CV 232 PDF - Basic Biology of the Cardiovascular System
Document Details
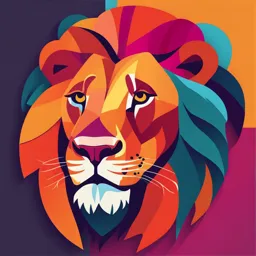
Uploaded by SimplestPhotorealism
Michigan State University
Joseph Loscalzo, Peter Libby, Calum A. MacRae
Tags
Summary
This document provides a comprehensive overview of the basic biology of the cardiovascular system. It covers developmental aspects, vascular structure, and function, and cardiac cell biology. The document also includes information on disease prevention and management.
Full Transcript
physical examination, and other features of the cardiac examination, as intervals for repeated examinations. If there is no evidence of dis- 1651 described in Chap. 234. ease, such continued attention may lead to the pat...
physical examination, and other features of the cardiac examination, as intervals for repeated examinations. If there is no evidence of dis- 1651 described in Chap. 234. ease, such continued attention may lead to the patient’s developing The majority of heart murmurs are midsystolic and soft inappropriate concern about the possibility of heart disease. (grades I–II/VI). When such a murmur occurs in an asymptomatic 2. If there is no evidence of cardiovascular disease but the patient child or young adult without other evidence of heart disease on clinical has one or more risk factors for the development of ischemic heart CHAPTER 232 Basic Biology of the Cardiovascular System examination, it is usually benign and echocardiography generally is not disease (Chap. 267), a plan for their reduction should be developed required. By contrast, two-dimensional and Doppler echocardiography and the patient should be retested at intervals to assess compliance (Chap. 236) are indicated in patients with loud systolic murmurs and efficacy in risk reduction. (grades ≥III/VI), especially those that are holosystolic or late systolic, 3. Asymptomatic or mildly symptomatic patients with valvular heart and in most patients with diastolic or continuous murmurs. disease that is anatomically severe should be evaluated periodically, every 6 to 12 months, by clinical and noninvasive examinations. PITFALLS IN CARDIOVASCULAR MEDICINE Early signs of deterioration of ventricular function may signify the Increasing subspecialization in internal medicine and the perfection need for surgical treatment before the development of disabling of advanced diagnostic techniques in cardiology can lead to several symptoms, irreversible myocardial damage, and excessive risk of undesirable consequences. Examples include the following: surgical treatment (Chap. 256). 1. Failure by the noncardiologist to recognize important cardiac mani- 4. In patients with CAD (Chap. 267), available practice guidelines festations of systemic illnesses. For example, the presence of mitral should be considered in the decision on the form of treatment (med- stenosis, patent foramen ovale, and/or transient atrial arrhythmia ical, percutaneous coronary intervention, or surgical revasculariza- should be considered in a patient with stroke, or the presence of tion). Mechanical revascularization may be employed too frequently pulmonary hypertension and cor pulmonale should be considered in the United States and too infrequently in Eastern Europe and in a patient with scleroderma or Raynaud’s syndrome. A cardiovas- developing nations. The mere presence of angina pectoris and/or the cular examination should be carried out to identify and estimate the demonstration of critical coronary arterial narrowing at angiography severity of the cardiovascular involvement that accompanies many should not reflexively evoke a decision to treat the patient by revascu- noncardiac disorders. larization. Instead, these interventions should be limited to patients 2. Failure by the cardiologist to recognize underlying systemic disor- with CAD whose angina has not responded adequately to medical ders in patients with heart disease. For example, hyperthyroidism treatment or in whom revascularization has been shown to improve should be considered in an elderly patient with atrial fibrillation and the natural history (e.g., acute coronary syndrome or multivessel unexplained heart failure, and Lyme disease should be considered CAD with left ventricular dysfunction). in a patient with unexplained fluctuating atrioventricular block. A cardiovascular abnormality may provide the clue critical to the rec- ognition of some systemic disorders. For example, an unexplained 232 Basic Biology of the pericardial effusion may provide an early clue to the diagnosis of tuberculosis or a neoplasm. 3. Overreliance on and overutilization of laboratory tests, particularly invasive techniques, for the evaluation of the cardiovascular system. Cardiovascular System Cardiac catheterization and coronary arteriography (Chap. 237) Joseph Loscalzo, Peter Libby, Calum A. MacRae provide precise diagnostic information that may be crucial in devel- oping a therapeutic plan in patients with known or suspected CAD. Although a great deal of attention has been directed to these exami- nations, it is important to recognize that they serve to supplement, not DEVELOPMENTAL BIOLOGY OF THE supplant, a careful examination carried out with clinical and nonin- CARDIOVASCULAR SYSTEM vasive techniques. A coronary arteriogram should not be performed The heart forms early during embryogenesis (Fig. 232-1), circulating in lieu of a careful history in patients with chest pain suspected of blood, nutrients, and oxygen to the other developing organs while having ischemic heart disease. Although coronary arteriography continuing to grow and undergo complex morphogenetic changes. may establish whether the coronary arteries are obstructed and to Early cardiac progenitors arise within crescent-shaped fields of lateral what extent, the results of the procedure by themselves often do not splanchnic mesoderm under the influence of multiple signals and provide a definitive answer to the question of whether a patient’s migrate to the midline to form the linear heart tube: a single layer of complaint of chest discomfort is attributable to coronary atheroscle- endocardium and a single layer of cardiomyocyte precursors. rosis and whether or not revascularization is indicated. The linear heart tube undergoes asymmetric looping, that coordinates with chamber specification and multilayer growth of different regions Despite the value of invasive tests in certain circumstances, they of the heart tube to produce the presumptive