a maximum value (Henry’s Law). Depends upon: ▫ Solubility of gas in the fluid. ▫ Temperature of the fluid. ▫ Partial pressure of the gas. [Gas] dissolved in a fluid depends directly on its partial pressure in the gas mixture. Significance of Blood P0 and PC0 2 2 Measurements At normal P0 arterial 2 blood is about 100 mm Hg. P0 level in 2 the systemic veins is about 40 mm Hg. ν PC0 is 46 mm Hg in the systemic veins. 2 Provides a good index of lung function. ν Pulmonary Circulation Rate of blood flow through the pulmonary circulation is = flow rate through the systemic circulation. ▫ Driving pressure is about 10 mm Hg. Pulmonary vascular resistance is low. ▫ Low pressure pathway produces less net filtration than produced in the systemic capillaries. Avoids pulmonary edema. Autoregulation: ▫ Pulmonary arterioles constrict when alveolar P0 2 decreases. ▫ Matches ventilation/perfusion ratio. Pulmonary Circulation (continued) In a fetus: ▫ Pulmonary circulation has a higher vascular resistance, because the lungs are partially collapsed. After birth, vascular resistance decreases: ▫ Opening the vessels as a result of subatmospheric intrapulmonary pressure. ▫ Physical stretching of the lungs. ▫ Dilation of pulmonary arterioles in response to increased alveolar P0. 2 Lung Ventilation/Perfusion Ratios Functionally: Insert fig. 16.24 ▫ Alveoli at apex are underperfused (overventilated). ▫ Alveoli at the base are underventilated (overperfused). Disorders Caused by High Partial Pressures of Gases Nitrogen narcosis: ▫ At sea level nitrogen is physiologically inert. ▫ Under hyperbaric conditions: Nitrogen dissolves slowly. Can have deleterious effects. ▫ Resembles alcohol intoxication. Decompression sickness: ▫ Amount of nitrogen dissolved in blood as a diver ascends decreases due to a decrease in PN. 2 If occurs rapidly, bubbles of nitrogen gas can form in tissues and enter the blood. Block small blood vessels producing the “bends.” Brain Stem Respiratory Centers Neurons in the reticular formation of the medulla Insert fig. 16.25 oblongata form the rhythmicity center: ▫ Controls automatic breathing. ▫ Consists of interacting neurons that fire either during inspiration (I neurons) or expiration (E neurons). Brain Stem Respiratory Centers (continued) I neurons project to, and stimulate spinal motor neurons that innervate respiratory muscles. Expiration is a passive process that occurs when the I neurons are inhibited. Activity varies in a reciprocal way. Rhythmicity Center I neurons located primarily in dorsal respiratory group (DRG): ▫ Regulate activity of phrenic nerve. Project to and stimulate spinal interneurons that innervate respiratory muscles. E neurons located in ventral respiratory group (VRG): ▫ Passive process. Controls motor neurons to the internal intercostal muscles. Activity of E neurons inhibit I neurons. ▫ Rhythmicity of I and E neurons may be due to pacemaker neurons. Pons Respiratory Centers Activities of medullary rhythmicity center is influenced by pons. Apneustic center: ▫ Promotes inspiration by stimulating the I neurons in the medulla. Pneumotaxic center: ▫ Antagonizes the apneustic center. ▫ Inhibits inspiration. Chemoreceptors 2 groups of chemo- receptors that monitor changes in blood PC0 , 2 Insert fig. 16.27 P0 , and pH. 2 Central: ▫ Medulla. Peripheral: ▫ Carotid and aortic bodies. Control breathing indirectly via sensory nerve fibers to the medulla (X, IX). Effects of Blood PC0 and pH on Ventilation 2 Chemoreceptor input modifies the rate and depth of breathing. ▫ Oxygen content of blood decreases more slowly because of the large “reservoir” of oxygen attached to hemoglobin. ▫ Chemoreceptors are more sensitive to changes in PC0. 