Psych 120B Notes Pt 1 PDF
Document Details
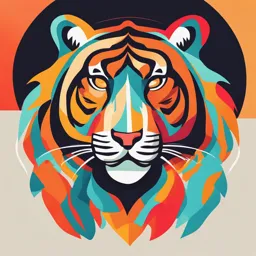
Uploaded by GuiltlessAcer2356
UCLA
Tags
Summary
This document provides lecture notes for Psych 120B, covering various aspects of perception, including sensation, neuroanatomy, psychophysics, and the visual system. Topics discussed include visual transduction, phototransduction in rods and cones, and psychophysical methods like absolute and difference thresholds.
Full Transcript
Lecture 1: Introduction Perception does NOT represent the real world 1. Everything we perceive is not real (ex. illusions) 2. Not everything that is real is perceivable (ex. change blindness) 3. What we do perceive can be distorted (ex. color, brightness, size)...
Lecture 1: Introduction Perception does NOT represent the real world 1. Everything we perceive is not real (ex. illusions) 2. Not everything that is real is perceivable (ex. change blindness) 3. What we do perceive can be distorted (ex. color, brightness, size) Lecture 2: Neuroanatomy and Psychophysics S&P Sensation: detecting physical energy (ex. light) from the world and encoding it into neural signals (transduction) Sending of raw data/information to the perception (typically a bottom-up process) Transduction: conversion of an external signal (energy, chemical) into a neural signal (electrical potential) → the type of energy and information transduced are going to shape our perceptions Perception: selecting, organizing, and interpreting sensory information, allowing us to recognize meaningful objects and events There is an ongoing debate about where sensation ends and perception begins Perception is an active process, not a passive one (makes sense of input → imposes meaning to the input → organizes the input) Top-down processes: higher cognition (knowledge, memory, reasoning) influence lower-level processes (such as perception) Neuroanatomy Neurons: Dendrites: detect incoming signals Cell body: nucleus and cellular machinery Axon (and terminals): carries electrical signals and transmits signals to other neurons Brain anatomy: Occipital: visual and processing regions Temporal: auditory processing regions Parietal: somatosensory processing (touch) Frontal: olfactory (smell) processing, gustatory (taste) processing Scientists can measure neural activity through: EEG, MEG, fMRI, single-cell recordings Psychophysical measurements Psychophysics: study of sensations evoked by physical stimuli (ex. light sensitivity, mechanical pressure) Accuracy and reaction time (RT) are typically the dependent variables Useful for sensory limits (ex. what is dimmest light you can detect?) and relative judgments (ex. which object is farther away?) Thresholds: stimulus intensities where participants can just detect a stimulus/just tell apart two stimuli → this is probabilistic Sensory limits → absolute thresholds (ex. what is the weakest light someone can see 50% of the time?) Relative judgments → difference thresholds (ex. how much brighter doe sa light have to be for someone to notice 50% of the time?) Psychophysical methods Constant stimuli: a predetermined set of trials to categorize what stimulus strengths people can detect and where the transition is Light detection example: 1. Experimenter pre-sets different stimulus strengths (strong, weak, and intermediate values) 2. Experimenter presents many trials of each of the strengths in a random order 3. Responses are fit to a curve to find the intensity that corresponds to 50% chance of accuracy (look at x-value for a 50% detection rate in graph) Limits: participant starts with a strong or weak stimulus, and researchers increases/decreases it until the participant says they can no longer detect it Light detection example: brighten or dim a light spot and ask the participant if they can see it at each adjustment, then average the intensity where the participant’s response changed Adjustment: present participant with a strong/weak light and let them adjust it until it is visible/not visible (participant is in control of adjusting the stimulus), then take the average of where their answer changes Similar to limits JND Just-Noticeable-Difference (JND): smallest difference in stimulus strength needed to discriminate (tell apart) two stimuli Original → Weber’s Law: JND changes as the intensity of the stimulus changes, but the proportion remains the same JND gets larger as intensity increases (we need larger and larger differences to notice something has changed/we are less sensitive to change as strength increases) Backpack example: when your backpack is full, you are less likely to notice if someone puts something in it Revision #1 → Fechner’s Law: revision of Weber’s Law to demonstrate sensory magnitude; shows that we get less sensitive to change as stimulus strength increase P = perceptual or sensory magnitude S = stimulus intensity (physical) K = constant = Weber’s and Fechner’s laws cannot capture relationships where perception is veridical to physical change or cases where perceived intensity is greater than the physical change Revision #2 → Steven’s Law: more flexible relationships captured P = perceptual or sensory magnitude S = physical stimulus intensity K = constant n = exponent, specific to each sensory dimension ○ If n1 → exaggeration Compression: less sensitive to change as stimulus strength increases (ex. brightness) Veridical: accurate perception of physical changes in the stimulus (ex. pencil length example) Exaggeration: become more sensitive to change as stimulus strength increases (ex. pain) How to fix response issues If participant has bias or a strategy, it can impact their threshold Catch trials: trials where the experimenter does NOT show the target stimulus to get a sense of how biased the participant is Discussion Section Notes Psychophysics: (1) choose some aspect of perceptual processing, (2) design stimuli to engage that process Absolute threshold: intensity of some stimulus to be detectable some percentage of the time Difference threshold: Signal Detection Theory SDT: common analytical framework in psychological research that separates sensitivity from response bias Sensitivity: perceptual performance (how sensitive are you to this perceptual input?) Criterion: measure of bias (are you being conservative or liberal when making your perceptual decisions?) Signal trials vs. Correct rejections + false alarms = 1 SDT Graph: Two distributions for activation: (1) Noise and (2) Signal + Noise ○ Noise: random brain activity that is not related to a signal ○ Signal: neural activity increased due to a signal Distance between distributions is sensitivity (d’) Criterion: the participant’s Yes/No cutoff on the graph ○ Participants respond “Yes” to sensory signals stronger than criterion ○ Participants respond “No” to sensory signals weaker than criterion Sensitivity changing: Higher sensitivity (d’): easier to tell apart the noise and signal → distributions move farther apart → decreases false alarms and misses Lower sensitivity (d’): harder to tell apart signal and noise → distributions move closer together → increases false alarms and misses Criterion changing: Conservative criterion: criterion is strict; want to be very sure the signal is present before responding → criterion line shifts right → hits and false alarms decrease Liberal criterion: you want to catch the stimulus any time it appears → line shifts left → hits and false alarms increase Lecture 3: Visual System Anatomy & Physiology Anatomy and physiology Light Light: the physical energy system that your visual system responds to The visual system only responds to a narrow band of light (400-700 nm) Refraction: light typically travels in a straight line (law of optics) but can be bent when it passes through curved lenses (refraction) Humans’ cornea and lens refract light from the environment to coverage on the back of your eyeball (retina) Accommodation: the process of focusing the lens on an object so that the light coverages on the retina (happens if the lens is focused on an object) Done by contracting or relaxing the ciliary muscles that are attached to the lens Important because light rays reflected from an object typically diverge, so the lens has to converge the diverging lights Retina image inversion: top-down and left-right inversion due to the way light travels and bends in the eyeball Visual field: the full region you can see with both your eyes Your left eye generally sees more on the left side of the space, and the right sees more of the right side of the space Regions overlaps and there is left-right inversion Visual hemifields: hald of your visual field Left side of space projects to the right side of your retina Right side of space projects to the left side of your retina Information from the light of the world → right half of the retina → travels to the right brain ○ Crosses over at the optic chiasm Phototransduction Receptors: cells that pick up information from the environment → for visual system, detect light and start the transduction cascade Visual receptors = rods and cones Phototransduction: visual transduction (a chemical transduction) Light energy causes a chemical reaction: changes the shape of a pigment molecule (isomerization) → isomerized molecules begin a chemical cascade that changes the electrical potential/polarization of the cell A single photon is enough to start this cascade (cones and rods are very sensitive) Retinal cross-section pathway: Rods & cones (receptors) → bipolar cells → ganglion cells (cells that fire action potentials) ○ Light is coming from the bottom because the rods & cones are at the back of the retina → light must penetrate the other cells to hit the rods & cones Cross-section of the vertebrate eye: (1) Cornea and lens for refraction (2) Retina at the back of the light, to detect light ○ Macula: section of retina that detects the light right in front of you → represents central vision The blind spot: area of eye that does not have photoreceptors (about 3 degrees in diameter & gets larger as you age) Reasoning: the photoreceptors are at the back of the retina, whereas the ganglion cells are at the front, but the axons and the ganglion cells need to leave the eye to pass information to the brain ○ The way biology has solved this is to bundle up the axons and have them leave the eye in one place (the optic nerve) ○ There are no photoreceptors where the optic nerve leaves the eye so you can’t pick up light in this space Implications: the filling-in phenomenon is not unique