atria and ventricles. Cells entail some small risk to the patient, involve discomfort and substantial continue to migrate into the heart at both ends from later, or second, cost, and place a strain on medical facilities. Therefore, they should be heart fields in pharyngeal mesoderm as looping and growth occur. carried out only if the results can be expected to modify the patient’s These cells exhibit distinctive gene expression (e.g., Islet-1) and dis- management. tinctive physiology (e.g., calcium handling), contributing to discrete DISEASE PREVENTION AND MANAGEMENT areas of the adult heart, including the right atrium and the right ven- The prevention of heart disease, especially of CAD, is one of the most tricle. Different embryologic origins of cells within the right and left important tasks of primary health care givers as well as cardiologists. ventricles help explain why some forms of congenital and adult heart Prevention begins with risk assessment, followed by attention to life- diseases affect regions of the heart to varying degrees. style, such as achieving optimal weight, physical activity, and smoking After looping and chamber formation, a series of morphogenetic cessation, and then aggressive treatment of all abnormal risk factors, events divide the left and right sides of the heart, separate the atria such as hypertension, hyperlipidemia, and diabetes mellitus (Chap. 396). from the ventricles, and form the aorta and pulmonary artery from After a complete diagnosis has been established in patients with the truncus arteriosus. Cardiac valves form between the atria and the known heart disease, a number of management options are usually ventricles and between the ventricles and the outflow vessels. Early in available. Several examples may be used to demonstrate some of the development, the single layer of myocardial cells secretes an extracellu- principles of cardiovascular therapeutics: lar matrix rich in hyaluronic acid, or “cardiac jelly,” which accumulates within the endocardial cushions, precursors of the cardiac valves. Sig- 1. In the absence of evidence of heart disease, the patient should be nals from overlying myocardial cells trigger migration, invasion, and clearly informed of this assessment and not be asked to return at phenotypic changes in underlying endocardial cells, which undergo Harrisons_20e_Part6_p1649-p1942.indd 1651 6/1/18 12:48 PM 1652 Early heart-forming Neural folds Pericardial Foregut Forming heart and are required for proper coronary pat- regions coelom terning. Other cell types within the heart, (e.g., fibroblasts) also can arise from the proepicardium. The cardiac conduction system, which generates and propagates elec- PART 6 trical impulses, differentiates from car- diomyocyte precursors. The conduction system is composed of slow-conduct- A B ing (proximal) components, such as the Disorders of the Cardiovascular System sinoatrial (SA) and atrioventricular (AV) First heart field Second heart field nodes, as well as fast-conducting (distal) components, including the His bundle, bundle branches, and Purkinje fibers. Precursors within the sinus venosus give rise to the SA node, whereas those RA LA within the AV canal mature into hetero- geneous cell types that compose the AV node. So-called decremental conduction RV through the AV node delays the electrical LV LV impulses between atria and ventricles, whereas the distal conduction system RV rapidly delivers the impulse throughout C D E the ventricles. Each compartment within the conduction system expresses distinct gap junction proteins and ion channels that characterize the discrete cell fates and electrical properties. Developmental defects in the conduction system can lead to clinical electrophysiologic disorders, such as congenital heart block or pre- excitation (Wolff-Parkinson-White syn- drome) (Chap. 241). ORIGIN OF VASCULAR CELLS F As noted above, smooth-muscle cells in various types of artery derive from dif- FIGURE 232-1 A. Schematic depiction of a transverse section through an early embryo depicts the bilateral regions ferent sources. Some upper-body arte- where early heart tubes form. B. The bilateral heart tubes subsequently migrate to the midline and fuse to form the rial smooth-muscle cells derive from the linear heart tube. C. At the early cardiac crescent stage of embryonic development, cardiac precursors include a neural crest, whereas lower-body arter- primary heart field fated to form the linear heart tube and a second heart field fated to add myocardium to the inflow ies generally recruit smooth-muscle cells and outflow poles of the heart. D. Second heart field cells populate the pharyngeal region before subsequently from neighboring mesodermal structures migrating to the maturing heart. E. Large portions of the right ventricle and outflow tract and some cells within the atria derive from the second heart field. F. The aortic arch arteries form as symmetric sets of vessels that then during development. Bone marrow– remodel under the influence of the neural crest to form the asymmetric mature vasculature. LA, left atrium; LV, left derived endothelial progenitors may aid ventricle; RA, right atrium; RV, right ventricle. repair of damaged or aging arteries. In addition, multipotent vascular stem cells an epithelial-mesenchymal transformation to invade and populate the resident in vessel walls may give rise to the smooth-muscle cells that endocardial cushion matrix with cells. Mesenchymal cells then prolifer- accumulate in injured or atheromatous arteries (Chaps. 