PSP Diagnostics Compendium Part II (PDF)
Document Details
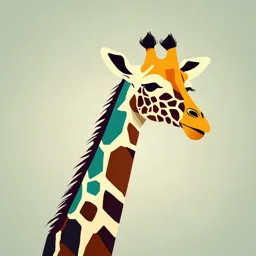
Uploaded by BestSellingBeige
null
Tags
Summary
This document is a training compendium for premium service providers, focusing on transformer diagnostics. It details dielectric properties, dissipation factors, and measurement techniques.
Full Transcript
Training Compendium Premium Service Provider Diagnostics Part II Part II Version 01,17.03.2020 17:07:09 2/101 Part II Version 01 PSP Diagnostics Compendium Part II_V01_EN.docx Table of contents Table of contents.........................
Training Compendium Premium Service Provider Diagnostics Part II Part II Version 01,17.03.2020 17:07:09 2/101 Part II Version 01 PSP Diagnostics Compendium Part II_V01_EN.docx Table of contents Table of contents................................................................................................................................... 2 List of figures......................................................................................................................................... 4 List of tables.......................................................................................................................................... 7 Formula.................................................................................................................................................. 8 1 Introduction.............................................................................................................................. 10 2 Basics for transformer measurements.................................................................................... 11 2.1 General.................................................................................................................................... 11 2.2 Dielectric properties of insulating material – the dissipation factor......................................... 12 2.2.1 Dissipation factor: definition of the term.................................................................................. 13 2.2.2 Dissipation factor: ideal component and ideal material........................................................... 13 2.2.3 Dissipation factor: real component and real material.............................................................. 14 2.2.4 Dissipation factor: typical values for transformers and bushings............................................ 15 2.2.5 Dissipation factor: influence of frequency and temperature.................................................... 15 2.2.6 Short circuit and potential connection of capacities................................................................ 16 2.2.7 Negative dissipation factor...................................................................................................... 18 2.2.8 Test methods UST and GST................................................................................................... 19 2.3 Polarization.............................................................................................................................. 21 2.4 Interference of test signal........................................................................................................ 24 2.5 Guarding.................................................................................................................................. 27 2.5.1 Definition of terms................................................................................................................... 27 2.5.2 Measuring principle................................................................................................................. 27 2.6 Device grounding.................................................................................................................... 29 2.6.1 General.................................................................................................................................... 29 2.6.2 Reduction of total resistance of ground loop........................................................................... 29 3 Dissipation factor and capacitance measurement on the transformer.................................... 32 3.1 General.................................................................................................................................... 32 3.2 Definition of terms................................................................................................................... 32 3.3 Components............................................................................................................................ 33 3.4 Influencing factors................................................................................................................... 33 3.5 Measuring principle................................................................................................................. 33 3.6 Evaluation................................................................................................................................ 34 4 Dissipation factor and capacitance measurement on bushings.............................................. 37 4.1 General.................................................................................................................................... 37 4.2 Definition of terms................................................................................................................... 41 4.3 Components............................................................................................................................ 42 4.4 Influencing factors................................................................................................................... 44 4.5 Measuring principle................................................................................................................. 48 4.6 Evaluation................................................................................................................................ 48 4.7 Hot collar................................................................................................................................. 53 5 Moisture in paper – dielectric frequency response with FDS and PDC.................................. 54 3/101 Part II Version 01 PSP Diagnostics Compendium Part II_V01_EN.docx 5.1 Definition of terms................................................................................................................... 54 5.2 Components............................................................................................................................ 54 5.3 Influencing factors................................................................................................................... 56 5.3.1 Measuring principle................................................................................................................. 58 5.3.2 Test setup................................................................................................................................ 