PVN 120 Notes 2024 Diagnostic Imaging (1) PDF
Document Details
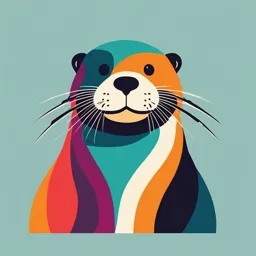
Uploaded by AmenableSnowflakeObsidian
Faculty of Veterinary Science
2024
Sr Cornelia Hanekom
Tags
Summary
These notes cover the introduction to basic diagnostic imaging, including different modalities like ultrasonography, computed tomography (CT), magnetic resonance imaging (MRI), scintigraphy, and endoscopy. The document also discusses principles of radiography and radiation safety. The target audience is Bachelor of Veterinary Nursing students.
Full Transcript
FACULTY OF VETERINARY SCIENCE Department of Companion Animal Clinical Studies BACHELOR OF VETERINARY NURSING I VETERINARY NURSING PRACTICE 120 (PVN 120) 2024 INTRODUCTION TO BASIC DIAGNOSTIC IMAGING Compiled by: Sr Cornelia Hanekom Copyright reserved 202...
FACULTY OF VETERINARY SCIENCE Department of Companion Animal Clinical Studies BACHELOR OF VETERINARY NURSING I VETERINARY NURSING PRACTICE 120 (PVN 120) 2024 INTRODUCTION TO BASIC DIAGNOSTIC IMAGING Compiled by: Sr Cornelia Hanekom Copyright reserved 2024 Table of contents 1. DIAGNOSTIC IMAGING MODALITIES................................................................................. 3 INTRODUCTION TO DIAGNOSTIC IMAGING........................................................................ 3 ULTRASONOGRAPHY............................................................................................................ 3 COMPUTED TOMOGRAPHY (CT)........................................................................................ 10 MAGNETIC RESONANCE IMAGING (MRI)........................................................................... 12 SCINTIGRAPHY (NUCLEAR MEDICINE).............................................................................. 14 ENDOSCOPY......................................................................................................................... 16 2. PRINCIPLES OF RADIOGRAPHY..................................................................................... 18 INTRODUCTION.................................................................................................................... 18 RADIATION SAFETY............................................................................................................. 19 RADIOGRAPHIC APPARATUS AND TECHNIQUE............................................................... 25 X-RAY EQUIPMENT............................................................................................................... 27 THE BEHAVIOUR OF THE PRIMARY BEAM AND IMAGE RECORDING............................ 46 INTERACTION BETWEEN X-RAYS AND MATTER.............................................................. 47 RECORDING THE RADIOGRAPHIC IMAGE........................................................................ 58 DIGITAL IMAGE RECEPTORS AND IMAGE ACQUISITION:............................................... 65 TELERADIOLOGY.................................................................................................................. 73 EVALUATION OF THE TECHNICAL QUALITY OF RADIOGRAPHS.................................... 73 COMPOSING A TECHNIQUE CHART................................................................................... 76 GENERAL PRINCIPLES AND RADIOLOGICAL TERMINOLOGY........................................ 76 GENERAL POSITIONING AND PREPARATION FOR RADIOGRAPHY............................... 78 CONTRAST MEDIA AND CONTRAST MEDIUM STUDIES.................................................. 81 Veterinary Diagnostic Imaging 1. DIAGNOSTIC IMAGING MODALITIES INTRODUCTION TO DIAGNOSTIC IMAGING In recent years different diagnostic imaging modalities, including advanced imaging, are more readily available to most clinicians. The use of computed or direct digital radiography is common, as is the use of computed tomography (CT) and Magnetic Resonance Imaging (MRI). Scintigraphy or Nuclear Medicine is popular to detect early physiological changes in order to provide a guide for further diagnostics, prognosis and treatment. Ultrasonography, computed tomography (CT) and magnetic resonance imaging (MRI) images are all examples of tomograms. Tomography is the cross-sectional slice imaging of an object under investigation in different anatomical planes. Endoscopy examinations are used to examine the hollow organs of the GIT as well as closed cavities such as joints and structures of the pelvic, abdominal and thoracic cavities. ULTRASONOGRAPHY Ultrasound is a form of energy consisting of mechanically produced waves with frequencies above the range of human hearing. Diagnostic ultrasound is an essential medical imaging modality and has the advantage of being very safe. Advantages of ultrasonography Ultrasound examinations are affordable, safe, non-invasive and painless and can very often be done without medication and sedation. Generally, ultrasonography has the advantage of producing real-time images and allows to view and evaluate internal organs with great accuracy. It can also be a useful tool in assisting with biopsies and guided cystocentesis. It is safe to diagnose pregnancies and assess foetal health. Doppler imaging can be used to examine blood flow in organs and structures of the heart. Disadvantages of ultrasonography The pressure applied on the transducer by the operator might cause discomfort in some cases. Ultrasonography is not the modality of choice to examine bone and gas/air containing structures. Image quality and accuracy as well as accurate reporting on findings are dependent on the operator’s skill and experience. The frequency of sound waves is measured in Hertz. The human ear can hear sound frequencies up to 20,000 Hz whereas diagnostic ultrasound utilizes frequencies of 1-15 MHz (1 MHz = 1,000,000 Hz). Sound is a mechanical wave of compressions and rarefactions within a medium and its components are amplitude (intensity of sound), velocity, frequency and wavelength. Diagram 1.1: Representation of a soundwave Velocity of sound in body tissues is fairly constant at ±1540 m/s or 13 microseconds per centimetre depth. Knowing the speed of sound in tissues allows one to calculate the depth of a particular reflecting surface within the body (remembering that the sound beam travels there and back again) and this, together with utilizing the intensity of the returning sound beam, is eventually converted by the on-board computer into the image. Sound interaction with matter Attenuation The transducer transmits the sound beam into the body where it undergoes attenuation (loss of beam strength). This is measured in dB/cm/MHz. Attenuation results from absorption and reflection. Absorption Absorption results in the formation of thermal energy which, theoretically, could be a biological hazard. Continuous exposure to high intensity sound might permanently damage cells. In considering the safety of diagnostic ultrasound: i. Diagnostic ultrasound uses low intensity ultrasound - only ± 1/1000 the strength of therapeutic ultrasound ii. Only transmitting 1/1000 of the time. The rest of the time the transducer is receiving signals. Transmission is only 3.6 seconds per hour iii. "Absence of evidence of safety is not evidence of absence of safety" - never image longer than you have to. The higher the frequency, the more sound beam absorption takes place, the less sound being available to evaluate deeper tissues. Doubling the frequency approximately doubles the absorption and approximately halves the beam intensity. Reflection (i) Scattered in all directions by very small irregular surfaces in an unpredictable manner. (ii) Reflected back to the transducer to make up the image. The amount of sound beam returned depends upon the acoustic impedance of adjacent tissues. Acoustic impedance = Tissue density X Tissue sound velocity All modern ultrasound systems are multi-frequency and thus the user can select a range of frequencies from a single probe/transducer. Most ultrasound transducers emit frequencies between 1-15MHz. The frequency plays an important role in image formation and resolution. In other word the ability to differentiate two adjacent individual structures as well as in the depth of penetration of the sound waves. Frequency of operation (1-15MHz): The absorption and reflection of the sound beam is directly affected by the frequency of the waves. Transducers are usually named after their frequency as well as the shape of the contact area of the transducer. As the frequency of the transducer increases, penetrability of the sound wave decreases but the ability to resolve small objects improves. Ultrasound transducers The sound beam is generated by a transducer which converts electrical energy into sound energy. This is done by Piezoelectrical crystals which are substances that on electrical or pressure stimulation alter their physical dimensions (Pierre & Jacques Curie - 1880). If the voltage is applied in a sudden burst the crystal vibrates and generates sound waves. The returning sound energy in turn causes a physical compression of the piezoelectric crystal which induces a voltage between the electrodes. This is amplified and transformed into a screen image. Piezoelectrical crystals are manufactured at a certain temperature and heating these crystals can result in loss of the Piezoelectrical effect. The crystal is also very fragile (Therefore be careful to never autoclave or drop a transducer!) Transducer components The case o Provides structural support for the internal filling and mechanical support for the foot print. The face o A protective window to match the active crystal. Piezoelectric crystal o The active element of the ultra sound transducer, where sound waves are generated and received to obtain a diagnostic image. Transducer types Electronic transducers Are commonly called arrays (group of elements) and consist of multiple adjacent piezoelectric crystals (64-120) which are fired rapidly in sequence to sweep across the patient. Modern electronic transducers can achieve variable focusing depths and have dynamic scanning frequencies. Linear array These result in rectangular images on the screen allowing for wide near field image visualization and as such are used commonly for gastrointestinal or soft tissue examinations (superficial structures or structures requiring increased detail). The crystals may be on the end of the transducer (good for abdominal scanning) or on the side of the transducer (good for rectal scanning of ovaries and the uterus). Convex array (curvilinear array, micro-convex): Produce a relatively wide near field sector image which is curved. Crystals are arranged in a convex fashion resulting in better far field resolution. Phased array: These produce a narrow near field sector image (looks like a slice of pie) and are formed by firing the crystals at slightly different times. Beam direction is changed by slight differences in phasing (time differences) Image 1.1: From left to right: curvilinear, micro-convex, linear, and phased-array transducers Image 1.2: Image produced by a curvilinear transducer Image 1.3: Image produced by a linear transducer Image 1.4: Image produced by a phased array transducer Operational Modes (Display formats) B mode (Brightness mode) Returning signals are displayed as grey dots on a screen with the echo intensity displayed as dot brightness on the screen. No echoes – black, anechoic; many echoes - grey to white, hypo- to hyperechoic. When B mode images are formed rapidly and presented in sequence organ movement may be visualized. This is known as real time B mode ultrasound and is the display format currently used. The number of times a complete image is composed per second is known as the frame rate. To form the sequential images enough time must be allowed for each ultrasound signal to enter and return from the patient. The deeper the image the slower the frame rate will be. Most machines operate at ± 30 frames per second. This is fast enough to exceed the ability of the human eye to perceive a flicker on the screen. In order to form these