92 and 473). ate and form the mature valve leaflets. The great vessels form as a series of bilaterally symmetric aortic THE BLOOD VESSEL arch arteries that remodel asymmetrically to define the mature central vasculature. Migrating neural crest cells from the dorsal neural tube VASCULAR ULTRASTRUCTURE orchestrate this process and are necessary for aortic arch remodeling Blood vessels participate in physiologic function and play roles in disease and the septation of the truncus arteriosus. The smooth-muscle cells biology in virtually every organ system. The smallest blood vessels— within the tunica media of the aortic arch, the ductus arteriosus, and the capillaries—consist of a monolayer of endothelial cells on a basement carotid arteries all derive from neural crest. By contrast, smooth-muscle membrane, adjacent to a discontinuous layer of smooth-muscle-like cells within the descending aorta arises from lateral plate mesoderm, and known as pericytes (Fig. 232-2A). Arteries typically have a trilaminar smooth muscle of the proximal outflow tract arises from the second structure (Fig. 232-2B–E). The intima consists of a monolayer of endo- heart field. Neural crest cells are sensitive to both vitamin A and folic thelial cells continuous with those of the capillaries. The middle layer, acid, and congenital heart disease involving abnormal remodeling of or tunica media, consists of smooth-muscle cells, in veins, the media can the aortic arch arteries can associate with maternal deficiencies of these contain just a few layers of smooth-muscle cells (Fig. 232-2B). The outer vitamins. The shared embryologic origins of different cardiovascular layer, the adventitia, consists of looser extracellular matrix with fibrob- cell types lead to syndromic associations between various congenital lasts, mast cells, and nerve terminals. Larger arteries have their own heart diseases and a range of extracardiac abnormalities. vasculature, the vasa vasorum, which nourishes the tunica media. Coronary artery formation requires the addition of yet another cell Arteriolar muscle tone regulates blood pressure and flow through population to the embryonic heart. Epicardial cells arise in the pro- arterial beds (Fig. 232-2C). Medium-size muscular arteries also con- epicardial organ, a derivative of the septum transversum, which also tain prominent smooth muscle layers (Fig. 232-2D) that participate in contributes to the fibrous portion of the diaphragm and to the liver. atherosclerosis. Larger elastic arteries have a highly structured tunica Proepicardial cells contribute smooth-muscle to the coronary arteries media with concentric bands of smooth-muscle cells, interspersed with Harrisons_20e_Part6_p1649-p1942.indd 1652 6/1/18 12:48 PM A. Capillary B. Vein C. Small muscular artery exposure to angiotensin II), can promote 1653 Pericyte local oxidative stress and inactivate NO. Normal endothelium exhibits lim- ited interaction with circulating leuko- cytes, but when activated by bacterial CHAPTER 232 Basic Biology of the Cardiovascular System Vascular products such as endotoxin or by proin- smooth-muscle cell flammatory cytokines released during Endothelial cell infection or injury, endothelial cells express an array of adhesion molecules that selectively bind various classes of leukocytes in different pathologic conditions. The adhesion molecules D. Large muscular artery E. Large elastic artery and chemokines generated during acute bacterial infection tend to recruit granulocytes, while in chronic inflam- Internal elastic matory diseases such as tuberculosis lamina or atherosclerosis, the adhesion mole- cules expressed favor monocyte recruit- External elastic ment. Endothelial cells participate in lamina the pathophysiology of many immune- mediated diseases. Complement- mediated lysis of endothelial cells is Adventitia an example of immunologically medi- ated tissue injury. The presentation of foreign histocompatibility complex antigens by endothelial cells in solid-or- gan allografts can promote allograft FIGURE 232-2 Schematics of the structures of various types of blood vessels. A. Capillaries consist of an arteriopathy, while immune-mediated endothelial tube in contact with a discontinuous population of pericytes. B. Veins typically have thin medias and endothelial injury also plays a role in thicker adventitias. C. A small muscular artery features a prominent tunica media. D. Larger muscular arteries have thrombotic thrombocytopenic purpura a prominent media with smooth-muscle cells embedded in a complex extracellular matrix. E. Larger elastic arteries or hemolytic-uremic syndrome. have cylindrical layers of elastic tissue alternating with concentric rings of smooth-muscle cells. The endothelium also regulates the balance between thrombosis and hemo- strata of elastin-rich extracellular matrix (Fig. 232-2E). Larger arteries stasis through a highly tuned set of regulatory pathways. When acti- form an internal elastic lamina between intima and media while an vated by inflammatory cytokines, bacterial endotoxin, or angiotensin external elastic lamina partitions media from surrounding adventitia. II, for example, endothelial cells can produce substantial quantities of the major inhibitor of fibrinolysis, plasminogen activator inhibitor VASCULAR CELL BIOLOGY 1 (PAI-1). Thus, in pathologic circumstances, the endothelial cell may promote local thrombus accumulation rather than combat it. Inflam- Endothelial Cell The endothelium forms the interface between matory stimuli also induce the expression of the potent procoagulant tissues and the blood compartment, regulating the passage of mole- tissue factor, a contributor to disseminated intravascular coagulation cules and cells. The ability of endothelial cells to serve as a selectively in sepsis. permeable barrier fails in vascular diseases, including atherosclerosis, Endothelial cells regulate the growth of subjacent smooth-muscle hypertension, and renal disease, as well as in pulmonary edema, sepsis cells. For example, heparan sulfate glycosaminoglycans elaborated and other situations of “capillary leak.” by endothelial cells can inhibit smooth-muscle proliferation, and in The endothelium also participates in the local regulation of vascular the setting of injury endothelial cells produce growth factors and tone and blood flow. Endogenous substances produced by endothelial chemoattractants, such as platelet-derived growth factor, which cause cells such as prostacyclin, endothelium-derived hyperpolarizing factor, the migration and proliferation of vascular smooth-muscle cells. nitric oxide (NO), and hydrogen peroxide (H2O2) provide tonic vasodila- Dysregulation of these growth-stimulatory molecules may promote tory stimuli under physiologic conditions in vivo (Table 232-1). Impaired smooth-muscle accumulation in atherosclerotic lesions. production or excess catabolism of NO impairs endothelium-dependent vasodilator function contributing to pathologic vasoconstriction. Mea- Vascular Smooth-Muscle Cell Contraction and relaxation of surement of flow-mediated dilatation can assess endothelial vasodilator vascular smooth-muscle cells in muscular arteries determines blood function in humans (Fig. 232-3). Endothelial cells also produce potent pressure, regional flow and the afterload experienced by the left ven- vasoconstrictor substances such as endothelin. Excessive production of tricle (see below). Venous