to the blind spot — the brain “doctors” certain images that are projected onto the retina Angular human field of view: Horizontal range: ~180 degrees (140 degrees that is binocular, 40 degrees outside of that on each side that is monocular) Vertical range: 60 degrees above horizontal meridian, 75 degrees below the horizontal meridian Fovea: central vision, almost entirely populated by cones; only 2 degrees in diameter 1% of your retina, but about 50% of your visual cortex processes this region Para-fovea: 4-5 degrees immediately surrounding your fovea (decent spatial resolution) Periphery: anything beyond this region of the retina (lots of rods) Eccentricity: vision is more detailed in the middle of your field of view than the edges (processing of features like details, color, and motion all vary with eccentricity) Differences between rods and cones: (1) cones support color vision while rods do not, (2) cones are most densely packed in central vision, rods are common in periphery Rods and Cones Dark adaptation mechanisms: (1) Pupil dilation: the pupil will dilate in the dark to allow the light to enter the eye (2mm pupil → 8mm pupil) (2) Photopigment bleaching: the photopigments in rods and cones will bleach (become transparent) in bright light to reduce sensitivity and absorb less light → Photopigments are no longer fully functional (a) Bleaching helps with maintaining contrast in the eye (critical because Ganglion cells code for contrast) (b) Cones: adapt faster to changes in light (about 3-4 minutes) but don’t reach the same level of sensitivity as rods (c) Rods: much longer to adapt (around 20-30 minutes) but become much more sensitive to light than cones after complete adaptation The Rod-Cone break: the point where rods become more sensitive than cones when adapting to dark lighting conditions (rods are better at detecting low light overall/they have higher sensitivity) In the dark, cones aren’t as sensitive and can’t get enough color information, but rods contribute since you can still probably get other basic visual information Acuity-Sensitivity tradeoff: acuity (detail) vs. sensitivity (low-light vision) Central vision: higher acuity (spatial resolution) and lower sensitivity ○ Higher concentration of cones → less convergence on ganglion cells (likely each cone has their own ganglion cell) Peripheral vision: lower acuity and higher sensitivity ○ Higher concentration of rods → higher convergence on ganglion cells (they pool their outputs to ganglion cells more than cones) Ganglion Cells Ganglion cells: the first cells that fire action potentials — ganglion cell axons are what are gathered together and exit the back of the eye as the optic nerve Center-surround structure: the center and surround of the receptive field respond preferentially to opposing stimuli On-center ganglion cell: Off-center ganglion cell: Lateral inhibition: cells that are next to each other inhibit each other's activity Photoreceptor cells (rods & cones) in the surround send signals to horizontal cells, which in turn send inhibitory signals to the cells in the center of the receptive field → inhibition prevents the center from being too active when the surround is activated (and vice versa) Significance of center-surround organization: this organization means that ganglion cells can respond preferentially to different (1) spatial frequencies, (2) phase frequencies Spatial frequency: luminance (brightness) changes per unit length Low spatial frequency → fewer light/dark changes happening per unit width of the display Fourier analysis: all signals (images) can be mathematically considered as a summation of sine waves of different spatial frequencies ○ By using Fourier decomposition, images can be broken down into their component spatial frequencies (by subtracting certain sine waves, we can alter the appearance of images) Must have both the high and low-frequency components of a signal to perform accurate analysis ○ High frequencies → sharp edges, details ○ Low frequencies → overall shapes, blobs ○ Relation to ganglion cells: the receptive fields of ganglion cells vary in size, but the center-surround structure means they respond well to sine wave gratings Phase sensitivity: ganglion cells are also sensitive to the phase of a light stimulus Phase: where a peak or trough of a wave (like a sine wave) falls on the receptive field The position of the wave’s peak relative to the center and surround affects how the ganglion cell responds Leaving the retina: the axons of ganglion cells leave the eye through the optic nerve → then they go to the optic chiasm (~60% of the axons cross here) → then the axons travel to the lateral geniculate nucleus (LGN) Lecture 5: The Visual Stream & Color Vision Leaving the retina → the LGN Pathway to leave the retina: 1. Axons of ganglion cells leave the eye through the optic nerve 2. Then they go to the optic chiasm (60% of the axons cross here; nasal axons cross over to meet temporal axons that do not cross) 3. Then they travel to the lateral geniculate nucleus (LGN) LGN: relay center in the thalamus This has topographic mapping: information close in physical space/on the retina is also close in structure. Magnocellular layers: receive input from M ganglion cells ○ Larger