sequential images, it is necessary to sweep the sound beam over the tissues. Different mechanisms exist to do this, and this has resulted in a wide variety of transducers being on the market today. M mode (Motion mode) Is used to image moving structures with a strip recording made at right angles to the B mode display. Used especially in echocardiography to measure various cardiac structures. Doppler mode The Doppler mode is to measure / determine the blood flow speed and direction. Ultrasonography interpretation, principles and terminology Ultrasound instruments are calibrated to interpret and display the depth in centimetres automatically. A grey scale is also assigned to each dot corresponding to the amplitude or strength of the returning echo. Correct description of ultrasonograms assists in interpreting images and is essential for scientific communication. Intensity of the returning echoes (echogenicity): Descriptions of echogenicity Anechoic echo pattern - no returning echoes, e.g. Water, urine Hypoechoic - low intensity returning echoes, e.g. Renal medulla Hyperechoeic - high intensity returning echoes, e.g. Scar tissue or bone Isoechoeic - same number of returning echoes, e.g. Adjacent tissues Normo-echoic - echoes are of the expected intensity for that organ Complex echo pattern - multiple/mixed hyperechoic, hypoechoic and anechoic patterns, e.g. Certain neoplasms Patient preparation for US examination Starve patients for abdominal examination – presence of gas due to digestion can cause artefacts and poor imaging. It is not necessary to withhold water. Clip area of interest – air trapped between hair causes artefacts or poor image quality. Clipping for abdominal ultrasound examination stretches from flank fold to flank fold (or sometimes slightly beyond), caudally from the level of the acetabula and cranially to the xiphoid and laterally lined up with the flank fold to include 2-3 intercostal spaces. Generous amounts of coupling gel should be placed on the skin to ensure good contact between the transducer and the patient. Failure of uniform transducer contact will result in no echoes where there is no contact with the skin, resulting in no or bad image quality, despite generous amounts of coupling gel and a clipped patient. Sedation if needed – easier patient restraint and better images (result in relaxed abdominal muscles; only certain sedatives to be used for echocardiography) Clinical application The clinical applications of ultrasonography include the following: Examination of abdominal organs (evaluation of organ size, internal structure, blood flow and presence of masses/nodules) Other abdominal examinations such as pregnancy diagnosis, foetal assessment, presence of foreign bodies, etc. Internal structures of the eye Thyroid Larynx Thoracic structures and organs Heart (echocardiography) Blood vessels Muscles, soft tissue, tendons and ligaments Pregnancy diagnosis Foetal health assessment Maintenance and care of the ultrasound equipment Sterilization and disinfection Clean and disinfect the equipment as soon as possible after use. Clean transducers after use by wiping off the contact gel. Do not autoclave transducers. Keep the equipment clean and dry when it is not in use. To ensure the correct cleansing agent and disinfectant is used, contact the manufacturer and ask their advice. Equipment care Clean the transducer-face (on and around membrane) with gauze damped with a suitable disinfectant or wash it with water. Clean scanner body and cable with gauze damped with a suitable disinfectant. Avoid applying heat to the transducer, as it consists of electronic components and plastics. Do not emerge the connector in liquid it will be damaged. Don’t drop transducers – the crystal will break (transducers are very expensive to replace) Freeze image when changing transducers or machine is not in use. Switch machine off after completion of the examinations for the day. Keep machine and keypad free of dust, hair and ultrasound gel – remember to clean the track- ball! Protect transducers during equine ultrasonographic procedures e.g., if the abdomen is not clipped, use 70 % ethanol to wet hair, protect transducer with a plastic cover (e.g., latex examination glove / condom). Room preparation Ensure availability of coupling gel. Clean the table before and after completion of each examination. Wipe the transducer after each examination is completed. The room should have dimmed lighting. If imaging in a bright room the operator tends to increase the gain to brighten the image. This often results in missing subtle changes. Have a PACS system, transfer images to PACS. Record keeping of images Modern ultrasound machines store DICOM images which can be exported in DICOM or JPEG format to a PACS system and/or flash drive to be stored on another computer. The name of the veterinary facility, attending doctor, owner detail and identification of patient must appear on all images. COMPUTED TOMOGRAPHY (CT) Physics of computed tomography CT allows the cross-sectional slice imaging of a subject. X-rays are used with similar principles to conventional radiography. Small density differences between soft tissues are however detectable and no overlying structures hinder the visibility of smaller structures. CT machine and imaging The X-ray machine rotates around the patient. X-ray detectors are placed opposite the X-ray tube and also rotate, or detectors are stationery and are placed in a 360-degree ring. The X-rays are attenuated as they pass through the patient. The detectors detect the remnant radiation. Using this information, the computer reconstructs an image. The CT image is divided into pixels, each representing a certain volume (or voxel) of tissue. Each image represents a certain slice thickness of tissue varying from 1-3 mm to 10 mm, depending on region imaged and size of patient. Each pixel is given a certain grey scale depending on the attenuation by that voxel of tissue. Grey scale is expressed in terms of CT numbers or Hounsfield units. Water is 0 (grey), bone is +1000 or higher (white) and air is -1000 (black). Settings can be manipulated to emphasize either bony structures or improve soft tissue contrast. Similar to conventional radiography the degree of opacity/whiteness is directly proportional to the density and atomic number of the tissue. A scout image or topogram is initially made, which appears similar to a normal radiograph. This allows assessment of the patient positioning and allows planning of where the slices are to be made. To prevent distortion of the images, patients must be symmetrically positioned. The scout image can also be used as a reference when examining the images later to see where a certain slice is positioned. Contrast agents can be injected and the study repeated. This improves image contrast and detect areas of pathology not seen on pre-contrast images. Areas of high vascularity will enhance including most tumours and inflammatory areas. Clinical applications (not limited to the following) Head Cranium (fractures) Brain tissue (mass effect, hydrocephalus) Nasal passages and periorbital region – location and extent of disease processes (rhinitis/neoplasia and bone involvement) Spine Disc prolapses (can combine with myelography) and cauda equina evaluation, particularly those cases with bone changes on the radiographs. Fractures Neoplasia Thorax Detection of masses especially near midline or in mediastinum not seen on routine radiography, metastases, causes for pneumothorax or pleural effusions. S.Lupi Vascular ring anomalies Abdomen Adrenal or other masses Ectopic ureters Portosystemic shunts Joints Fragmented medial coronoid process Tarsal OCD, particularly if difficult to see on radiographs Patient preparation Patients for CT examinations need to be anaesthetized or under heavy sedation as this examination takes time. Patients need to be starved appropriately and the preparation, induction and maintenance of the anaesthetic should be executed and monitored carefully. Post procedural observation for proper recovery from the GA needs to be done. Proper patient position is maintained with positioning aids. A scout image is made prior to the CT examination to ensure proper patient positioning and to plan the slices for the imaging. If the patient is not properly positioned symmetry in the image will be distorted which hampers interpretation. Radiation safety principles during CT examinations All persons participating in the CT examination should adhere to normal radiation safety protocol (appropriate personal protection, behind barriers, safe distance from CT scanner). Ideally no person should be in the vicinity of the CT scanner whilst the machine is scanning. If possible, the patient should be on its own in the CT room during the relatively short time of the scan, but proper monitoring of the patient should be done throughout the entire procedure and the patient should not be left unattended / out of sight. Additional reading: https://www.vin.com/apputil/content/defaultadv1.aspx?pId=14365&catId=73687&id=7259422 MAGNETIC RESONANCE IMAGING (MRI) Advantage of MRI is the excellent soft tissue contrast compared to other imaging modalities as well as ability to build up cross-sectional images. Even tissues with minor differences in physical density can be distinguished. MRI is especially ideal for central nervous system imaging. Basic imaging procedure involves placing the patient in a magnetic field, a radiofrequency wave is applied which interacts with protons in the patient. The radiofrequency wave is turned off which results in a signal being emitted from patient which is received by an antenna and the image is reconstructed by computer. Image construction The large main magnet is a superconducting magnet. (A superconductor has zero resistance at very low temperatures, and thus once a current is started, it continues without further power input). Permanent magnets are also used. Field strength varies from 0,5 - 7 tesla (T). Superconductor magnets have excellent homogeneity but the entire system must be maintained at 40 Kelvin (-269 ° Celsius), using liquid helium and liquid nitrogen, which is expensive and must be replenished at times. The main magnetic field is modified by gradient coils within the bore of the magnet to allow accurate localization of all given signal to a specific slice of tissue and thus allow cross-sectional imaging and spatial resolution. Radiofrequency coils transmit and receive radiofrequency signals. The computer controls the RF signals and reconstructs the image. Protons in the patients are hydrogen nuclei. These protons have a positive charge and are constantly spinning, allowing them to act as tiny bar magnets. During scanning, when the patient is placed in the magnetic field, the protons all align themselves with the long axis of the main magnetic field. Radiofrequency waves are then pulsed into the patient, and the energy is absorbed by the protons. The protons then move out of their alignment with external magnetic field and start rotating around the original axis (precession) and then again return to their original alignment (relaxation). The precession induces an electrical signal in a receiver coil. Relaxation and signal vary with tissue types. The signals are converted by a computer to reconstruct the image. The image is divided into pixels each with a certain grey-scale value. The signal strength gives rise to the intensity of the grey-scale value that is assigned to that specific volume of tissue (voxel). Structures and abnormalities are described according to their intensity on each sequence, often in relation to other tissues e.g., T2W hyperintense to white matter. Tissues with high hydrogen content (fat and water) are well imaged whereas regions of bone and air appear as voids with no signal. Proper positioning of patient is important to ensure exact symmetry. Clinical applications of MRI (not limited to the following) Head MRI is ideal for CNS imaging and superior to CT for brain imaging. Brain lesions detected