tone regulates the capacitance of the venous reactive oxygen species, such as superoxide anion (O2–), by endothelial tree and so influences ventricular preload. Smooth-muscle cells in or smooth-muscle cells under pathologic conditions (e.g., excessive the adult vessel seldom replicate in the absence of arterial injury or inflammatory activation, but proliferation and migration of arterial smooth-muscle cells contributes to arterial stenoses in atherosclerosis, TABLE 232-1 Endothelial Functions in Health and Disease arteriolar remodeling in hypertension, and the hyperplastic response HOMEOSTATIC PROPERTIES DYSFUNCTIONAL PROPERTIES of arteries injured by percutaneous intervention. In the pulmonary Optimize balance between Impaired dilation, vasoconstriction circulation, smooth-muscle migration and proliferation underlie the vasodilation and vasoconstriction vascular disease that occurs in sustained high-flow states such as left- Antithrombotic, profibrinolytic Prothrombotic, antifibrinolytic to-right shunts in congenital heart disease. Anti-inflammatory Proinflammatory Smooth-muscle cells secrete the bulk of vascular extracellular Antiproliferative Proproliferative matrix. Excessive production of collagen and glycosaminoglycans Antioxidant Prooxidant contributes to the remodeling, altered biomechanics and physiology of arteries affected by hypertension or atherosclerosis. In larger elastic Permselectivity Impaired barrier function arteries, such as the aorta, the ability to store the kinetic energy of Harrisons_20e_Part6_p1649-p1942.indd 1653 6/1/18 12:48 PM 1654 rises due to transmembrane influx and triggered release from intra- cellular calcium stores (Fig. 232-4). In vascular smooth-muscle cells, voltage-dependent L-type calcium channels open with membrane depolarization. Local changes in intracellular calcium concentration, termed calcium sparks, can trigger release from intracellular stores which results in more contraction and higher vessel tone (see below). PART 6 Opposing currents balance the effects of individual ionic fluxes, pro- moting homeostasis which is tightly regulated by neural and metabolic influences. Biochemical agonists also increase intracellular [Ca2+] by various Disorders of the Cardiovascular System mechanisms including receptor-dependent phospholipase C activation with hydrolysis of phosphatidylinositol 4,5-bisphosphate to generate diacylglycerol (DAG) and inositol 1,4,5-trisphosphate (IP3). These membrane lipid derivatives in turn activate protein kinase C and increase intracellular [Ca2+]. In addition, IP3 binds specific sarcoplasmic reticulum (SR) receptors to increase calcium efflux from this storage pool into the cytoplasm. Vascular smooth-muscle cell contraction depends on myosin light chain phosphorylation, which in the steady state reflects the balance between the actions of the relevant kinases and phosphatases. Calcium activates myosin light chain kinase via calmodulin, augmenting myo- sin ATPase activity enhancing contraction. Myosin light chain phos- phatase conversely reduces myosin ATPase activity and contractile force. Other kinase/phosphorylase combinations result in a complex regulatory network that refines vascular tone and links it to physiologic requirements. Control of Vascular Smooth-Muscle Cell Tone The auto- nomic nervous system and endothelial cells modulate vascular smooth-muscle cells through similar convergent pathways. Autonomic neurons enter vessel media and modulate vascular smooth-muscle cell tone in response to baroreceptors and chemoreceptors within the aortic arch or carotid bodies and to thermoreceptors in the skin. Rap- idly acting reflex arcs modulated by central inputs respond to multiple sensory inputs as well as emotional stimuli through three neuronal classes: sympathetic, whose principal neurotransmitters are epinephrine and norepinephrine; parasympathetic, whose principal neurotransmitter is acetylcholine; and nonadrenergic/noncholinergic, which include two subgroups—nitrergic, whose principal neurotransmitter is NO, and peptidergic, whose principal neurotransmitters are substance P, vaso- active intestinal peptide, calcitonin gene-related peptide, as well as a non-peptide, adenosine triphosphate (ATP). Each of these neurotransmitters acts through specific receptors on the vascular smooth-muscle cell to modulate intracellular Ca2+ and consequently, contractile tone. Norepinephrine activates α adrenergic receptors, and epinephrine activates both α and β receptors; in most blood vessels, norepinephrine activates postjunctional α1 receptors in large arteries and α2 receptors in small arteries and arterioles, leading to vasoconstriction. Most blood vessels express β2-adrenergic recep- tors on their vascular smooth-muscle cells and respond to β agonists by cyclic AMP–dependent relaxation. Acetylcholine released from parasympathetic neurons binds to muscarinic receptors on vascular smooth-muscle cells causing vasorelaxation. Nitrergic neurons release NO, which relaxes vascular smooth-muscle cell via the cyclic GMP– FIGURE 232-3 Assessment of endothelial function in vivo using blood pressure cuff occlusion and release. Upon deflation of the cuff, an ultrasound probe dependent and –independent mechanisms outlined, and other pepti- monitors changes in diameter (A) and blood flow (B) of the brachial artery (C). dergic inputs that regulate vascular tone. For the detailed molecular (Reproduced with permission of J. Vita, MD.) physiology of the autonomic nervous system, see Chap. 432. The release of endothelial effectors of vascular smooth-muscle cell systole promotes tissue perfusion during diastole. Arterial stiffness tone (Figs. 232-2 and 232-3) integrates mechanical (shear stress, cyclic associated with aging or disease, evident in a widening pulse pressure, strain, etc.) and biochemical stimuli (purinergic agonists, muscarinic increases left ventricular afterload and portends a poor outcome. agonists, peptidergic agonists). In addition to these local paracrine Like endothelial cells, vascular smooth-muscle cells do not merely modulators, a complex system of circulating modulators ranging from respond to vasomotor or inflammatory stimuli elaborated by other cell norepinephrine to the natriuretic peptides also modulate vascular types, but can themselves serve as a source of such stimuli. For exam- smooth-muscle cell tone. ple, when exposed to proinflammatory stimuli, smooth-muscle cells elaborate cytokines and other mediators