60 5.3.3 Short circuit............................................................................................................................. 62 5.4 Measuring result...................................................................................................................... 63 5.5 Measures for improving the test signals................................................................................. 66 6 Magnetizing current................................................................................................................. 70 6.1 Definition of terms................................................................................................................... 70 6.2 Components............................................................................................................................ 71 6.3 Influencing factors................................................................................................................... 72 6.4 Measuring principle................................................................................................................. 73 6.5 Evaluation................................................................................................................................ 73 7 Vibro-acoustic measurement.................................................................................................. 77 7.1 General.................................................................................................................................... 77 7.2 Definition of terms................................................................................................................... 77 7.3 Measuring principle................................................................................................................. 78 7.4 Components............................................................................................................................ 79 7.5 Influencing factors................................................................................................................... 80 7.6 Performance of the measurement........................................................................................... 82 7.6.1 Diverter switch operation with diverter switch and tap selector.............................................. 82 7.6.2 Diverter switch operation with selector switch........................................................................ 83 7.6.3 Potential connection sounds................................................................................................... 83 7.6.4 Multi-column application.......................................................................................................... 84 7.7 Documentation........................................................................................................................ 85 7.8 Evaluation................................................................................................................................ 86 8 Frequency response of transformer – FRA............................................................................. 88 8.1 General.................................................................................................................................... 88 8.2 Definition of terms................................................................................................................... 90 8.3 Components............................................................................................................................ 90 8.4 Influencing factors................................................................................................................... 92 8.5 Measuring principle................................................................................................................. 93 8.6 Steps to perform...................................................................................................................... 94 8.7 Evaluation................................................................................................................................ 98 A Attachments.......................................................................................................................... 100 Bushing: Template for temperature documentation.............................................................. 100 Factors, powers and names.................................................................................................. 101 4/101 Part II Version 01 PSP Diagnostics Compendium Part II_V01_EN.docx List of figures Figure 1 – Ideal and loss-free dielectric................................................................................................ 13 Figure 2 – Substitute diagram of an ideal insulator............................................................................... 14 Figure 3 – Vector diagram of an ideal insulator..................................................................................... 14 Figure 4 – Substitute diagram of the real insulator, parallel connection............................................... 14 Figure 5 – Vector diagram of real insulator........................................................................................... 14 Figure 6 – Two-winding transformer: shorted capacitances of the secondary and grounded winding. 17 Figure 7 – Two-winding transformer: Switch matrix for UST-A measurement...................................... 19 Figure 8 – Plate capacitor: free electrons in conductive material and resulting electric field in dielectric............................................................................................................................................................... 21 Figure 9 – Plate capacitor: free electrons in dielectric.......................................................................... 21 Figure 10 – Plate capacitor: aligned electrons...................................................................................... 22 Figure 11 – Polarization: Schematic overview of types of polarization and time constant................... 23 Figure 12 – Schematic representation of conductor, shield and field lines........................................... 25 Figure 13 – Three-conductor cable: Schematic design with conductor and two shields...................... 26 Figure 14 – Three-conductor cable: Connection to ground potential of inner shield on one side and of outer shield on two sides....................................................................................................................... 26 Figure 15 – Current of a simple arrangement of an insulation.............................................................. 27 Figure 16 – Current over simple arrangement of an insulation with guard........................................... 28 Figure 17 – Schematic ground loop resistance between transformer and test equipment, dotted....... 