include congenital lesions (cerebellar hypoplasia, arachnoid cysts, and hydrocephalus), haemorrhage and infarcts, neoplasia (meningiomas, gliomas, choroid plexus tumours). The brain is assessed for intensity and symmetry of intra-cranial structures, the size and symmetry of lateral ventricles, degree and pattern of enhancement, signs of increased cranial pressure or mass effect etc. Other indications include ocular imaging. Spine Spinal column lesions can also be imaged. Assessment of discs (degeneration, protrusions and extrusions), intramedullary lesions, and lumbosacral disease (cauda equina compression). Joints Joint imaging allows improved soft tissue evaluation. Ligaments, tendons, and joint capsules can be distinguished and articular cartilage can be assessed. Joints most often imaged in small animals are the shoulder and stifle. In equine patients it is considered the gold standard for evaluation of structures within the hoof capsule. Abdomen Caudal abdominal imaging can be done although ultrasound will usually be the first-choice imaging modality. Cranial abdomen has too much respiratory motion (e.g., Liver next to diaphragm). This is mostly for research purposes in veterinary medicine at this stage. Thorax MRI is not used routinely for thoracic studies due to excessive movement and gas not being well imaged by MRI. Patient care and preparation During the procedure the patient is exposed to a large magnetic field. This implies that all ferrous / magnetic objects such as collars, chains, leads and other metallic objects should be removed from the patient. Careful consideration before imaging of patients with metallic implants and pacemakers is necessary as certain implants are magnetic and can move or cause severe artefacts. In these cases, an alternative and suitable diagnostic imaging modality should be used. Patients should be under general anaesthetic and all the necessary preparation pertaining to the GA should be done. Patients need to be starved appropriately and the preparation, induction and maintenance of the anaesthetic should be executed and monitored carefully. Post procedural observation for proper recovery from the GA needs to be done. The MRI scan takes quite some time to be completed. During this time the patient is exposed to high levels of noise. It is advised that the ears are plugged or covered for noise protection. All the equipment or furniture within the MRI room should be non-ferrous / non-magnetic to ensure patient and general safety. Potential hazards and precautions The use of these magnets may be dangerous and loose ferrous metallic objects in the imaging area can become potential missiles. People with pacemakers must be excluded from the imaging room. All anaesthetic equipment must be made of non-ferrous material so it can be used next to the magnet. Veterinary patients are often given long-acting intravenous anaesthesia. The magnetic field will cancel magnetic strips on bank and credit cards as well as university ID cards. The magnetic field will also render smart phones and watches non-functional. SCINTIGRAPHY (NUCLEAR MEDICINE) Terms commonly used in scintigraphy Radiopharmaceuticals are a group of drugs that contains radioactive isotopes that are bound to organic molecules that is used during the Scintigraphy examination. Radionuclide (radioisotope) is an unstable form of an atom containing excess nuclear energy that radioactively disintegrates (decays), resulting in the emission of nuclear (gamma) radiation. Radioactive decay / half-life is the process of spontaneous break down and loss of energy through radiation of an atomic nucleus, and the time it takes for 50 % of the radioactive atoms to decay, respectively. (A material or element containing an unstable nucleus is considered radioactive.) Data collection / image formation Scintigraphy is an imaging procedure that utilizes radiopharmaceuticals to diagnose abnormal function by detecting the distribution of gamma rays emitted by the radiopharmaceutical in the affected structure over a period of time. Scintigraphy can detect diseases that alter physiology before morphological changes become evident. Some atoms have nuclei that contain excess energy and are known as radionuclides. They exist in an abnormally excited state and spontaneously emit particles and energy (alpha, beta or gamma rays). This is known as radioactive decay. The gamma rays are produced at a constant rate of decay for each radionuclide. This radioactivity is measured in Becquerels (= 1 disintegration per second). Radionuclides may be used for radiation therapy or diagnostic purposes. The most commonly used radionuclide is Technetium-99m which is formed by the beta decay of Molybdenum. Technetium-99m is an ideal radionuclide as it has a short half-life (6 hours), emits easily detectable low energy gamma rays and readily binds to other compounds to form radiopharmaceuticals. A radiopharmaceutical is a chemical substance that contains a radionuclide within its structure and is suitable for the diagnosis or treatment of disease. It may be formulated in various physicochemical forms (commercially available as kits) to deliver the radionuclide to a particular organ. The emitted gamma rays can be externally detected, measured and an image created by means of a gamma (scintillation) camera. Image formation The gamma camera consists of a collimator, detector, positional circuitry, counter and recording device. The patient is positioned in front of the gamma-ray-detecting camera. In normal/healthy patients the gamma radiation that is emitted will display symmetrical or normal distribution. Cells, tissue or organs with physiological activity / early disease will have an increased uptake of the radiopharmaceutical and emitted gamma radiation will display asymmetrical or will be evenly distributed in the active area. The image is built up for a certain number of counts for a specific time and displayed on a monitor. Focal areas of increased uptake of radiopharmaceutical are known as “hot spots” whereas lack of radiopharmaceutical uptake is known as a “cold spot” or photopaenia. Radioisotopes used for bone and thyroid examination The radiopharmaceutical used for bone examinations is methylene diphosphonate attached to Technitium-99m. For examinations of the thyroid, an iodine compound is used. The radiopharmaceutical is administered intravenously it is carried by the organic molecule to the cells, tissue or organ investigated. Clinical applications (not limited to the following) Skeletal scintigraphy Bone imaging is one of the more commonly performed procedures in veterinary nuclear medicine. It has a high sensitivity for detecting early disease before radiographic changes become apparent, and can rapidly survey the entire skeleton for metastatic or polyostotic lesions. The evaluation can be divided into 3 phases: 1. The vascular phase is performed within the first 2 minutes and is a sensitive test for loss or increase of vascularity and acute inflammatory processes. 2. This is followed within 2-5 minutes by the soft tissue/extracellular fluid phase which is useful for detecting tendon/ligament injuries, synovitis and myositis. 3. Thirdly the bone phase, performed 2-3 hours later to detect increased bone metabolic activity as seen with non-displaced fractures, osteoarthrosis, periosteal reactions, osteomyelitis and tumours. The technetium-99m is bound to a diphosphonate (e.g., methylene diphosphonate). After intravenous injection, the diphosphonate binds to the exposed hydroxyapatite crystals of the bone matrix during the bone phase. The isotope intensity reflects the perfusion and osteoblastic activity of bone in this region, particularly that of reparative processes. Portosystemic shunt scintigraphy (PSS scintigraphy) Portosystemic shunts are a common congenital or acquired disorder seen in dogs (and to a lesser extent in cats). Multiple clinical-pathologic tests as well as imaging procedures are available but are not always specific. PSS scintigraphy provides a rapid and accurate non-invasive screening mechanism to detect the condition and its severity (shunt fraction) as well as to evaluate the efficacy of any surgical intervention (gold standard for post-surgical evaluation). A dynamic study of multiple image frames of the liver and heart regions is performed. Interpretation involves visual assessment of the images to see if the isotope reaches the heart before the liver (confirming a large shunt), or reaches both at about the same time (smaller shunt). The shunt fraction can be determined by generating time-activity curves for the liver and heart regions. Thyroid scintigraphy This is a common procedure in older cats suspected of having hyperthyroidism to determine the size and functional status of both glands, the location of ectopic functional tissue for surgical planning, metastasis, and evaluating response to therapy. In the dog it is used for evaluating thyroid tumours (size, functional status, and metastasis), evaluating suspect hypothyroidism and cervical masses of unknown tissue origin. Sodium 99mTc-pertechnetate is more commonly used than iodine isotopes because of the longer half-life and absorption time of the latter. Anti-thyroid drugs inhibit iodide uptake for 1 week, but not technetium, whereas both iodide and technetium uptake is inhibited by iodinated radiographic contrast media for 1-2 months. This should be considered when referring a patient for thyroid scintigraphy. ENDOSCOPY Endoscopy is the examination of the inside of the body by using a lighted, flexible instrument called an endoscope. In general, an endoscope is introduced into the body through a natural opening such as the mouth or anus. Although endoscopy can include examination of other organs, the most common endoscopic examination / procedures evaluate the oesophagus, stomach and portions of the intestine. (https://www.medicinenet.com/script/main/art.asp?articlekey=12538) Endoscope An endoscope is a medical device used to examine the inside of the body by entering either through a natural opening (mouth or anus) or through a small incision. The endoscope consists of: A tube (either flexible or rigid) containing bundles of optical fibre. The optical fibre is made from very thin glass and is surrounded by a durable cladding. A light source is located at the end of the tube outside the body. Visible light is guided from here along bundles of optical fibre to illuminate the organ or artery under examination. Lenses at the inner end of the tube. The lens system collects light to form the image. This light is sent through another bundle of optical fibre, back to a viewing lens / eye piece / video camera. An additional channel to allow entry of medical instruments or manipulators is present. Clinical applications / uses (not limited to the following): Hollow organs Gastrointestinal tract Oesophagus Stomach Duodenum Small intestine Large intestine / colon Rectum Respiratory tract Nose Lungs Urinary tract Other organs / structures Arteries Ears Normally closed body cavities (via small incision) Pelvic cavity Joints Organs of the thorax Other functions Biopsies – tissue collection from organ under examination Cut or destroy tissue – by means of laser technology Care and maintenance of the endoscope Proper cleaning and maintenance of the endoscope is important to ensure longevity of the equipment, diagnostic images of good quality and it will improve patient safety. Endoscopes that are not properly cleaned is a source of nosocomial infections. Bacterial infections such as Pseudomonas, Salmonella and kennel cough can easily be transmitted by dirty endoscopes. Cleaning and disinfecting the endoscope Basic eight step routine for cleaning and disinfecting of endoscopes (http://www.beckersasc.com/gastroenterology-and-endoscopy/8-steps-for-proper-endoscope- maintenance.html). 1. Pre-clean. Immediately after the procedure, wipe the endoscope with an approved enzymatic solution and flush out channels with a solution to lubricate and loosen debris. Wash the suction, air and water channels according to the manufacturer's instructions. 