which drive thrombosis and VASCULAR REGENERATION fibrinolysis as well as proliferation. Growth of new blood vessels can occur in response to conditions such Vascular Smooth-Muscle Cell Contraction Vascular as chronic hypoxemia and tissue ischemia. Growth factors, including smooth-muscle cells contract as cytoplasmic calcium concentration vascular endothelial growth factor (VEGF) and forms of fibroblast Harrisons_20e_Part6_p1649-p1942.indd 1654 6/1/18 12:48 PM Beta- 1655 NE, ET-1, Ang II NO ANP Agonist CHAPTER 232 Basic Biology of the Cardiovascular System VDCC K+ Ch Na-K ATPase PIP2 PLC G pGC G AC sGC GTP ATP SR RhoA DAG cGMP cAMP PKG PKA IP3R Plb RyrR ATPase IP3 PKC Calcium Rho Kinase MLCK Caldesmon Calponin MLCP FIGURE 232-4 Regulation of vascular smooth-muscle cell calcium concentration and actomyosin ATPase-dependent contraction. AC, adenylyl cyclase; Ang II, angiotensin II; ANP, atrial natriuretic peptide; DAG, diacylglycerol; ET-1, endothelin-1; G, G protein; IP3, inositol 1,4,5-trisphosphate; MLCK, myosin light chain kinase; MLCP, myosin light chain phosphatase; NE, norepinephrine; NO, nitric oxide; pGC, particular guanylyl cyclase; PIP2, phosphatidylinositol 4,5-bisphosphate; PKA, protein kinase A; PKC, protein kinase C; PKG, protein kinase G; PLC, phospholipase C; sGC, soluble guanylyl cyclase; SR, sarcoplasmic reticulum; VDCC, voltage-dependent calcium channel. (Modified from B Berk, in Vascular Medicine, 3rd ed. Philadelphia, Saunders, Elsevier, 2006, p. 23; with permission.) growth factor (FGF), activate a signaling cascade that stimulates and thin myofilaments. Thicker filaments, composed principally of the endothelial proliferation and tube formation, defined as angiogenesis. protein myosin, traverse the A band; they are about 10 nm (100 Å) in Guidance molecules, including members of the semaphorin family of diameter, with tapered ends. Thinner filaments, composed primarily of secreted peptides, direct blood vessel patterning by attracting or repel- actin, course from the Z lines through the I band into the A band; they ling nascent endothelial tubes. The development of collateral vascular are ~5 nm (50 Å) in diameter and 1.0 μm in length. Thus, thick and thin networks in the ischemic myocardium, an example of angiogenesis, can filaments overlap only within the (dark) A band, whereas the (light) I result from selective activation of local or circulating endothelial pro- band contains only thin filaments. On electron-microscopic examina- genitor cells. True arteriogenesis, or the development of a new blood tion, bridges extend between the thick and thin filaments within the vessel that includes all three cell layers, normally does not occur in A band; these are myosin heads (see below) bound to actin filaments. adult mammals, but recent scientific advances might help obviate such limitations (Chaps. 92 and 473). THE CONTRACTILE PROCESS The sliding filament model for muscle contraction rests on the central CELLULAR BASIS OF CARDIAC observation that both the thick and the thin filaments are constant in CONTRACTION length during both contraction and relaxation. With activation, the actin filaments are propelled farther into the A band. In the process, the A CARDIAC ULTRASTRUCTURE band remains constant in length, whereas the I band shortens and the Most of the ventricular mass is composed of cardiomyocytes, normally Z lines move toward one another. 60–140 μm in length and 17–25 μm in diameter (Fig. 232-5A). Each The myosin molecule is a complex, asymmetric protein with a molec- cell contains multiple myofibrils that run the length of the cell and are ular mass of about 500,000 Da; it has a rod-like portion that is about composed of series of repeating sarcomeres. The cytoplasm between 150 nm (1500 Å) in length with a globular portion (head) at its end. The the myofibrils contains other cell constituents, including a single cen- globular portions of myosin form the bridges to actin and are the site trally located nucleus, mitochondria, and the intracellular membrane of ATPase activity. In thick myofilaments, composed of ~300 longitu- system, the SR. dinally stacked myosin molecules, the rod-like segments of myosin The sarcomere, the structural and functional unit of contraction, lies assume an orderly, polarized manner, with outwardly projecting between adjacent Z lines, which are dark repeating bands apparent on globular heads interacting with actin to generate force and shorten transmission electron microscopy. The distance between Z lines varies (Fig. 232-5B). with the degree of contraction or stretch of the muscle and ranges Actin has a molecular mass of about 47,000 Da. Thin filaments con- between 1.6 and 2.2 μm. At the center of the sarcomere is a dark band sist of a double helix of two chains of actin molecules wound about of constant length (1.5 μm), the A band, which is flanked by two lighter each other on a larger molecule, tropomyosin. A group of regulatory bands, the I bands, which are of variable length. The sarcomere of heart proteins—troponins C, I, and T—localize at regular intervals on this fil- muscle, like that of skeletal muscle, consists of interdigitating thick ament (Fig. 232-6). In contrast to myosin, actin lacks intrinsic enzymatic Harrisons_20e_Part6_p1649-p1942.indd 1655 6/1/18 12:48 PM 1656 Myofiber PART 6 A Myocyte 10 µm Ca2+ Disorders of the Cardiovascular System enters Na+ Ca2+ Exchange T tubule Pump Ca2+ Myofibril “trigger” Ca2+ te leaves Myocy Free Ca2+ SR Myofibril Mitochondrion Contract Relax B Systole Myofibril Z C Diastole Actin Head Titin Myosin M 43 nm Z D FIGURE 232-5 A shows the branching myocytes making up the cardiac myofibers. B illustrates the critical role played by the changing [Ca2+] in the myocardial cytosol. Ca2+ ions are schematically shown as entering through the calcium channel that opens in response to the wave of depolarization that travels along the sarcolemma. These Ca2+ ions “trigger” the release of more calcium from the sarcoplasmic reticulum (SR) and thereby initiate a contraction-relaxation cycle. Eventually the small quantity of Ca2+ that has entered the cell leaves predominantly through an Na+/Ca2+ exchanger, with a lesser role for the sarcolemmal Ca2+ pump. The varying actin- myosin overlap is shown for (B) systole, when [Ca2+] is maximal, and (C) diastole, when [Ca2+] is minimal. D. The myosin heads, attached to the thick filaments, interact with the thin actin filaments. (From LH Opie: Heart Physiology: From Cell to