30 Figure 18 – Schematic overview ground loop resistance of transformer and test equipment with increased lead cross-section based on parallel conductors.................................................................. 31 Figure 19 – Three-winding transformer: names of all capacitances..................................................... 34 Figure 20 – Dissipation factor on the transformer: Voltage run for capacitances and dissipation factors of a three-winding transformer, FFAC15118......................................................................................... 35 Figure 21 – Dissipation factor on the transformer: Frequency run for capacitances and dissipation factors of a three-winding transformer, FFAC15118......................................................................................... 36 Figure 22 – Bushing: Typical test tap (left), American version (right)................................................... 37 Figure 23 – Bushing: insulation installed later on.................................................................................. 38 Figure 24 – Bushing: cross section of middle tube, paper insulation and condenser layers................ 39 Figure 25 – Bushing: Schematic overview of condenser coatings and insulation material.................. 40 Figure 26 – Bushing: Setup with test tap and condenser body............................................................. 41 Figure 27 – Bushings: Overview manufacturing technologies and distribution in the market............... 42 Figure 28 – Bushing: Test setup with HV cable and potential connection using spiral cable............... 45 Figure 29 – Bushing: Test setup with HV cable leads to measurement errors due to partial discharges............................................................................................................................................................... 46 Figure 30 – High-voltage bushing: Nameplate...................................................................................... 48 Figure 31 – Bushing: Frequency run for capacitances and negative dissipation factors, MR185904.. 49 Figure 32 – OIP bushing: dissipation factor frequency run with moisture in bushing of phase V, deviating measurement for neutral conductor; MR083167................................................................................... 50 Figure 33 – OIP bushing: dissipation factor voltage run; MR083167.................................................... 50 5/101 Part II Version 01 PSP Diagnostics Compendium Part II_V01_EN.docx Figure 34 – Bushing: Note very low values of dissipation-factor measurement for bushing N up to 50 Hz, MR1014185........................................................................................................................................... 51 Figure 35 – Bushing: Voltage run on RIP bushings with irregular test contact (red) and regular test contact (blue)......................................................................................................................................... 51 Figure 36 – Bushing: Frequency run and voltage run of capacitance with typical course for technologies OIP or RBP; FFAC000601.................................................................................................................... 52 Figure 37 – Hot collar: Overview of measurement of bushing without test tap..................................... 53 Figure 38 – Overview main insulation CHL with test circuit.................................................................... 55 Figure 39 – Dissipation factor over frequency – influence of various parameters................................ 57 Figure 40 – Test setup dielectric frequency response.......................................................................... 58 Figure 41 – Charging and discharging of a capacitor and polarization / depolarization assignment.... 60 Figure 42 – Shield potential connection of test cable on Kelvin clamp: red circle guard connection, blue circle: short-circuit bridge to the other bushings.................................................................................... 61 Figure 43 – Shield potential connection to transformer: a) with Kelvin clamp, b) with screw terminal. 62 Figure 44 – Example of an FDS measurement using IDAX 300 and software IDAX 5.0..................... 63 Figure 45 – Dielectric frequency response using OMICRON DIRANA and PTM 4.5........................... 64 Figure 46 – Dissipation factor for dielectric frequency response with interferences............................. 64 Figure 47 – Currents of different dielectric response measurements with OMICRON DIRANA, current in nA and time in seconds on x axis.......................................................................................................... 65 Figure 48 – Disturbed dielectric frequency response with OMICRON DIRANA, current in nA on y axis and time in seconds on x axis............................................................................................................... 65 Figure 49 – HV power lines with electromagnetic waves that can lead to electrical interferences....... 66 Figure 50 – Disturbed signal improved by input in highest bushing...................................................... 67 Figure 51 – Two-conductor cable: Example of shielded test cable, left to right: jacket, shield net and foil, dielectric, soul........................................................................................................................................ 68 Figure 52 – On the left, OMICRON DIRANA front with GUARD bushing; on the right: Kelvin clamp with connection box for three-conductor cable and guard over 4-mm bushing and lab plug (hidden in the picture)................................................................................................................................................... 68 Figure 53 – Extension of cross-section for guard connection between test equipment and Kelvin clamp............................................................................................................................................................... 69 Figure 54 – Substitute diagram, neglecting the secondary-side winding impedance........................... 71 Figure 55 – Substitute diagram, single-phase, in no-load operation..................................................... 71 Figure 56 – No-load test current: presentation in typical, frequently used bar chart............................. 74 Figure 57 – No-load test current: presentation of eddy iron losses in typical, frequently used bar chart............................................................................................................................................................... 74 Figure 58 – Diagram of transformer turns ratio..................................................................................... 75 Figure 59 – No-load test current I0m at 10 kV test voltage................................................................... 