receptive fields ○ Primarily rods ○ Bottom two layers Parvocellular layers: receive input for P ganglion cells ○ Smaller receptive fields ○ Primarily cones ○ Top four layers Visual Cortex (V1/Striate Cortex) Organization of V1: (1) Mapped topographically: adjacent visual information is represented in adjacent cellular columns (2) Cortical magnification factor: foveal representations are “expanded” relative to peripheral representations → fovea is a tiny part of the visual field but a large part of V1 Cells neurons respond to: (1) Simple cells: respond to bars of light at a preferred orientation (2) Complex cells: preferred orientation + motion (3) End-stopped cells: orientation + motion + length Simple cells: respond to edges/bars of light in specific orientations Simple cells are phase-sensitive (the location of the light patch on the receptive field matters) Different receptive field structure than the center/surround in the LGN Orientation tuning: simple cells have bars of excitatory/inhibitory activity → edges/bars of light that align with the cells preferred orientation activate the cell more strongly ○ Orthogonal light will have the lowest firing rate ○ Orientations between optimal/least optimal will partially activate the cell Complex cells: orientation specific (like simple cells), but most active when oriented stimuli are moving Some cells respond to motion in any direction, some respond to motion in only specific directions Complex cells are phase invariant (the location of the light patch in the receptive field doesn’t matter End-stopped cells: similar to complex cells, but only respond to lines of a certain length They are: (1) orientation specific, (2) motion specific, (3) length specific Organization of V1 V1 is organized into columns of tissue: each column represents various features of visual information 3 types of columns: (1) orientation columns, (2) location columns, (3) ocular dominance columns Orientation columns: which orientation of light is present in this part of the space The columns of cells all prefer light in the same orientation Aligned perpendicular to the cortical surface Location columns: columns of neurons that have their receptive fields in roughly similar locations of the retina Run perpendicular to the cortex ALL of these different types of columns are organized systematically into hypercolumns Hypercolumns: complete set of orientation and ocular-dominance columns for a given retinal location Each hypercolumn processes every possible orientation and input from both eyes for that specific region of space → complete visual representation Beyond V1 → Estrastriate cortex V2: illusory contours V3: uncertain function, but possible to combine information to construct 3D percepts V4: color V5/Area MT (medial temporal): motion IT (inferior temporal): shapes, forms, faces Cells in this cortex respond to patterns This cortex also has columnar organization Stream for leaving V1 for further processing: → dependent on which type of ganglion cell initiated the signal M ganglion cells (larger receptive field; primarily rods) → dorsal stream P ganglion cells (smaller receptive field; primarily cones) → ventral stream Ventral stream: the “what” pathway (processing for shape, form) Dorsal stream: the “where/how” pathway (processing location in space) Also processing how to interact with something (planning actions) Color & the physics of light — ventral stream Color: determined by light reflected from a surface The most relevant aspects of this are: (1) wavelength of the light, (2) wavelength purity vs broadband composition White light: all wavelengths are present with equal contributions Light from many sources apporximates white light (the sun, many lightbulbs, etc). Conditions for color to occur: (1) a limited number of wavelengths are given off by a light source, (2) a surface reflects light of particular wavelengths → wavelengths that aren’t reflected are absorbed Psychological dimensions of light Hue: related to wavelength (but the relationship is not always direct) The vertical strip that lets you pick your color from the rainbow Brightness: determined by how much light is reflected (if more light reflected, a color will be brighter) Brightness is organized vertically on the color map Saturation: relates to the purity of the wavelengths of your light Colors with more white have mor either wavelengths included — they’re less saturated Saturation displayed horizontally on color chart Eg. pastels are less saturated than vibrant colors (pink is aless saturated version of red) Functions of color vision Fruit theory: suggests our color vision developed as a way to more easily detect ripe fruit on plants (origin of color vision) Object recognition and identification: colors help us recognize objects and identify them in space → it’s easier to recognize objects if tey are the color we expect Phenomena in color & color vision: color mixing, contrast, color intuitions, color blindness, color cancellation Color mixing: light and pigments mix colors in different ways Lights do additive color mixing (all colors combine to make white) ○ Additive color mixing: when you add lights with different wavelengths you’re getting closer and closer to having all the