2. Test for leaks. Immerse the scope in water and test for leaks, following your manufacturer's instructions. 3. Soak and clean. Using the proper type and amount of enzymatic solution, fill the endoscope channels and soak for the prescribed time. While it is soaking, brush out the channels and clean the valve-ports, all valves, attachments and the exterior of the scope. After the soak, flush out the channels once again and clean behind the device's elevator. Repeat the process if necessary. 4. Rinse. Thoroughly rinse the exterior of the endoscope using clean water. Flush out all channels with the manufacturer-supplied adapters. Repeat this process and then purge channels with air to remove excess water. 5. Disinfect. Fill all channels with the proper disinfectant and then completely submerge the endoscope into the solution. Soak for the prescribed time. 6. Rinse. Completely submerge the endoscope in fresh water and again flush all the channels. Repeat the process if there are any traces of disinfectant on the endoscope. 7. Flush with alcohol and dry. Flush all endoscope channels with the prescribed amount of alcohol-water mixture, which is usually approximately 70-80 percent alcohol. The alcohol aids drying. Wipe the exterior with a cloth and dry all attachments. 8. Storage. Store the endoscope in a well-ventilated container, storing all valves and attachments separately. Always follow the manufacturer’s guidelines for proper care and maintenance of endoscopic equipment. Follow the link below to watch a short visual on endoscope care and maintenance. https://www.youtube.com/watch?reload=9&v=Pv0I3s7xzUs 2. PRINCIPLES OF RADIOGRAPHY INTRODUCTION Radiography is an important procedure in general veterinary practice which encompasses the art - and science of permanently recording radiographic images of patients for diagnostic purposes. Roentgen radiation is potentially dangerous and it is therefore compulsory for anyone regularly involved in radiography, even in a part-time capacity, to be registered as a radiation worker and to be monitored regularly for exposure to radiation. Proficiency in the use of radiographic apparatus and in the evaluation of the quality of radiographs, combined with a sound knowledge of contrast medium techniques and correct positioning of patients, ensures a more accurate and reliable diagnostic service, while an awareness of the dangers of exposure to radiation ensures your own personal safety as well as that of your assistants, clients, and patients. RADIATION SAFETY RADIATION DANGERS AND THEIR PREVENTION DANGERS OF EXPOSURE TO ROENTGEN RADIATION X-rays, both primary and scattered, are potentially dangerous to living beings exposed to them. Theoretically any dose of radiation, no matter how small, must be assumed to carry some potential risk for the recipient. This assumption must however be placed in proper perspective in order to avoid over-exaggeration of the danger involved. All living beings are constantly and unavoidably subjected to so-called background radiation coming from cosmic sources as well as from a variety of natural and manmade sources. The degree of exposure varies from place to place but on average the exposure per person is estimated to be 1 millisieverts (mSv) per year. The effect of this exposure on the general population of the earth is negligible. That does not mean to say that one should not be careful about exposing oneself to radiation. Exposure without justification simply cannot be condoned. When using radiographic equipment we are exposed to additional radiation over and above this background radiation and if we do not take the necessary, routine precautions to limit such exposure we will be exposed to a higher risk than the general population. It is for this reason that people using any equipment capable of producing ionizing radiation or who are potentially exposed to the radiation produced by such equipment on a regular basis should be registered as radiation workers and be monitored regularly to determine their exposure to such additional avoidable radiation. The quantity of additional radiation to which one is exposed may not exceed the prescribed dose equivalent limit (DEL). The DEL is the maximum quantity of radiation which, when it is received in a specified time, is construed not to carry a degree of risk which is unacceptable to the exposed individual i.e. can be considered as reasonably safe. Driving a motorcar carries the risk of getting involved in an accident and yet we all continue to drive around in them thereby accepting the risk involved! So, although a health risk exists when one is involved with radiography, it is regarded as relatively small provided every necessary care is taken to limit the exposure incurred to the absolute minimum. The fact that veterinary patients have to be restrained manually for radiographic examinations increases the risk. The higher the radiographic workload the higher the risk involved. We should therefore conscientiously apply all the safety measures at our disposal to protect ourselves, other employees, our patients, as well as the general public, and to avoid legal liability due to negligence. HEALTH RISKS ASSOCIATED WITH EXPOSURE TO RADIATION The biological effects of radiation are caused by the absorption of X-ray photons in the cells of the body. The severity of the effects will depend on the degree to which the delicate biochemical functions of the recipient cells are disturbed and may consist of deranged function, a growth abnormality, or even cellular death. The susceptibility of different tissues in the body to the harmful effects of radiation varies considerably. Tissues in which there is a high rate of cell division e.g., bone marrow, spleen, dermis, mucosae, embryos, and growing tissues generally are very vulnerable. Connective tissue, bone, and nervous tissues on the other hand are fairly resistant. The size of the area exposed to radiation also plays a role. Simultaneous exposure of the whole body or large parts of the body to radiation is much more dangerous than radiation confined to only a small part of the body. Smaller areas can withstand much higher doses of radiation than the body as a whole before harmful effects will result. The increased hazard associated with whole body irradiation is due to the fact that many vulnerable tissues are exposed simultaneously. The blood-forming tissues in particular are very prone to the effects of such widespread irradiation. The biological effects of exposure to radiation are cumulative. Numerous small exposures will eventually have the same effect as a single massive exposure. This applies to both whole body and localized radiation. The harmful effects of radiation exposure can be classified as: 1. Somatic effects There are short term changes that affect the recipient of radiation which affect rapidly reproducing cells. These include diarrhoea, erythema, acute dermatitis, hyperkeratosis, necrosis, hair loss, cataracts, temporary or permanent sterility. 2. Carcinogenic This is an increased risk of neoplasia, and the effect usually only manifests itself after a latent period of several years in the form of leukaemia or squamous cell carcinoma of the skin. 3. Genetic effects These effects arise from changes in the genes or chromosomes of the reproductive cells of the recipient and will therefore only become evident in the progeny of the recipient. These mutations are invariably disadvantageous. PROTECTION AGAINST RADIATION EXPOSURE It is the duty of the radiation worker in charge of radiographic examinations to be conversant with the health hazards relevant to radiography and the protective measures they are obliged to apply in order to safeguard their own health as well as that of assistants and patients, and clients called upon to assist in radiographic examinations. Radiography can be a safe procedure provided one is always aware of the possibility of exposure to either primary or scattered radiation and on condition that the following general rules are applied routinely in order to limit exposure to the minimum. General protection of public and workers (a) Persons not participating in the radiographic examination of a patient must leave the room. (b) Under no circumstances should pregnant women or children under 18 years be permitted to assist in the positioning of patients for radiographic examination or be allowed in the room during the examination. (c) Switch on warning lights at the entrance to the radiography room or lock the door so that nobody can enter. (d) Veterinarians, veterinary nurses and their assistants who participate in radiographic examination of patients must be registered as radiation workers with the Department of Health and a radiation monitoring service. Veterinary nursing students are registered as radiation workers at the beginning of their senior year. Allotted BIN's (Bureau Identity Numbers) are retained for life. (e) While on duty every registered radiation worker must wear his/her personal dosimeter under the protective apron. These dosimeters must not be worn when radiation workers are themselves subjected to radiographic procedures for diagnostic purposes or for radiotherapy. Protective clothing/structures (Barriers) All persons present should: (a) Wear protective aprons. This effectively reduces the surface area of the body of the radiation worker exposed to scattered radiation thus reducing the quantity of whole-body radiation received (b) Wear lead gloves. Gloves and aprons are expensive and should be cared for properly. They must be regularly inspected for damage. Gloves are very soft and easily damaged. They should never be used for handling vicious dogs, cats, birds of prey, parrots etc. in the false expectation that they will protect one's hands against bites, scratches, or clawing. Gloves can be radiographed using a high kV value to demonstrate cracks which may not be visible from the outside. Aprons should never be draped over sharp edges or folded repeatedly as this will cause cracking. When not worn they should preferably be suspended from heavy duty coat hangers. Damaged gloves and aprons should be discarded because they will not provide maximum protection. (c) Stand behind shielding devices like lead-covered or lead glass partitions during exposures. (d) When available protective glasses (to protect eye lenses) and a thyroid protector should be worn, especially by those people who regularly position animals or are exposed to high mAs procedures Specific protective procedures for radiation workers (a) Face the roentgen unit during the actual exposure (aprons often do not protect the back). (b) Preferably position themselves as far as possible from the patient during the exposure to decrease the intensity of the scattered radiation reaching them. The inverse square law also applies to scattered radiation. (Distance) (c) The hands of any person positioning a patient are particularly prone to radiation exposure. To avoid such exposure protective lead-plastic gloves must be worn and the hands, even when gloved, kept out of the primary beam if possible. When feasible, positioning aids that will dispense with the need for manual positioning should be used. Manual positioning of patients is less dangerous if collimation is sophisticated, and less acceptable and more hazardous if it is poorly controlled or limited as is often the case with smaller radiographic units using cones or fixed-aperture diaphragms. Cassettes should not be hand-held for horizontal exposures unless collimation is very sophisticated and the beam can be reduced to small area thus avoiding exposure of the gloved hand. (d) Never handhold a portable roentgen tube-head during exposures. Some primary radiation penetrates the tube-housing and will expose the hands. Additionally, the weight of the head is likely to result in motion blur. (e) A radiation worker who becomes pregnant must immediately inform her employer. She may continue to do radiography