75 Figure 60 – Vibro-acoustic measurement: Example of a specimen with excited transformer during change-over selector operation, with motor sounds............................................................................. 79 Figure 61 – Vibro-acoustics: Frequency-time presentation in the upper half and envelope graph for frequency band 10-100 kHz in the lower half of the picture; bad signal to noise relation..................... 81 6/101 Part II Version 01 PSP Diagnostics Compendium Part II_V01_EN.docx Figure 62 – Vibro-acoustics: Frequency-time presentation in upper half and envelope graph for frequency band 10-100 kHz in lower half of picture; good signal to noise ratio.................................... 81 Figure 63 - Vibro-acoustics: Frequency-time presentation of diverter switch operation and arc of a change-over selector operation with arc............................................................................................... 83 Figure 64 – Vibro-acoustics: Voltage signal of a complete on-load tap-changer operation without tap selector or change-over selector........................................................................................................... 86 Figure 65 – Vibro-acoustic measurement: Frequency-time presentation of energized transformer with drive shaft noise.................................................................................................................................... 87 Figure 66 – Vibro-acoustic measurement: Envelope graph of frequency band 10 kHz -100 kHz with very good distance between peak at 10 dB and sound at < -40 dB............................................................. 87 Figure 67 – RLC network overview....................................................................................................... 88 Figure 68 – Frequency response of the transformer: Schematic presentation of the test method frequency response on the transformer with Bode diagram for amplitude and phase......................... 89 Figure 69 – Frequency response of transformer: Dampening in decibel, top part, and phase shift in degrees, see below............................................................................................................................... 89 Figure 70 – Frequency response of transformer: Dampening and influence of components on typical frequency bands.................................................................................................................................... 91 Figure 71 – Frequency response of transformer: Positive and negative example of grounding strap.. 92 Figure 72 – Frequency response of transformer: Terminal with coaxial cables for input and reference............................................................................................................................................................... 93 Figure 73 – Frequency response of transformer: Terminal with coaxial cable for measurement......... 94 Figure 74 – Frequency response of transformer: Grounding straps on bushing base.......................... 95 Figure 75 – Frequency response of transformer: Terminals with coaxial cables and strain relief on bushing pin............................................................................................................................................ 95 Figure 76 – Frequency response of transformer: BNC adapters for coax cables................................. 96 Figure 77 – Frequency response of transformer: Example of connection of all three cables on bushing for zero measurement........................................................................................................................... 96 Figure 78 – Frequency response of transformer: Documentation of test setup and check of test equipment and cables using zero measurement.................................................................................. 97 Figure 79 – Frequency response of transformer: Example for pictures and assignment..................... 97 Figure 80 – Frequency response of transformer: Auto transformer with complete regulating and parallel winding................................................................................................................................................... 98 Figure 81 – Frequency response of transformer: Auto transformer with all winding turns................... 99 7/101 Part II Version 01 PSP Diagnostics Compendium Part II_V01_EN.docx List of tables Table 1 – Substitute diagram, vector diagram and dissipation factor of the ideal capacitor................. 14 Table 2 – Substitute diagram, vector diagram and dissipation factor of the real capacitor.................. 14 Table 3 – UST measurements............................................................................................................... 20 Table 4 – GST measurements.............................................................................................................. 20 Table 5 – Dissipation factors: Typical values and limit values for bushings at rated frequency and 20 °C............................................................................................................................................................... 52 Table 6 – Bushing: Limit values for deviation of measured to original capacitance at 20 °C and rated frequency............................................................................................................................................... 52 Table 7 – Frequency and cycle duration of a sinusoidal wave.............................................................. 59 Table 8 – Bushing: Template for documentation of temperatures during measurement.................... 100 8/101 Part II Version 01 PSP Diagnostics Compendium Part II_V01_EN.docx Formula C̅1 Capacitance of all condenser layers of condenser bushings C̅2 Capacitance of condenser bushings between test tap and ground potential C̅HL Capacitance between primary and secondary winding (H for High and L for Low) C̅H Capacitance between primary winding and ground potential (H for High) C̅L Capacitance between secondary winding and ground potential (L for Low) ϵr relative permittivity Hi electric field strength in the conductor of a cable HO electric field strength in the shield of a cable 𝐼0̅ No-load current at rated voltage ̅ 𝐼0m No-load test current, no-load current at measured voltage IC̅ capacitive reactive current, current through purely capacitive consumer / component ̅ 𝐼CH Current of capacitance between primary winding and ground potential (H for High) ̅ 𝐼CHL Current of capacitance between primary and secondary winding (H for High and L for Low) ̅ 𝐼CL Current of capacitance between secondary winding and ground potential (L for Low) ̅ IFE Iron loss current 𝐼m̅ Test current 𝐼µ̅ Magnetizing current 𝐼n̅ Rated current IR̅ Active current, current through purely ohmic consumer / component ̅q U Voltage of a voltage source ̅n U Rated voltage between two phases in three-phase transformer ̅ FE R Iron loss resistance 9/101 Part II Version 01 PSP Diagnostics Compendium Part II_V01_EN.docx 𝑋̅h Reactance of main inductance ≂ Minus Tilde, stands for AC and DC voltage source 10/101 Part II Version 01 PSP Diagnostics Compendium Part II_V01_EN.docx 1 Introduction The basics about the transformer that are described in Compendium Part I are essential prerequisite for the present Compendium Part II. We refer to the titles of the relevant chapters of Part I only occasionally. The described physical and electrical phenomena are presented in simplified terms, with the intention to achieve basic understanding. This presentation is not intended for a more in-depth examination. 