wavelengths present Pigments do subtractive color mixing (all colors combine to make black) ○ Pigments absorb light → the color of the pigment is the color they reflect ○ When you combine pigments, more and more wavelengths are being absorbed (instrad of reflected) → you are subtracting certain wavelengths that used to be reflected when you combine two pigments ○ Only wavelengths that are reflected by both will be seen Color contrast: (1) simultaneous and (2) successive Simultaneous color contrast: a phenomenon where the same color is perceived to be different based on them having a different background color Demonstrates the colors are interpreted based on more than just wavelength → the color context they are in plays a role in our interpretation of color Successive color contrast (diplayed via color afterimages): when you look at a picture for a long time and then it is removed, you’ll often see the inversion of the colors Color intuition: specific combinations of colors feel intuitive to us, where others does (eg. combining red and green lights makes yellow, a color that feels like a mixture of red and green) How color vision works → rods Why rods are not good at seeing color: rods are sensitive to scotopic light levels Scotopic: dim light levels at or below the level of bright moonlight All rods contain the same type of photopigment molecule rhodopsin → all rods have the same sensitivity to different wavelengths — there is only one “type” of rod Cones have three varieties that allow them to see color: S-cones, M-cones, and L-cones S-cones: short wavelength cones sensitive to short wavelengths (eg. light in the blue end of the color spectrum) M-cones: medium wavelength cones sensitive to middle wavelengths (eg. light in the green part of the color spectrum) L-cones: long wavelength cones sensitive to long wavelengths of light (eg. light in the red end of the spectrum) Photoreceptor response: photoreceptors fire more strongly when: (1) the wavelength of light is closer to their preferred wavelength, (2) there is more being absorbed by the photoreceptor/it is brighter For cone pigments, absorption spectra are all a little different and peak at different points/each cone will respond most strongly to a different wavelength It is necessary to have 3 cones because of the problem of univariance The problem of univariance: two dimensions need to be represented: (1) intensity and (2) wavelength, but the cell only has one means of reporting information: the firing rate ○ Formal definition: you have an infinite set of wavelength intensity combinations that can elicit the same response from a single type of photoreceptor → one type of photoreceptor cannot allow you to discriminate color on its own ○ Problem 1: if one cell is responding at about half of its maximum response, there will be two colors it corresponds to, so you can’t tell from the response alone ○ Problem 2: brighter light causes a stronger response from cells, so it is not clear if the percent absorbed is from the more favorable wavelength or a stronger light at a less favorable wavelength ○ How the problem is fixed: is fixed if we have two cells with different preferred wavelengths and, therefore at different firing rates (can use this info from BOTH receptors to account for wavelength and intensity) Trichromacy: with three cone types, we can tell the difference between lights of many different wavelengths Firing patterns of all 3 cone types allow us to neurally code for color 2 is sufficient, but 3 allows us to make more distinctions (dogs have 2) However, this does not make us wavelength detectors → any two stimuli where the output of one color would match the output of other will give us the same color experience Why we fail as wavelength detectors: (1) metamers and (2) nonspectal hues Metamers: stimuli that are physically different, but perceptually identical ○ Eg. even though the image below has two sets of wavelengths, they will look the exact same Nonspectal hues: colors that we perceive but don’t correspond with a particular wavelength (eg. magenta is just a strong activation of L and S cones/blue and red light, but these are on opposite sides of the visible light spectrum) Color blindness: vision without trichromacy (they have two cones) Red-green color deficiencies are the most common: protanopia = people can’t see red (lack L cone); deuteranopia = people can’t see green (lack M cone) Yellow-blue color blindness: tritanopia = people can’t see blue (lack S cone) Theories of color vision Trichromatic theory (Young-Helmholtz theory): color vision is supported by 3 different primary color sensations (red, green, and blue) → maps neatly onto the physiological discovery of cones Supporting experiment: color matching — participants are given a test region that has light of one specific wavelength ○ Participant adjusts light on a comparison region, with a goal of making the color of the region match the test region ○ Results: participants needed three different wavelengths to make the colors march, and the wavelengths needed to be independent of each other (ie. the other two could not match it on their own) Main idea → all of our color experience comes from a combination of these colors (eg. yellow is a mixture of red