Circulation, 4th ed. Philadelphia, Lippincott, Williams & Wilkins, 2004. Reprinted with permission. Copyright LH Opie, 2004.) activity, but combines reversibly with myosin in the presence of ATP (Fig. 232-6). Repetitive interaction between myosin heads and actin and Ca2+. Calcium activates the myosin ATPase, which breaks down filaments is termed cross-bridge cycling, and results in sliding of the ATP to supply the energy for contraction (Fig. 232-6). The activity of actin along the myosin filaments, with muscle shortening and/or the myosin ATPase determines the rate of actomyosin cross-bridge forma- development of tension. The splitting of ATP then dissociates the myo- tion and breakdown, and ultimately determines contraction velocity. sin cross-bridge from actin. In the presence of ATP (Fig. 232-6), actin In relaxed muscle, tropomyosin inhibits this interaction. Titin (Fig. and myosin filaments bind and dissociate cyclically if sufficient Ca2+ is 232-5D) an enormous, flexible, myofibrillar protein, connects myosin present; these linkages cease when [Ca2+] falls below a critical level, and to the Z line; its stretching contributes to the elasticity of the heart. the troponin-tropomyosin complex once more inhibits actin-myosin Dystrophin, a long cytoskeletal protein that binds to the dystroglycan interactions (Fig. 232-7). complex at adherens junctions on the cell membrane, tethers the sarco- Intracytoplasmic [Ca2+] is a principal determinant of the inotropic mere to the cell membrane at regions tightly coupled to adjacent con- state of the heart. Most agents that stimulate myocardial contractility tracting myocytes. Mutations in multiple sarcomeric and cytoskeletal (positive inotropic stimuli), including digitalis glycosides and β-ad- proteins cause different forms of inherited disease involving the heart renergic agonists, increase cytoplasmic [Ca2+], triggering cross-bridge and skeletal muscle. cycling. Increased adrenergic neuronal activity stimulates myocardial During activation of the cardiac myocyte, Ca2+ binds the heterotri- contractility through norepinephrine release, activation of β adrenergic mer troponin C, resulting in conformational changes in the regulatory receptors and, via Gs-stimulated guanine nucleotide-binding proteins, protein tropomyosin and exposing actin cross-bridge interaction sites activation of the adenylyl cyclase, which leads to the formation of Harrisons_20e_Part6_p1649-p1942.indd 1656 6/1/18 12:48 PM 1657 ADP ATP Pi 1. ATP hydrolysis CHAPTER 232 Basic Biology of the Cardiovascular System Relaxed Relaxed, energized 4. Dissociation of Actin Actin 2. Formation of actin and myosin active complex ATP Pi ADP ADP 3. Product dissociation Rigor complex Active complex FIGURE 232-6 Four steps in cardiac muscle contraction and relaxation. In relaxed muscle (upper left), ATP bound to the myosin cross-bridge dissociates the thick and thin filaments. Step 1: Hydrolysis of myosin-bound ATP by the ATPase site on the myosin head transfers the chemical energy of the nucleotide to the activated cross- bridge (upper right). When cytosolic Ca2+ concentration is low, as in relaxed muscle, the reaction cannot proceed because tropomyosin and the troponin complex on the thin filament do not allow the active sites on actin to interact with the cross-bridges. Therefore, even though the cross-bridges are energized, they cannot interact with actin. Step 2: When Ca2+ binding to troponin C has exposed active sites on the thin filament, actin interacts with the myosin cross-bridges to form an active complex (lower right) in which the energy derived from ATP is retained in the actin-bound cross-bridge, whose orientation has not yet shifted. Step 3: The muscle contracts when ADP dissociates from the cross-bridge. This step leads to the formation of the low-energy rigor complex (lower left) in which the chemical energy derived from ATP hydrolysis has been expended to perform mechanical work (the “rowing” motion of the cross-bridge). Step 4: The muscle returns to its resting state, and the cycle ends when a new molecule of ATP binds to the rigor complex and dissociates the cross-bridge from the thin filament. This cycle continues until calcium is dissociated from troponin C in the thin filament, which causes the contractile proteins to return to the resting state with the cross-bridge in the energized state. ADP, adenosine diphosphate; ATP, adenosine triphosphate; ATPase, adenosine triphosphatase. (From AM Katz: Heart failure: Cardiac function and dysfunction, in Atlas of Heart Diseases, 3rd ed, WS Colucci [ed]. Philadelphia, Current Medicine, 2002. Reprinted with permission.) the intracellular second messenger cyclic AMP from ATP (Fig. 232-7). The Ca2+ released from the SR diffuses to interact with myofibrillar Cyclic AMP in turn activates protein kinase A (PKA), which phospho- troponin C (Fig. 232-7), repressing this protein’s inhibition of contrac- rylates sarcolemmal Ca2+ channels, thereby enhancing the influx of Ca2+ tion, and so activating myofilaments to shorten. During repolarization, into the myocyte. the activity of the SR Ca2+ ATPase (SERCA2A) leads to Ca2+ uptake The SR (Fig. 232-8), a complex network of anastomosing intracellu- against a concentration gradient into the SR where complexes with lar channels, invests the myofibrils. The transverse tubules, or T sys- another specialized protein, calsequestrin. The uptake of Ca2+ is ATP tem, closely related to the SR, both structurally and functionally, arise (energy)-dependent and lowers cytoplasmic [Ca2+] to a level where from invaginations of the sarcolemma that extend into the myocardial actomyosin interaction is inhibited and myocardial relaxation occurs. fiber along the Z lines, i.e., the ends of the sarcomeres. There is also a sarcolemmal exchange of Ca2+ for Na+ (Fig. 232-8), reduc- ing the cytoplasmic [Ca2+]. Additional control of calcium compartmen- CARDIAC ACTIVATION talizaion results from cyclic AMP–dependent PKA phosphorylation of In the inactive state, the cardiac cell is electrically polarized; i.e., the inte- the SR protein phospholamban, permitting SERCA2A activation, increasing rior has a negative charge relative to the outside of the cell, with a trans- SR Ca2+ uptake, and so accelerating the relaxation rates, loading the SR membrane potential of –80 to –100 mV (Chap. 238). The sarcolemma, with Ca2+ for subsequent release, and stimulating contraction. which in the resting state is largely impermeable to Na+, and a