2 H20 + C02 H2C03 H+ + HC03- Rate and depth of ventilation adjusted to maintain arterial PC0 of 40 mm Hg. 2 Chemoreceptor Control Central chemoreceptors: ▫ More sensitive to changes in arterial PC0. 2 H20 + C02 H 2C03 H+ H+ cannot cross the blood brain barrier. C02 can cross the blood brain barrier and will form H2C03. ▫ Lowers pH of CSF. Directly stimulates central chemoreceptors. Chemoreceptor Control (continued) Peripheral chemoreceptors: ▫ Are not stimulated directly by changes in arterial PC0. 2 H20 + C02 H2C03 H+ Stimulated by rise in [H+] of arterial blood. ▫ Increased [H+] stimulates peripheral chemoreceptors. Chemoreceptor Control of Breathing Insert fig. 16.29 Effects of Blood P0 on Ventilation 2 Blood P0 affected by breathing indirectly. 2 ▫ Influences chemoreceptor sensitivity to changes in PC0. 2 Hypoxic drive: ▫ Emphysema blunts the chemoreceptor response to PC0. 2 ▫ Choroid plexus secrete more HC03- into CSF, buffering the fall in CSF pH. ▫ Abnormally high PC0 enhances sensitivity of carotid 2 bodies to fall in P0. 2 Effects of Pulmonary Receptors on Ventilation Lungs contain receptors that influence the brain stem respiratory control centers via sensory fibers in vagus. ▫ Unmyelinated C fibers can be stimulated by: Capsaicin: Produces apnea followed by rapid, shallow breathing. Histamine and bradykinin: Released in response to noxious agents. ▫ Irritant receptors are rapidly adaptive receptors. Hering-Breuer reflex: ▫ Pulmonary stretch receptors activated during inspiration. Inhibits respiratory centers to prevent undue tension on lungs. Hemoglobin and 02 Transport 280 million hemoglobin/RBC. Insert fig. 16.32 Each hemoglobin has 4 polypeptide chains and 4 hemes. In the center of each heme group is 1 atom of iron that can combine with 1 molecule 02. Hemoglobin Oxyhemoglobin: ▫ Normal heme contains iron in the reduced form (Fe2+). ▫ Fe2+ shares electrons and bonds with oxygen. Deoxyhemoglobin: ▫ When oxyhemoglobin dissociates to release oxygen, the heme iron is still in the reduced form. ▫ Hemoglobin does not lose an electron when it combines with 02. Hemoglobin (continued) Methemoglobin: ▫ Has iron in the oxidized form (Fe3+). Lacks electrons and cannot bind with 02. Blood normally contains a small amount. Carboxyhemoglobin: ▫ The reduced heme is combined with carbon monoxide. ▫ The bond with carbon monoxide is 210 times stronger than the bond with oxygen. Transport of 02 to tissues is impaired. Hemoglobin (continued) Oxygen-carrying capacity of blood determined by its [hemoglobin]. ▫ Anemia: [Hemoglobin] below normal. ▫ Polycythemia: [Hemoglobin] above normal. ▫ Hemoglobin production controlled by erythropoietin. Production stimulated by PC0 delivery to kidneys. 2 Loading/unloading depends: ▫ P0 of environment. 2 ▫ Affinity between hemoglobin and 02. Oxyhemoglobin Dissociation Curve Graphic illustration of the % oxyhemoglobin saturation at different values of P0. 2 ▫ Loading and unloading of 02. Steep portion of the sigmoidal curve, small changes in P0 2 produce large differences in % saturation (unload more 02). Decreased pH, increased temperature, and increased 2,3 DPG: ▫ Affinity of hemoglobin for 02 decreases. Greater unloading of 02: Shift to the curve to the right. Oxyhemoglobin Dissociation Curve Insert fig.16.34 Effects of pH and Temperature The loading and unloading of O2 influenced by the Insert fig. 16.35 affinity of hemoglobin for 02. Affinity is decreased when pH is decreased. Increased temperature and 2,3-DPG: ▫ Shift the curve to the right. Effect of 2,3 DPG on 02Transport Anemia: ▫ RBCs total blood [hemoglobin] falls, each RBC produces greater amount