provided she: - stands behind a proper protective wall (barium plastered) during exposures; - does not participate in screening, radiotherapy, or any radiography utilizing mobile or portable radiographic units in hospital wards, operating theatres, on farms, etc., even if wearing a protective apron. Decrease exposure factors (Time – exposure time) (a) Reduce the quantity of scattered radiation produced to a minimum by avoiding high mAs values and collimating properly. (b) Use high kV low mAs techniques where possible. Decrease the number of retakes (Time – times exposed) (a) Plan the whole radiographic procedure carefully (views, grid, direction of exposure, exposure factors, positioning, etc.) so as to avoid unnecessary retakes. Every retake contributes to radiation exposure. (b) Utilize a technique chart. (c) When the patient is in pain or uncooperative, or if the exposure time is going to be very long, a sedative or general anaesthetic should be given. General (a) When making horizontal exposures (commonly done on the limbs of large animals) ensure that nobody is standing in the path of the divergent primary beam. It is better to do these examinations in a stable or partial enclosure which is roofed so that a wall is present behind the patient for absorbing the X-rays and sunlight cannot interfere with the visibility of the collimated area. (b) The walls of the radiography room or any partitions used in these rooms must comply with safety regulations so that they do not allow penetration by primary X-rays. (c) Avoid fluoroscopy as far as possible due to the dangers involved. This procedure is best left to competent examiners with sophisticated equipment. (d) Do not attempt to use a diagnostic roentgen unit for radiotherapy. This will damage the anode by overheating it and the procedure is also unsafe due to the vast quantity of radiation that is generated. Radiotherapy should be left to qualified persons who are properly equipped. (e) When a tube-head is accidentally damaged, e.g. kicked by a horse or dropped, it should be examined by a properly qualified and equipped technician for excessive leakage of primary radiation. PROTECTION OF THE PATIENT Health risks resulting from diagnostic radiography are relatively remote in our patients because they have short lifespans, which reduces the possibility of cumulative effects, and few of them are subjected to frequent and numerous radiographic examinations and/or screening. Radiotherapy could have serious consequences especially when a large area has to be treated e.g. extensive squamous cell carcinoma of the ventral abdominal skin in certain canine breeds. Some extra consideration should be given to the possibility of ill-effects on the progeny of breeding animals caused by gonadal irradiation, and on foetuses when pregnant animals are examined radio-graphically. The overriding principle involved here is to limit exposure to the absolute minimum or, if feasible, to eliminate it altogether. Exposure of the patient can be limited by: collimating the primary beam properly using fast image receptors (when possible) to decrease the mAs required. using high kV, low mAs techniques Exposure of the testes can easily be prevented by clamping a homemade lead cup around the scrotum or by covering them with protective gloves or a lead-plate. In the female exposure of the ovaries can be prevented by proper collimation, or covering the abdomen with protective aprons or lead plates when the beam cannot be reduced sufficiently. DOSIMETRY AND MONITORING OF RADIATION WORKERS Monitoring of registered radiation workers is currently done by the Panasonic TLD (thermoluminescent dosimeter) system. The dosimeter is worn under the protective apron to determine the quantity of whole-body radiation to which the wearer is exposed. It should be worn at all times while on duty and kept where it will not be exposed to radiation during off-duty hours. A dosimeter should only be worn by the person whose name appears on the plastic holder. The dosimeter is worn for a period of 28 days specified by the monitoring service and then returned promptly for evaluation. The results are sent to the permit holder. The radiation dose to which a radiation worker has been exposed in the wearing period of 28 days is reported in millisievert (mSv). An exposure of < 0,20 mSv is reported as a nil exposure. The dose equivalent limits (DEL) are: Per wearing period Per year: of 28 days: _________ Whole body 4 mSv 50 mSv Extremities 40 mSv 500 mSv The DEL serves to determine the adequacy of protection afforded to workers in radiation areas. The risk associated with exposure amounting to less than the DEL is considered to be very small indeed. However, as risk increases gradually with the dose received, every effort should be made to keep the level of exposure as low as possible. If the DEL for a specific wearing period is exceeded the monitoring service will inform the permit holder by telegram and also notify the Radiation Control section of the Department of Health. The permit holder must file a report containing an explanation of how the "radiation accident" came about with the latter department and the over-exposed person must immediately report for the routine medical examination to which radiation workers are subjected. The permissible accumulated lifetime dose is calculated with the formula 50(N-18) mSv where N is the person's age in years. This means that from age 18 on a person may accumulate a maximum annual dose equal to 50 mSv. If a dosimeter is lost or gets damaged so that it cannot be read by the monitoring service a "notional dose" of 4mSv will be reported on the printout and the monitoring service will charge a stiff replacement fee. LEGAL CONSIDERATIONS The following laws govern the use of radiation equipment: Hazardous substances Act 15 of 1973 (amended April 1989) Public health Act 42 of 1971 (amended August 1973) These deal inter alia with the use of electronic products like x-ray units, their registration and the safety of radiation workers, public and patients. These include: 1. All persons potentially exposed to radiation in the course of their duties must be registered as radiation workers with the Directorate: Radiation Control, Department of Health, P O Box 977, Pretoria 0001. Tel: 012 324 1041. Fax: 012 328 3406 (forms attached). 2. Radiation workers must be registered with a recognized Monitoring Service and be monitored on a regular basis for exposure to radiation e.g. the Radiation Protection Service of the Bureau of Standards, Private Bag X191, Pretoria, 0001. 3. Radiation workers must be medically examined by approved medical practitioners (forms attached): (a) at original registration (b) immediately after being notified by the Monitoring Service that exposure to radiation has exceeded the DEL for a specific period (c) before re-registering after childbirth or a period of unemployment or different employment. Regular annual medical examinations during registration as a radiation worker are no longer compulsory. The onus is on the worker to arrange for periodic examinations at intervals correlating with radiographic workload. Annual examinations are still recommended for those who do a lot of radiographic work but for others an examination once every 3 years should suffice. 4. Dosimeters must be worn by all radiation workers during working hours. 5. Radiation workers must wear protective clothing when doing radiography. 6. Radiation workers must have sufficient knowledge of, and the necessary experience in, the operation of a radiographic unit so that they can use it independently and must be conversant with the potential health hazards involved as well as the safety measures which should be applied to limit these hazards. Unqualified persons using a radiographic unit (e.g. student trainees) should be supervised by experienced radiation workers. 7. Radiation workers are obliged to co-operate with the permit holder (usually the employer) in the application of these regulations. 8. All medical - and radiation reports as well as registration documents of radiation workers must be filed for 10 years by the permit holder. 9. X-ray equipment and premises must be licensed. If disposing of an X-ray machine the Department of Health must also be notified (forms attached). RADIOGRAPHIC APPARATUS AND TECHNIQUE i. THE NATURE AND PROPERTIES OF ROENTGEN RAYS Knowledge of the nature and behaviour of x-rays is the first step in understanding the production of a radiograph. X-rays Definition X-rays are defined as a form of electromagnetic radiation similar to visible light but of much shorter wavelength. Electromagnetic radiation Is a method of transporting energy through space and is distinguished by its wavelength, frequency, and energy. Characteristic of electromagnetic radiation is that it behaves as a particle as well as a wave. Electromagnetic radiation that has a short wavelength has a high frequency. Electromagnetic radiation that has a long wavelength has a low frequency. Wavelength All radiant energy travels in a wave form along a straight path and is measured by its wavelength. In a series of waves, the distance between two consecutive corresponding points on a wave is called wavelength. (Refer to diagram 1.1) Diagram 0.1: Wavelength motion showing two corresponding points on consecutive waves. 4. Frequency Is measured by the number of cycles of the wave that pass a stationary point per second (cycles per second). Diagram 0.2: The waves illustrated differ in wavelength but their speeds are identical. The lower wave (short wave-length) vibrates faster i.e. has greater frequency Electro-magnetic waves differ from one another in their wavelengths and frequencies (number of waves executed per second). The shorter the wavelength the higher will be the frequency and the higher will be the energy level associated with the photons. At a constant speed, wavelength and velocity are thus inversely proportional (velocity=frequency X wavelength). X-rays have very short wavelengths and therefore relatively high energy levels. Electromagnetic spectrum All forms of electromagnetic radiation are grouped according to their wavelength and frequency in what is called the electromagnetic spectrum. (Refer to Diagram 3.3) Diagram 0.3: The electromagnetic spectrum All electro-magnetic rays share the following properties: Electromagnetic rays deliver their energy as a series of separate "packages". These packages of energy move in a wave-like fashion and are referred to as X-ray PHOTONS. They travel with the same tremendous speed (310,000 km/s) They travel in straight lines (even if wavelike!) They transfer energy from place to place through space In passing through matter their intensity is reduced by absorption of some of their energy, as well as by deflections from their original paths (scattering). The matter is said to be exposed or irradiated. They all obey the inverse square law which relates intensity of radiation to the distance travelled from its source of origin In addition to the general properties of electro-magnetic radiation listed earlier, X-rays (Roentgen rays) have the following properties which are exploited in radiography: The ability to cause fluorescence and phosphorescence in various chemical crystals (see fluoroscopy). It was this property that led to their initial discovery in 1895 by Roentgen. The ability to affect photographic emulsions directly (e.g. non-screen film used for industrial purposes). The ability to penetrate objects. The ability to cause biological changes through biochemical effects in living tissues. This property explains their potential danger but also forms the basis of roentgen ray therapy. X-RAY EQUIPMENT The X-Ray unit The control panel It is essential that one is familiar with all components of the face of the panel and understands that most control panels are alike but are not identical. The following is a list of mechanisms found on most x-ray consoles/control panel: On/off switch Provides a closure to the electrical circuit (main