Na+- and Thus, the combination of the cell membrane, transverse tubules, and K+- pump energized by ATP extrudes Na+ from the cell, and maintains SR, with their ability to transmit the action potential and release and then the resting potential. In the resting state, intracellular [K+] is relatively reaccumulate Ca2+, controls the cyclic contraction and relaxation of heart high and [Na+] is far lower; conversely, extracellular [Na+] is high and muscle. Genetic or pharmacologic alterations of any component, whatever [K+] is low. At the same time, in the resting state, extracellular [Ca2+] its etiology, can disturb any of the functions of this finely tuned system. greatly exceeds free intracellular [Ca2+]. The action potential has four phases (see Fig. 238-1B). During CONTROL OF CARDIAC PERFORMANCE the action potential plateau (phase 2), there is a slow inward cur- AND OUTPUT rent through sarcolemmal L-type Ca2+ channels (Fig. 232-8). Depo- The extent of shortening of heart muscle and, therefore, ventricular larizing current spreads across the cell membrane, penetrating stroke volume in the intact heart, depends on three major influences: deeply into the cell via the T tubular system. The absolute quantity (1) the length of the muscle at the onset of contraction, i.e., the preload; of Ca2+ traversing sarcolemma and T tubules is modest and insuf- (2) the tension that the muscle must develop during contraction, i.e., ficient to fully activate contraction. However, this Ca2+ current, the afterload; and (3) muscle contractility, i.e., the extent and velocity through Ca2+-induced Ca2+ release, triggers substantial Ca2+ release of shortening at any given preload and afterload. Table 232-2 lists the from the SR, inducing contraction. major determinants of preload, afterload, and contractility. Ca2+ is released from the SR through a Ca2+ release channel, a cardiac isoform of the ryanodine receptor (RyR2). Several regulatory proteins, THE ROLE OF MUSCLE LENGTH (PRELOAD) including calstabin 2, inhibit RyR2 and thus SR Ca2+ release. Inherited Preload determines sarcomere length at the onset of contraction. Con- disorders or exogenous factors affecting the efficiency or stability of tractile force is optimal at specific sarcomere lengths (~2.2 μm) where SR Ca2+ handling can impair contraction, leading to heart failure, or to myofilament Ca2+ sensitivity is maximal, and where myofilament inter- ventricular arrhythmias. actions and activation of contraction are most efficient. The relationship Harrisons_20e_Part6_p1649-p1942.indd 1657 6/1/18 12:48 PM 1658 Ca2+ β - Adrener PART 6 β γ αs Adenyl P cyclase SL Ca2+ + Disorders of the Cardiovascular System β GTP Receptor + cAMP SR Via protein kinase A + P Metabolic lycolysis Ca2+ lysis e cycle ADP + Pi + + ATP Troponin C cAMP Myosin + 2 via Tnl ATPase ADP + Pi + cAMP 1 via PL Increased 1. rate of contraction + β 2. peak force 3 3. rate of relaxation Control Force Time Pattern of contraction FIGURE 232-7 Signal systems involved in positive inotropic and lusitropic (enhanced relaxation) effects of a-adrenergic stimulation. When the β-adrenergic agonist interacts with the β receptor, a series of G protein–mediated changes leads to activation of adenylyl cyclase and the formation of cyclic adenosine monophosphate (cAMP). The latter acts via protein kinase A to stimulate metabolism (left) and phosphorylate the Ca2+ channel protein (right). The result is an enhanced opening probability of the Ca2+ channel, thereby increasing the inward movement of Ca2+ ions through the sarcolemma (SL) of the T tubule. These Ca2+ ions release more calcium from the sarcoplasmic reticulum (SR) to increase cytosolic Ca2+ and activate troponin C. Ca2+ ions also increase the rate of breakdown of adenosine triphosphate (ATP) to adenosine diphosphate (ADP) and inorganic phosphate (Pi). Enhanced myosin ATPase activity explains the increased rate of contraction, with increased activation of troponin C explaining increased peak force development. An increased rate of relaxation results from the ability of cAMP to activate as well the protein phospholamban, situated on the membrane of the SR, that controls the rate of uptake of calcium into the SR. The latter effect explains enhanced relaxation (lusitropic effect). P, phosphorylation; PL, phospholamban; TnI, troponin I. (Modified from LH Opie: Heart Physiology: From Cell to Circulation, 4th ed. Philadelphia, Lippincott, Williams & Wilkins, 2004. Reprinted with permission. Copyright LH Opie, 2004.) between initial muscle fiber length and the developed force is the VENTRICULAR AFTERLOAD basis of Starling’s law of the heart, which states that within limits, In the intact heart, as ex vivo, the extent and velocity of shortening the ventricular contraction force depends on the end-diastolic length of ventricular muscle fibers at any level of preload and of myocardial of the cardiac muscle; which in vivo relates closely to the ventricular contractility relate inversely to the afterload, i.e., the instantaneous load end-diastolic volume. opposing shortening. In the intact heart, the afterload may be defined as the tension developed in the ventricular wall during ejection. Afterload CARDIAC PERFORMANCE is determined by the aortic pressure as well as by the volume and thick- Ventricular end-diastolic or “filling” pressure can serve as a surrogate ness of the ventricular cavity. Laplace’s law specifies that the tension for end-diastolic volume. In isolated heart and heart-lung preparations, of the myocardial fiber is the product of the intra-cavitary ventricular stroke volume varies directly with the end-diastolic fiber length (pre- pressure and ventricular radius divided by wall thickness. Therefore, load) and inversely with the arterial resistance (afterload), and as the at any given aortic pressure, the afterload on a dilated left ventricle heart fails—i.e., as its contractility declines—it delivers a progressively exceeds that on a normal-sized ventricle. Conversely, at the same smaller stroke volume from a normal or even elevated end-diastolic aortic pressure and ventricular diastolic volume, the afterload on a volume. The relation between ventricular end-diastolic pressure and hypertrophied ventricle is lower than that on a normal chamber. Aor- the stroke work of the ventricle (the ventricular function curve) tic pressure in turn depends on the peripheral vascular resistance, the provides a working definition of cardiac contractility in the intact