and green) Drawbacks of trichromatic color theory: does not explain color intuitions, successive contrast (color afterimages), and colorblindess phenomena (1) Color Intuitions: ○ Some colors, like yellow, feel like fundamental, pure colors to us. Yellow doesn’t seem like a mixture of red and green, even though the trichromatic theory suggests it is. ○ It’s difficult to imagine certain color combinations, like a “reddish-green,” while it’s easier to picture “reddish-yellow.” This suggests the theory doesn’t fully explain how we perceive colors intuitively. (2) Successive contrast (color afterimages): ○ When you stare at a red spot for a while, the L-cone (which detects long wavelengths like red) gets fatigued. ○ Once you look away, your visual system compensates by boosting signals from other cones, like those for blue and green, leading you to expect a cyan afterimage. ○ However, most people report seeing a green afterimage, which doesn’t align with what the trichromatic theory would predict. (3) Colorblindess ○ The most common type of colorblindness is red-green color blindness, which occurs due to issues with either the L-cone (long wavelengths) or the M-cone (medium wavelengths). ○ But if you have a problem with one of these cones, you struggle to distinguish both red and green colors, which suggests these colors come in pairs. ○ This paired difficulty isn’t easily explained by trichromatic theory, which focuses on the three types of cones individually rather than pairing colors. Opponent-process theory: color vision is the product of the output of 3 different opponent systems (red-green, blue-yellow, black-white) 4 primary color sensations (red, green, yellow, blue), organized into opponent pairs (red-green, blue-yellow) Perceiving one of the color pairs will inhibit the perception of the other (eg. red light will inhibit activation by green light from a certain point of space; same thing for yellow-blue pair) How OPT addresses problems with trichromatic color theory: Intuition #1: problem with trichromatic theory is that yellow doesn’t feel like a primary color ○ Solution: yellow is a primary color that we oppose with blue Intuition #2: red-green as a combo is hard to imagine ○ Solution: in OPT, these colors oppose one another, so you can’t perceive both as a combination Successive contrast: problem with trichrome theory is that we see green instead of cyan from from the afterimage from red light ○ Solution: the red-green opponency detector will be very excited by red light. When the red light is removed, it is fatigued and does not fire as much. To the cell, this looks like inhibition from from green since they are opponent pairs → so we will see a green afterimage Colorblindness: trichrome does not explain why red-green color blindness is the most common ○ Solution: in OPT, color is coded as opposing perception of red/green or yellow/blue. So if you remove the ability to receive red, green is also knocked out bc it can’t be contrasted with the opponent process Hue cancellation: process in opponent-process theory where one color (hue) is neutralized or "canceled out" by its opposing color, such as canceling out yellow with blue or red with green How the OPT theory gained traction Famous experiments by Hurvich & Jameson (1957): participants given a unique hue and asked to use the hue to cancel the opposing color in the blue-green patch. They use red to cancel the green. Unique hues are wavelengths of light that are “pure” version of the colors → so the primary colors in OPT are the unique hues in blue, green, yellow, and red Cancelling one color with another does indicate that opponent processes may be occurring because you can only cancel a color with their opponent color, supporting the existence of opponent pairs Physiological evidence for OPT: opponent process neurons were discovered in primate LGN in parvocellular layers Input from 2+ cones go to each cell, and operate in red-green or blue-yellow opponency pairs Combining trichromatic & opponent process theories: both of these theories are included in neural models → we have 3 photoreceptors, so trichromacy has to be at play, but we need them to organize into 4 primary colors for OPT to also exist Retinal layer should capture trichromatic theory, next layers may include opponent process theory Red-green opponency: green photoreceptors excite the opponent neuron; red photoreceptors inhibit this neuron Blue-yellow opponency: L+M cones firing together on an interneuron code for yellow. The interneuron inhibits the blue-yellow neuron when yellow light is present, but the S-cones excite the blue-yellow neuron if blue light is present Cerebral achromatopsia: color blindness that occurs due to damage to the cortex, meaning that we have a color area that can be damaged, but neurophysiological recordings don’t support this. Therefore, color vision appears to be a process that is distributed across many parts of the visual stream Color perception Unrelated colors: colors experienced in isolation with no environmental context Related colors: color seen in relationship to another color in physical space, which often changes our interpretation of the color Color constancy: understanding colors in natural scenes usually requires