supply) to allow the flow of electricity necessary for subsequent exposure. Kilovoltage (kV) selector The unit is calibrated so that the desired kV value can be selected. However, in a number of older x-ray units the kV control is automatically linked with a certain milliamperage. mAs selector The Milli-amperage (mA) selector allows one to select the desired current to the cathode filament. Millisecond selector / Timer The Timer (s) allows one to pre-select the time of each exposure. The timer varies among various models of x-ray units. Most x-Ray units have a combined mA and time (s)selector which allows the operator to select the desires mAs for a specific exposure. Exposure button The exposure button is on the face of the control panel or can be mounted on the cable. The button should be in a position to allow the operator of the unit to be at least 2m from the tube housing when an exposure is made. Many x-ray units operate on a two-stage button. Two stages are necessary for the cathode (filament) to be activated and heated to produce the electrons necessary for the exposure. Depression of the first half of the button activates the filament and rotating anode, if present and after a few seconds, the button is fully depressed to complete the circuit for exposure. Warning light Control panels have a light that illuminates when an exposure is made and x-rays are being emitted. X-Ray generator The tube support The tube support system is designed to permit the x-ray tube to be manipulated to various locations necessary to obtain examination procedure projections and hold the tube immobile during the exposure. Tube suspension systems are available in five versions i Overhead iv Floor ii Floor- to- ceiling v Mobile iii C-arm Diagram 0.1: Floor-to-ceiling suspension of an x-ray tube Protective tube housing The entire tube is encased in a metal housing which is composed of cast steel to prevent the escape of stray radiation and to protect the glass envelope from physical damage. The protective housing is composed of cast steel and is capable of absorbing most of the unwanted photons. The house is usually lined with lead for additional absorption only at the cathode end because of the direction of the photons being emitted from the anode. (Refer to diagram 2.2). The housing is equipped with a window to permit restricted exit for the useful photons from the glass envelope window. Special dielectric oil is used to fill the space between the glass envelope and the tube housing. The oil absorbs much of the heat produced by x-ray production. The protective tube housing controls: Leakage and scatter radiation Isolates the high voltages Provides a means to cool the tube Diagram 0.2 : X-ray tube housing The X-Ray Tube The purpose of the x-ray tube is to produce a controlled x-ray beam. To better understand the x-ray tube, one needs to consider what is necessary for the production of x-rays. Elements necessary for X-ray production: A source of electrons – free electrons must be made available at the cathode A method of accelerating the electrons – at a very high speed in a predetermined direction – towards the anode. An obstacle- free path for the passage of high-speed electrons A dense metallic object placed in their pathway to abruptly slow down or totally stop the moving electrons (anode. A target in which the electrons can interact, releasing energy in the form of x-rays and heat. An envelope to provide a vacuum environment, eliminating the air molecule obstacles for the electron stream and preventing rapid oxidation of the elements The construction of the x-ray tube The x-ray tube is a special type of diode. It is a component of the x-ray unit and the function of the x-ray tube is to produce x-rays. It is contained in the protective housing and is therefore inaccessible. The x-ray tube consists of a cathode (negative electrical charge) and an anode (with a positive electrical charge), each of these is called an electrode, encased in a glass envelope (x-ray tube / Roentgen tube), which is evacuated. Diagram 0.3: Construction of the x-ray tube For the generation of roentgen rays (x-rays) the following 3 basic steps are required in sequence: * Free electrons must be made available (at the cathode) * These electrons must be forced to move at a very high speed in a predetermined direction (towards the anode). * The moving electrons must be abruptly slowed down or totally stopped in a dense metallic object placed in their path of travel (anode). The Cathode The purpose of the cathode is to provide a source of electrons and to direct them toward the anode. It has two primary parts: Filament Focusing cup Diagram 0.4: Illustration of the cathode - filament and the focusing cup Filament The filament in most x-ray tubes measures approximately 0.2 cm in diameter and 1 cm in length. It is mounted on rigid wires that support it and carry the electrical current used to heat the filament. A low–energy circuit heats the filament. Emits electrons when heated. Most modern tubes have two filaments mounted side by side. One is smaller than the other, each having a different capacity for heat and electron emission. o The small focal spot is associated with the small filament. o The large focal spot with the large filament. The amount of energy in the circuit is referred to as the milli-amperage. Focusing cup The filament is located in a concave cup called the focusing cup. It is made of molybdenum because it has a high melting point and is a poor conductor of heat. Since all the electrons accelerated from the cathode to anode are electrically negative, the beam tends to spread out owing to electrostatic repulsion; some electrons can even miss the anode completely. The focusing cup is negatively charged so it condenses the electron beam to a small area of the anode. The Anode The anode is the positive side of the x-ray tube. The two types of anodes 1. Stationary anode 2. Rotating anode 1. Stationary anode Is found in small portable (old) radiography units. These units have relatively small capacity for x-ray production. The primary limitation is its inability to withstand large amounts of heat. Repeated bombardment by electrons and subsequent heat production can damage the target. Diagram 0.5: Stationary anode 2. Rotating anode The rotating anode is in the shape of a disk that rotates on an axis through the centre of the tube. The rotation provides a cooler surface for the electron stream. With the use of a rotating anode heat is distributed over a larger area, yet still provides a small focal spot. Diagram 0.6 : Rotating anode * Focal spot The small area of the surface of the target that is bombarded by electrons during an exposure is called the focal spot. The focal spot is the area of the target from which x-rays are emitted. The size of the focal spot is affected by the size of the filament which is determined at the time of tube construction. The size of the focal spot has an important effect on the formation of the image. Recommendations for an extended tube life Warm up the anode following manufacturer’s recommendations. Do not hold the rotor switch unnecessary. Do not make repeated exposures near tube loading limits. Do not rotate the tube housing rapidly from one position to another. X-ray tube filters X-ray units have metal filters, usually 1-3 mm aluminium, positioned in the primary beam. Types of filtration 1. Inherent filtration 2. Added filtration Thin sheets of aluminium attached between the protective tube housing and the collimator. * Total filtration Is equal to the sum of inherent and added filtration. Diagram 0.7: Total filtration in X-Ray generator Collimator The beam emerges from the x-ray tube in a diverging manner. If uncontrolled, the beam could extend to considerable width. Many x-ray units incorporate a lead plate or cone over the aperture of the tube to alter the size of the x-ray beam. A more versatile method of collimation is by means of adjustable lead shutters, which are permanently attached to the tube housing, correlating with the tube window. Knobs located on the collimator allow for adjusting the field size. (Refer to diagram 3.11 The collimator is a restricting device utilized to control the size of the primary x-ray beam. Diagram 0.8: Collimator with lead shutters. (More about collimators under control of scatter radiation) Radiography tables Radiolucent table The radiographic table is designed to support the patient in a position that will enhance radiographic examination. The table top must be uniformly radiolucent to easily permit x-rays to pass through. The table top must be easily cleaned, hard to scratch, and without crevices where radiographic contrast media can accumulate. The table must include space for a tray to hold image receptor and a radiographic grid. The tray is often called a” Bucky- tray” in honour of Dr Gustav Bucky (1880-1963). Diagram 0.9: A diagnostic radiographic floating top table Other tables Wooden (or other material) tables covered with a lead lining is sometimes used for the purpose of radiograph making. When these tables are used, it should have the same properties than the Bucky-table with regards to patient support, cleaning and disinfecting. The table will not have a build-in radiographic grid and image receptor tray and therefore the patient, radiographic grid and image receptor are all used table-top. The Process of x-Ray generation The exposure button is on the face of the control panel or can be mounted on the cable. The button should be in a position to allow the operator of the unit to be at least 2 m from the tube housing. Many x-ray units operate on a two-stage button. Two stages are necessary for the cathode filament to be activated and heated to produce the electrons necessary for the exposure. Depression of the first half of the button activates the filament and rotating anode, if present and after a few seconds, the button is fully depressed to complete the circuit for exposure. Stage 1: (exposure button halfway in) In the x-ray tube free electrons are made available through heating of the filament (cathode) by passing an electric current (known as the filament current) through it. When a metal is heated sufficiently its atoms acquire thermal energy and loosely bound electrons in the outer shells are "boiled off" i.e. will become agitated enough to become temporarily separated from the atoms. This continuous "boiling" process is known as thermionic emission and the loose ions are called thermions. A large number of thermions are liberated in this process. They crowd together in the immediate vicinity of the filament and are collectively referred to as the space charge. The number of electrons freed, and thus the size of the space charge, will be determined by the degree to which the filament is heated. The latter in turn is determined by the strength of the electric current passed through the filament. In order to provide a useful number of thermions the filament must be heated to at least 2200 °C. The electrical circuit through the filament incorporates a step-down transformer which lowers the mains-voltage from 250 volts to about 10 volts, and a milli-ampère selector for selecting the strength of the filament current. Stage 2: (exposure button completely in) The instant when the exposure button is activated the freed electrons will be forced to move at a very high speed from the filament to the anode as a result of a high potential difference which becomes effective between these electrodes. Potential difference is defined as the difference in electrical potential energy (or stored energy) existing between two points in an electric circuit due to an excess of electrons having accumulated at one point and a deficiency of electrons at the other. The point in a circuit where the electrons accumulate has a negative potential and the point where a relative deficiency of electrons exists has a positive potential. Potential difference is the sum total of negative and positive potentials, or the difference between two negative potentials. The