biomechanics of the arterial tree, and the volume of blood it contains organism. An increase in contractility is accompanied by a shift of the at the onset of ejection. ventricular function curve upward and to the left (greater stroke work Ventricular afterload finely regulates cardiovascular performance at any level of ventricular end-diastolic pressure, or lower end-diastolic (Fig. 232-10). As noted, elevations in both preload and contractility volume at any level of stroke work), whereas a shift downward and to increase myocardial fiber shortening, whereas increases in afterload the right characterizes depression of contractility (Fig. 232-9). reduce it. The extent of myocardial fiber shortening and left ventricular Harrisons_20e_Part6_p1649-p1942.indd 1658 6/1/18 12:48 PM atrial contribution to ventricular contraction can 1659 Na+ Na+/Ca2+ Plasma membrane be tolerated without a reduction in resting cardiac pump exchanger Ca2+ pump output. Under these circumstances, other factors, B1 B2 such as adrenergic neuronal impulses to the heart, Extracellular Plasma heart rate, and venous tone, will serve as compen- CHAPTER 232 Basic Biology of the Cardiovascular System T tubule membrane satory mechanisms and sustain cardiac output in a normal individual. Ultimately, understanding the Plasma Intracellular complex interactions between these different vari- (cytosol) ables requires rigorous models to predict relevant membrane Cisterna Ca2+ outcomes, and led to the early application of sys- channel tems engineering principles in medicine. Sarcoplasmic reticulum Ca2+- A release channel EXERCISE ('foot' protein) The integrated response to exercise illustrates the Sarcotubular network interactions among the three determinants of stroke A1 G volume: preload, afterload, and contractility (Fig. Calsequestrin 232-9). Hyperventilation, the pumping action of Sarcoplasmic reticulum the exercising muscles, and venoconstriction dur- Ca2+ pump Mitochondria ing exercise all augment venous return and hence C D ventricular filling and preload (Table 232-2). Simul- H taneously, the increase in the adrenergic neuronal stimulation of the myocardium, the increased con- centration of circulating catecholamines, and the tachycardia that occur during exercise combine to augment the myocardial contractility (Fig. 232-9, E F curves 1 and 2), together elevating stroke volume and stroke work, without a change in or even a reduction of end-diastolic pressure and volume (Fig. 232-9, points A and B). Vasodilation occurs in the exercising muscles, thus limiting the increase in Z-line Troponin C Thin Thick Contractile afterload that otherwise would occur as cardiac out- filament filament proteins put rises to levels as high as five times greater than basal levels during maximal exercise. This vasodila- FIGURE 232-8 The Ca2+ fluxes and key structures involved in cardiac excitation-contraction coupling. tion ultimately allows the achievement of elevated The arrows denote the direction of Ca2+ fluxes. The thickness of each arrow indicates the magnitude cardiac outputs during exercise at arterial pressures of the calcium flux. Two Ca2+ cycles regulate excitation-contraction coupling and relaxation. The larger cycle is entirely intracellular and involves Ca2+ fluxes into and out of the sarcoplasmic reticulum, as well only moderately higher than the resting state. as Ca2+ binding to and release from troponin C. The smaller extracellular Ca2+ cycle occurs when this cation moves into and out of the cell. The action potential opens plasma membrane Ca2+ channels to ASSESSMENT OF CARDIAC allow passive entry of Ca2+ into the cell from the extracellular fluid (arrow A). Only a small portion of FUNCTION the Ca2+ that enters the cell directly activates the contractile proteins (arrow A1). The extracellular cycle Several techniques can define impaired cardiac is completed when Ca2+ is actively transported back out to the extracellular fluid by way of two plasma membrane fluxes mediated by the sodium-calcium exchanger (arrow B1) and the plasma membrane function in clinical practice. Cardiac output and calcium pump (arrow B2). In the intracellular Ca2+ cycle, passive Ca2+ release occurs through channels in stroke volume may decline in the presence of heart the cisternae (arrow C) and initiates contraction; active Ca2+ uptake by the Ca2+ pump of the sarcotubular failure, but these variables are often within normal network (arrow D) relaxes the heart. Diffusion of Ca2+ within the sarcoplasmic reticulum (arrow G) limits, especially at rest. A more sensitive index of returns this activator cation to the cisternae, where it is stored in a complex with calsequestrin and cardiac function is the ejection fraction, i.e., the ratio other calcium-binding proteins. Ca2+ released from the sarcoplasmic reticulum initiates systole when of stroke volume to end-diastolic volume (normal it binds to troponin C (arrow E). Lowering of cytosolic [Ca2+] by the sarcoplasmic reticulum (SR) causes this ion to dissociate from troponin (arrow F) and relaxes the heart. Ca2+ also may move between value = 67 ± 8%), which is frequently depressed in mitochondria and cytoplasm (H). (Adapted from AM Katz: Physiology of the Heart, 4th ed. Philadelphia, systolic heart failure even when stroke volume is Lippincott, Williams & Wilkins, 2005, with permission.) normal. Alternatively, abnormally elevated ventric- ular end-diastolic volume (normal value = 75 ± 20 mL/m2) or end-systolic volume (normal value = 25 ± size determine stroke volume. An increase in arterial pressure induced 7 mL/m2) signifies left ventricular systolic impairment. by vasoconstriction, for example, augments afterload, which opposes Noninvasive techniques, particularly echocardiography, radionu- myocardial fiber shortening, reducing stroke volume. clide scintigraphy and cardiac magnetic resonance imaging (MRI) When myocardial contractility is impaired and the ventricle dilates, (Chap. 236) have great value in the clinical assessment of myocardial afterload rises (Laplace’s law) and limits cardiac output. Increased function. They provide measurements of end-diastolic and end- afterload also may result from neural and humoral stimuli that occur systolic volumes, ejection fraction, and systolic shortening rate, and in response to a fall in cardiac output. This increased afterload may they allow assessment of ventricular filling (see below) as well as reduce cardiac output further, thereby increasing ventricular volume reg