that we be able to perceive that objects as having the same color even when their lighting conditions (and reflectance) is different Why the visual system codes for contrast — helps us maintain constancy Physical constraints help us with this: guesses about the illuminant, assumptions about the light sources/surfaces These mechanisms also involved in: chromatic adaptation + memory color Chromatic adaptation: visual system’s ability to predict lighting conditions and adjust its sensitivity accordingly, so we can keep a sense of color under different lightings One of the mechanisms involved in color constancy Lecture 8: Object Perception Objects Object: basic units in our representations of the world Object perception: the process by which we abstain descriptions of shape, size, and material composition → don’t necessarily fully know what the object is yet Segmenting objects for humans often includes knowledge of an object/info about how the physical world actually works Allows us to understand this segmentation from a distance/being able to put together complex scenes Efficiency of object perception: humans perceive objects relatively quickly (80-100 ms) Obstacles to object perception: (1) segmentation, (2) grouping/unit formation, (3) 3D representations from certain angles, (4) describing and representing shape Segmentation: how is the visible world broken into separate objects? Grouping/unit information: how do separate visual regions get connected into objects? Object recognition: the process of matching the description obtained from perception with something stored in memory Basic-level recognition: recognition of an object category Instance recognition: eg. “this is my favorite armchair, this is my nephew’s ball” Edge detection Edges: regions where contrast (color, brightness, spatial frequency, etc.) exist going in a particula orientation Detecting edges helps identify where objects may end, and where another may begin Given by differences in: ○ Luminance ○ Color ○ Motion ○ Depth ○ Texture (different surface properties) Edges are not always clear: edges may be impossible to detect if they are: low/no contrast, blurry/not sharp, behind another object → but this doesn’t mean they don’t exist, the brain just needs to fill in the gaps Missing edges Spurious edges: edges that emerge from noise, and are not neccesarily real edges Challenges to edge detection: (1) integrating local edges into global contours, (2) distinguishing different kinds of edges (object boundary, surface markings, shadows, textures) Junction detection/edge intersection: when two edges intersect, you have a junction Junctions provide information about how edges are intersecting in space Junctions influence how we segment and group the scenes T junctions: when one object partially blocks another (occluding edges) X junction: occlusion with a transparent object L junction: edges that make corners Y junction: indicate a 3D object corner Boundary detection: what happens when you put edges together, helps us determine which edges mark the outside edges of an object We use these to separate objects from other objects and their background Form and object perception: the process helps us to detect form and put parts together to form an object → this needs to happen in cases where sections of an object are separate Segmenting and grouping: processes by which we decide what goes together in a visual scene (segmet objects that are separate, group objects that go together) → classical approach are the Gestalt principles Gestalt Theory Gestalt psychology: “the whole is greater than the sum of its parts” Emerged in the late 1800s as an approach to studying psychology generally Humans organize visual information into meaningful whole, which adds information not available from the parts that built the representation Properties of Gestalt theory: (1) reification, (2) multistability, (3) invariance Reification: the perceived object has more spatial information than the sensory stimulus on which it is based Multistability: ambiguous stimuli can have reversible interpretations Invariance: we can recognize objects regardless of their orientation, position, etc. Gestalt grouping principles: Gestalt ways applied to segmentation and grouping (1) Good continuation/law of good continuity: objects within an object should be grouped together (a) Objects tend to be connected with smooth curves/abrupt changes are less likely to be connected with one another (2) Similarity: objects that are similar are grouped together (similar = color,shape,texture,size) (3) Proximity: objects that are close in space to one another are more likely to be grouped together (4) Common fate: things that move together are often group together (we perceive motion as being on the smoothest path) (5) Closure: closure occurs when we perceive a connection or continuity between elements which do not touch each other (why we complete figured even when part of the information is missing) (6) Symmetry: symmetrical objects are perceived collectively (7) Simplicity/good form: explanations for shape consist of simple objects are preferred over explanations that consist of compex objects (8) Figure-Ground relationship: seeking to separate figures from those behind them