potential difference between a negative potential of 10 volt and a positive potential of 10 volt is 20 volts, and the potential difference between a negative potential of 30 volts and a negative potential of 10 volts is also 20 volts. Electrons will, when given the chance, always flow towards the point with the weaker negative potential (the "positive" side). Electron flow constitutes an electric current. In the switched-on roentgen apparatus the potential difference delivered by the generators will be located on the mains-supply side of the exposure button. Only when the exposure button is activated (thereby closing the electrical circuit and providing a pathway along which this accumulated energy can be channeled) will the potential difference move instantaneously through the step-up transformer, where it is boosted to kilovolts, and then on to the cathode (negative potential) and anode (positive potential) of the roentgen tube. This potential difference created between the two electrodes will instantaneously force the previously freed electrons constituting the space charge from the filament across the short gap to the anode thereby causing an electric current (known as the tube current) to flow across the gap. The discharge causes a spark between the two electrodes. The potential difference or force moving the electrons across the gap is maintained for the duration of the timed exposure by the generators in the power station. The freed electrons constituting the space charge are not responsible for the negative potential created at the filament. This is the function of the potential difference caused by the generators at the power station. The thermions only serve as carriers of the electrical current across the gap during the exposure. The speed with which the electrons move is related to the magnitude of the potential difference operative in the roentgen tube during the radiographic exposure. The greater the difference the faster will they move and vice versa. A potential difference of at least 25 kV (25000 volts) is required for X-ray generation. To achieve these very high voltages the incoming line voltage of the mains supply (normally 220 - 250 volts) must be boosted by a step-up transformer incorporated in the electrical circuit of the X-ray apparatus. The electrons moving across to the anode are focused into a thin stream by the negatively charged focusing cup around the filament (negative charges repel negative charges !) and strike only a very small surface area of the target (± 1 mm2) known as the focal spot. The level of the latter is marked on the outside of the tube-housing by a small red dot. The smaller the focal spot the better (see penumbra). Smaller and cheaper roentgen units tend to have relatively large focal spots. When reaching the target the electrons are slowed down abruptly or completely stopped as the result of different interactions with atoms of the target and the kinetic energy which they acquired as a result of the potential difference is transformed into two different forms of energy namely X-ray photons (1%) and heat (99%). The intense heat produced by this process is the main limiting factor of X-ray production. The target consists of tungsten (Wo) which has the highest melting point of all metals and can therefore tolerate the most heating before melting. In cheaper stationary anode machines the heat in the target is conducted away from it by the copper rod, into which the target is embedded, and transmitted to the oil between the X-ray tube and the tube-housing to cool down. Higher output machines have a rotating anode (and thus tend to make a noise) which has 3600 r.p.m. This means that any point of the target is only bombarded by electrons for 1/60th of a second before rotating away to cool down for 59/60th of a second. The mechanics of rotating anodes is complicated to manufacture making these machines very expensive for ordinary veterinary practice. However, their high output capabilities result in superior radiographs and many practices buy second-hand rotating anode machines. The x-ray beam The roentgen rays leaving the tube-housing through the port collectively form the primary beam. The x-ray beam may be divided into two parts: Primary radiation It is radiation from the tube without interaction with matter. It is confined to the portion of the x- ray beam emitted from the x-ray tube. It is not altered in its quality as it approaches the object being examined. Remnant radiation (Secondary radiation) Primary radiation is the x-rays that come from the target of the x-ray tube. Secondary radiation is x-radiation that is created when the primary beam interacts with matter. Remnant radiation is that radiation that emerges from the body tissues to reach the image receptor and record an image (also referred to as image-forming radiation). Remnant radiation also contains secondary radiation, the amount depending upon its wavelength and the manner in which it is controlled. The total intensity of the remnant beam striking the film is about one-fifth of the intensity of the primary beam. 15% out of the 20%, of the remnant radiation striking the film is secondary radiation produced in the subject (patient). Intensity of Radiation in the primary beam (Inverse square law) The term intensity of radiation refers to the number of X- rays which will reach (or pass through) an imaginary unit surface area, placed perpendicularly to the direction of the rays at any given point in the beam, per second. The larger the number of X-rays reaching the unit surface area the higher will be the intensity at that point and vice versa. X-rays follow divergent paths, i.e., spread further and further apart, as they travel away from the focal spot where they originate. It follows that the rays present in the primary beam will irradiate an ever increasing surface area with increasing distance from the focal spot. The intensity of radiation must therefore decrease progressively because the same number of rays has to cover an increasing number of unit surface areas. Diagram 0.10: Inverse square law The decrease in intensity of radiation takes place in accordance with the inverse square law which states that the intensity of radiation (I) is inversely proportional to the square of the distance (D) travelled from its source: Diagram 0.11: Radiation intensity decrease From the above illustration it becomes clear that the intensity of radiation is reduced in a predictable manner: Increase in distance: Radiation intensity decrease at new D relative to initial D doubled (10 to 20 cm) (0.0025/.01) = 0.25 = 1/4 = 1/2x2 trebled (10 to 30 cm) (.00111/.01) = 0.11 = 1/9 = 1/3x3 quadrupled (10 to 40 cm) (.000625/.01) = 0.0625 = 1/16= 1/4x4 fivefold (10 to 50 cm) (.0004/.01) = 0.04 = 1/25= 1/5x5 Decreasing the distance will similarly increase the intensity of radiation in a predictable manner: Decrease in distance: Radiation intensity increase at new D relative to old D halving (40 to 20 cm) (.0025/.000625) = 4 times one quarter (40 to 10 cm) (.01/.000625) = 16 times a. EXPOSURE FACTORS Before any radiographic exposure can be made the exposure factors (kV, mA, s) have to be selected by adjusting the appropriate selectors on the control panel of the unit. In order to produce x-rays, suitable electrical currents must be supplied to both the cathode filament and field between the cathode and anode. Radiographic exposure charts based on the ranges of thicknesses of the various anatomical regions should be compiled and regularly updated. A typical exposure chart will contain the following variables / columns: Region of interest (anatomical region) Various thicknesses of a specific anatomical region in cm, Columns showing the kV, mA, and s, (or mAs) settings, Columns to say what type of image receptor must be used Column to indicate if a radiographic grid (CR / DR) must be used By changing the 3 exposure factors [kV, mA, and s, (mAs)] we predetermine the QUANTITY and QUALITY of the X- rays that will be produced by the roentgen tube and it is important to understand clearly what the mechanisms are that are brought into action when these factors are selected. The quality of an x-ray beam is determined by its penetrating power. Radiation of shorter wavelength has increased penetrating ability. This is determined and controlled by the kV (kilo- Volts) required for a specific exposure. The quantity or intensity of an x-ray beam is defined as the amount of energy flowing per second through a unit area perpendicular to the direction of the beam. The mAs selected for a specific exposure determines the quantity or intensity of the primary x-ray beam. The quantity (mAs) and quality (kV) of the x-ray beam are affected by various factors: The spectrum of radiation produced by the x-ray generator. The voltage potential used to generate the x-rays (kVp). The amperage used to generate the x-rays (mA). The exposure time. The quantity and quality of the x-ray beam reaching the image receptor are affected by various factors: The distance between the radiation source and the film. (SID – source to image distance) The material (tissue type) of the component being radiographed. The thickness of the material that the radiation must travel through. The amount of scattered radiation reaching the image receptor. Milliampere-seconds (mAs) The quantity of x-rays required for a given exposure is the product of the current (mA) and time (s) in milliampere-seconds (mAs). These two exposure factors directly control the quantity of x-rays produced and therefore the amount of radiographic density. The quantity of radiation produced during an exposure plays a vital role in: radiographic density (image resolution) of radiographs quantity of scattered radiation formed radiation safety Milliamperage (mA) The electrical current that heats the filament is measured in mA. Heating the cathode filament, produces electrons. As the mA is increased, the number of electrons available is increased. Advantages of using a high mA value Higher mA setting allows shorter exposure time setting with the same number of x-rays produced. A shorter exposure time setting, avoids motion blur, thus reducing the possibility of repeating a radiograph. Greater amount of x-rays is produced and it allows examination of thicker anatomic areas of the patient. Influence of mA setting on the focal spot On some control panels the operator selects focal spot independently from the mA station. On other x-ray units, the focal spot and mA station is automatically selected. When a smaller body part is radiographed, it is advised that a smaller focal spot is used. Selecting a smaller focal implies that a smaller mA station (tungsten filament) is used during x-ray production. Thus, when a large area is radiographed, a large focal spot and larger mA station (tungsten filament) is used for x-ray generation. Example A small focal spot cannot be used on every radiograph because the small filament cannot produce the higher mA that should be used for most radiographic exams performed. Trying to heat a small filament using a high mA can cause the filament wire to burn and break. A small focal spot is usually used on small body parts like the carpus, tarsus, metacarpus, nasal bones, etc. A small focal spot is also used to enhance recorded detail. Thorax, abdomen, spine, skull, and gastrointestinal work all require high mA and therefore a large focal spot. Time (s) The period during which the x-rays are permitted to leave the x-ray tube is termed exposure time. The total quantity of x-rays produced during an exposure is also dependent on the length of exposure (time). To calculate mAs values On some control panels the mA and time (s) are selected independently and the mAs is not shown. Other control panels show the mAs value and s value and not the mA value. mAs = current (mA) x time (s) Example: If the mA is kept constant and only the time setting is changed, the intensity / quantity of x-rays in the primary x-ray beam changes. 