of 2,3 DPG. Since RBCs lack both nuclei and mitochondria, produce ATP through anaerobic metabolism. Fetal hemoglobin (hemoglobin f): ▫ Has 2 γ-chains in place of the β-chains. Hemoglobin f cannot bind to 2,3 DPG. Has a higher affinity for 02. Inherited Defects in Hemoglobin Structure and Function Sickle-cell anemia: ▫ Hemoglobin S differs in that valine is substituted for glutamic acid on position 6 of the β chains. Cross links form a “paracrystalline gel” within the RBCs. Makes the RBCs less flexible and more fragile. Thalassemia: ▫ Decreased synthesis of α or β chains, increased synthesis of γ chains. Muscle Myoglobin Red pigment found exclusively in striated muscle. Insert fig. 13.37 ▫ Slow-twitch skeletal fibers and cardiac muscle cells are rich in myoglobin. Have a higher affinity for 02 than hemoglobin. ▫ May act as a “go- between” in the transfer of 02 from blood to the mitochondria within muscle cells. ν May also have an 02 storage function in cardiac muscles. C02 Transport C02 transported in the blood: ▫ HC03- (70%). ▫ Dissolved C02(10%). ▫ Carbaminohemoglobin (20%). H20 + C02 ca H2C03 High PC0 2 Chloride Shift at Systemic Capillaries H20 + C02 H2C03 H+ + HC03- At the tissues, C02 diffuses into the RBC; shifts the reaction to the right. ▫ Increased [HC03-] produced in RBC: HC03-diffuses into the blood. ▫ RBC becomes more +. Cl- attracted in (Cl- shift). ▫ H+ released buffered by combining with deoxyhemoglobin. HbC02 formed. ▫ Unloading of 02. Carbon Dioxide Transport and Chloride Shift Insert fig. 16.38 At Pulmonary Capillaries H20 + C02 H2C03 H+ + HC03- At the alveoli, C02 diffuses into the alveoli; reaction shifts to the left. Decreased [HC03-] in RBC, HC03- diffuses into the RBC. ▫ RBC becomes more -. Cl-diffuses out (reverse Cl- shift). Deoxyhemoglobin converted to oxyhemoglobin. ▫ Has weak affinity for H+. Gives off HbC02. Reverse Chloride Shift in Lungs Insert fig. 16.39 Respiratory Acid-Base Balance Ventilation normally adjusted to keep pace with metabolic rate. H2CO3 produced converted to CO2, and excreted by the lungs. H20 + C02 H2C03 H++ HC03- Respiratory Acidosis Hypoventilation. Accumulation of CO2in the tissues. ▫ Pc02 increases. ▫ pH decreases. ▫ Plasma HCO3- increases. Respiratory Alkalosis Hyperventilation. Excessive loss of CO2. ▫ Pc02 decreases. ▫ pH increases. ▫ Plasma HCO3- decreases. Effect of Bicarbonate on Blood pH Insert fig. 16.40 Ventilation During Exercise During exercise, breathing becomes deeper and more rapid. Produce > total minute volume. Insert fig. 16.41 Neurogenic mechanism: ▫ Sensory nerve activity from exercising muscles stimulates the respiratory muscles. ▫ Cerebral cortex input may stimulate brain stem centers. Humoral mechanism: ▫ PC0 and pH may be different 2 at chemoreceptors. ▫ Cyclic variations in the values that cannot be detected by blood samples. Lactate Threshold and Endurance Training Maximum rate of oxygen consumption that can be obtained before blood lactic acid levels rise as a result of anaerobic respiration. ▫ 50-70% maximum 02 uptake has been reached. Endurance trained athletes have higher lactate threshold, because of higher cardiac output. ▫ Have higher rate of oxygen delivery to muscles. ▫ Have increased content of mitochondria in skeletal muscles. Acclimatization to High Altitude Adjustments in respiratory function when moving to an area with higher altitude: Changes in ventilation: ▫ Hypoxic ventilatory response produces hyperventilation. Increases total minute volume. Increased tidal volume. Affinity of hemoglobin for 02: ▫ Action of 2,3-DPG decreases affinity of hemoglobin for 02. Increased hemoglobin production: ▫ Kidneys secrete erythropoietin.