400 mA X 0.01 s = 4 mAs 400 mA X 0.025 s = 10 mAs If the time is kept constant and only the mA is changed, the intensity / quantity of x-rays in the primary x-ray beam changes. 200 mA X 0.02 s = 4 mAs 500 mA X 0.02 s = 10 mAs Reciprocity law A variety of different mA and time settings could produce the same mAs and therefore the same beam intensity. This law works well most of the time, except when very high or very low time settings. See examples below. Examples 400 mA x 0.05 s = 20 mAs 50 mA x 0.4 s = 20 mAs Clinical applications To use shortest possible exposure time To control motion Adjust when using a small focal spot size The quantity of radiation produced during an exposure plays a vital role in: Image resolution / image quality quantity of scattered radiation formed radiation safety Kilovoltage During an exposure, the anode is maintained at a very high positive electrical potential relative to the cathode. Because of this difference in electrical charge, the electron-cloud at the filament is formed into a narrow beam and accelerates toward the anode at a very high speed. The kinetic energy of the electrons when they reach the target is proportional to the potential difference placed between the anode and the cathode. The potential difference between the anode and cathode, is measured in kV. Another term commonly used is kilovoltage peak (kVp). The word “peak” indicates the maximum energy available at that kV setting. kVp determines the quality of x-ray beam and thus, its ability to penetrate tissue. A change in kV has a number of effects Results in change in the penetrating power of the beam. An increase in kVp results in, shorter wavelength x-rays produced. As the penetration is increased, radiographic contrast / image quality is altered as a result of excessive penetration of the object and the resultant scatter radiation with ‘higher energy’ and direction closer to that of the x-rays in the primary beam. High kVp = high photon energy = low contrast = long scale Low kVp = low photon energy = high contrast = short scale Radiographic contrast Definition: Radiographic contrast is the density difference between two adjacent areas on a radiographic image. The type of contrast desired for each radiograph, is dependent on the anatomic area. Radiographic contrast is influenced by Subject contrast kVp level Scatter radiation Contrast and grey scale Short scale contrast When the density difference between two adjacent areas is great, the radiograph is said to have short gray scale / high contrast. Example: That is, a radiograph with short gray scale / high contrast exhibits black and- white tones (Radiographs of abdomen and extremities). Long scale contrast A radiograph that exhibits many shades of gray and a small density difference between two adjacent areas has a long gray scale / low contrast. Example: A radiograph that exhibits increased number of gray tones between black and white tones (e.g., thoracic radiographs). Subject contrast Definition: It is the degree of differential absorption resulting from the differing absorption characteristics of tissue types, e.g. the different density of bone, soft tissue and gas. Subject contrast is dependent on the specific characteristics of an individual patient’s tissues, disease, age and body condition. The kVp can be estimated utilizing an equation (Santè’s rule): kV = (2x cm [body part thickness ]) + 30 Abdominal Radiographs kV = (2x cm [body part thickness ]) + 40 Bone kV = (2x cm [body part thickness ]) + 70 Thoracic Radiographs (CR) The type of imaging system (computed radiography (CR) or direct digital radiography (DR)) might have an effect on the preferred kV and mAs to be used for a specific exposure. Due to the different sensitivity of the image receptors, some DR systems requires less energy (kV and mAs) to obtain good radiographic images. Relationship between mAs and kV mAs controls beam intensity / image resolution, kV controls contrast but also affects image quality. A primary consideration when one selects exposure factors is the kV, because the kV controls the quality of the beam and its penetrating power. The kV selected should be the optimum kV for the body part being examined. Optimum kV is the kV that will be able to penetrate the body part, produce sufficient radiographic contrast and produce an acceptable level of scatter radiation. When excessive levels of kV are used, unwanted radiation becomes visible. An increase in penetration of the beam through the patient makes the remnant beam more intense, similar to an increase in mAs. Therefore, excessive kV levels both overexpose and decrease the radiograph, resulting in poor image quality. Exposure latitude Exposure latitude can be defined as the range in mAs values that can be chosen from that will produce acceptable resolution of images for diagnostic purposes. An acceptable radiograph, from the image resolution point of view, will be produced regardless of which mAs value within the range is chosen. Exposure latitude will vary with the kV applied and the contrast scale produced. Depending on the number of acceptable mAs values falling within these limits, we distinguish between narrow and wide exposure latitudes. Narrow exposure latitude With a narrow exposure latitude, the number of suitable mAs values from which one can choose is very restricted and they are of a relatively high value, e.g. 55 and 60 mAs. It is associated with low kV values and short grey scale / high contrast technique. Wide exposure latitude A wide exposure latitude signifies a wide choice in mAs values and is associated with high kV values and a long grey scale / low contrast technique. The mAs values are low e.g. 2, 3, 4, 5, 6, 7, or 8. THE BEHAVIOUR OF THE PRIMARY BEAM AND IMAGE RECORDING a. Distances affecting beam intensity / image quality: The central ray The photon in the exact center of the beam is called the central ray. The angle of the beam is measured at the central ray rather than at the edges of the beam. The central ray should interact at 90˚ with the object and image receptor. Distances Source to image distance (SID) Is the distance between the focal spot (source of x-rays) and image receptor. The most common used SID is 100 –110 cm With older portable units the SID used is 90cm (e.g. at OVAH using the mobile unit). The distance from the x-ray tube to the image receptor (SID) is very important because it has substantial influence upon three radiographic image qualities. Radiographic image density/ resolution Distortion Sharpness of recorded detail A change in the distance is similar to change in mA in its effect on the overall intensity of the beam. Radiographic density is affected by a change in distance. Only a 20% change in distance will cause a visible change in image quality. When the SID is changed, the total amount of x-rays must be increased or decreased in order to make a comparable exposure employing the new distance. An increase in SID, with a set OID (object to image distance), decreases the density by the ISL (inverse square law). A decrease in SID, with a set OID, increases the intensity by the ISL To calculate mAs for change in SID, use the following formula: New mAs = Old mAs x New distance2/old distance2 Object to image distance (OID) The distance between the object and the image receptor The patient is inelegantly referred to as the object in distance terms. The patient’s body is a three-dimensional structure. If the radiographer is interested in producing a radiograph of just one part inside of the patient, then that part becomes the object. The object must always be in close contact with the image receptor. Source to object distance (SOD) The distance from the source to the object INTERACTION BETWEEN X-RAYS AND MATTER The primary X-rays reaching the patient are collectively referred to as the incident radiation. After entering the patient's body the incident rays may or may not interact (collide) with the atoms in the body. Those that do not collide will pass through (penetrate), while those that do collide will either be absorbed or scattered depending on the nature of the collision. Those rays that penetrate together with those scattered rays that emerge from the far side of the patient's body collectively constitute the remnant radiation. The quantity of remnant radiation is always less than that of the incident radiation due to the attenuation (weakening) of the primary beam by absorption of roentgen rays. Penetration The fact that X-rays can pass through a body without colliding with a single atom is made possible by the fact that matter consists mainly of space. X-rays are also electrically neutral and will therefore not be attracted by the atoms through which they are passing. The interrelationship existing between the kV used in the propagation of X-rays and the energy level of the rays produced is of crucial significance when considering both the absorption and penetrating power of X-rays. As the kV increases so absorption of X-rays and attenuation of the primary beam will decrease and penetration improve. Conversely, as the kV decreases so absorption of X-rays and attenuation of the primary beam will increase and penetration decrease. Absorption The terms absorption and penetration of X-rays are mutually exclusive in the sense that a roentgen ray can either be absorbed or penetrate but not both. Absorption takes place when X- ray photons collide with tightly bound inner shell electrons of atoms. In this collision all of the photon's energy is expended and the ray ceases to exist. The electron struck by the photon is dislodged from the atom. Replacement of the dislodged electron by substitution with an electron from the adjoining outer electron shell, and the chain reaction of electron substitutions which follows peripherally, causes the emission of secondary rays from the atom. These secondary rays are extremely soft (have long wavelengths and very low energy values) and become totally absorbed in the surrounding tissues of the body. The expended energy absorbed in the tissues of the body causes biochemical changes in the cells which absorbed it. If sufficient energy is absorbed the cells will die. This is the basis of radiation therapy. Differential absorption of x-rays To what extent X-rays will become absorbed in different tissues of the body or be able to penetrate them depends on the interaction between the energy levels (wavelengths) of the rays and the atomic number (Z), density and thickness of the various tissues through which the rays have to travel. Different tissues are composed of different combinations of elements e.g. calcium and phosphorous in bone, or hydrogen and oxygen in water, fat, muscles, etc. The atoms of any specific element can only absorb (or "capture") X-rays below a certain energy level which is specific for that element. If the energy possessed by the rays exceeds this level the rays can no longer be absorbed by that specific element and they will succeed in penetrating it. Conversely stated, the penetrating power of every specific energy level of X-rays has a definite limit. The atomic number of any element is the factor which determines the ceiling of penetration or the level of energy of rays required to penetrate it. As the atomic number increases, e.g. silver (47), iodine (53), barium (56), lead (82), so the energy level of rays must increase in order to penetrate. From the above explanation it follows that X-rays produced by low kilovoltages (long wavelengths, low energy levels) will be easily absorbed by most atoms including those with low atomic numbers, whereas x-rays produced by higher kilovoltages will increasingly succeed in penetrating atoms with higher atomic numbers. As the various tissues in the body are composed of a mixture of elements their average atomic numbers are used for purposes of comparison. Bone (average Z = 14) will absorb X-rays which easily penetrate soft tissues (average Z = 6) whereas barium (Z = 56) will absorb rays which have succeeded in penetrating bone. Although air, fluid and soft tissues all have approximately the sa