11/101 Part II Version 01 PSP Diagnostics Compendium Part II_V01_EN.docx 2 Basics for transformer measurements 2.1 General The terms used in part II of the compendium are difficult to understand at the beginning. Therefore we will first give a brief summary of why and for what reason dissipation-factor measurements are performed. We are dealing with the power transformer part that insulates high voltages: oil and paper, so-called dielectrics. If dielectrics cannot insulate two potentials against each other anymore, flashovers will occur that usually mean the end of a transformer's life. The components that are to be isolated from one another and the dielectric between the components form a capacitor. This is an advantage for the measuring technician, because the capacitor can be measured. To be more specific, impedance of the capacitor is measured. Impedance represents an ideal and a real part of the capacitor. The ideal part is the loss-free capacitor that consists of capacitance only. The real part represents the losses in the capacitor. Basically, these losses are very low. The corresponding current is very small. Current is so small that it is necessary to equip the test cables with double shields. Sometimes this is not sufficient either, and additional measures must be taken, which means greater effort than, e.g., test setup for a winding resistance measurement at 6 A test current. Over the years, that is, when the dielectric ages, losses in the capacitor or dielectric of the transformer increase. In addition, there will be more and more water in the paper. On the molecular level, water consists of dipoles whose behavior depends on frequency. This is another fact that can be exploited by the measuring technician. At very low frequencies, the behavior of dipoles can be clearly differentiated from other molecules. Therefore interpretation of the dissipation factor was developed further, and the original method was enhanced and adjusted. Dissipation factors of dielectrics can be measured using high voltages, frequency sweep, rated frequency or very low frequencies. All of the methods have the same goal: interpretation of the results should allow to assess the condition of the dielectric in the transformer and bushings. The correlations presented in short form here will be discussed in detail in the following chapters. 12/101 Part II Version 01 PSP Diagnostics Compendium Part II_V01_EN.docx 2.2 Dielectric properties of insulating material – the dissipation factor Transformers utilize voltages and currents. This means that components of different potentials must be separated from each other or in other words, insulated against each other. Electrically insulating materials are used for this purpose. Insulating materials that are penetrated by electric fields are called dielectrics. The property to insulate electric fields more or less well is called permittivity. As an illustration, let us look at a simple application that is very common in electrical technology. When a plate capacitor made from two conductive, parallel plates and a dielectric that is placed in-between, the main job of the dielectric is to let the electric field pass as lossless as possible and at the same time prevent the charge carriers on the plate from penetrating through the dielectric. If electrically conductive molecules or molecules that can be changed by the electric field are present in the dielectric, the dielectric properties of the dielectric change; the keyword here is "polarization". Ohmic losses can occur, or, in the worst case, faults such as breakdowns. Losses of a dielectric are very much dependent on frequency and temperature. On the one hand, this means that temperature as test quantity must always be measured and recorded when testing in the field and on the other hand, that you can perform tests with different frequencies and identify the influence of disturbing molecules at specific frequencies. The majority of transformers that are tested by MR are oil-immersed transformers. Most of the times, mineral oils, cellulose, that is paper, and pressboard are used as insulation. Cellulose and pressboard also take on design and mechanical protection tasks and prevent direct contact of conductive components with different potentials when impact is exerted by external forces. Apart from its job to improve insulation, mineral oil also cools and greases the on-load tap-changer. Regarding application of dielectrics in the transformer, one can generally say that aging, moisture, gases and particles account for the largest amount of weakening of dielectric properties. Therefore insulations in transformers and bushings are very often tested for water content and electric conductivity. 13/101 Part II Version 01 PSP Diagnostics Compendium Part II_V01_EN.docx 2.2.1 Dissipation factor: definition of the term The dissipation factor - together with other parameters that will not be discussed here - was introduced to identify quality and aging of an insulator or dielectric. There are other terms that are used as synonyms and mean the same thing. When dealing with this compendium and with customers, the term dissipation factor is used. Dissipation factors for insulator and dielectric are described below. The insulator is a technical component that comprises a dielectric. The dielectric is to be understood as integral part of a component, only rarely as single material. 2.2.2 Dissipation factor: ideal component and ideal material If a dielectric is ideal, it insulates two conductors from each other without losses. The measured current of the capacitor in which the dielectric is arranged, is then purely capacitive, that is, current precedes voltage by 90 degrees. The elements in the substitute diagram are presented using AC voltage source and capacitor, see Figure 1. IC Uq ~ Im = IC A Figure 1 – Ideal and loss-free dielectric Impedance of the ideal insulator can be calculated as follows 1 (1) 𝑍̅ = R ∢ 0° + X ∢ − 90 ° = 𝑋̅𝐶 = ∢ − 90 ° 2π𝑓𝐶 Ohmic resistance is zero, and therefore the real portion of the current of an ideal insulator becomes zero. When the ohmic portion of the current becomes zero, the angle between capacitive current and ohmic current is zero, too. With this, the dissipation factor becomes zero. 14/101 Part II Version 01 PSP Diagnostics Compendium Part II_V01_EN.docx The related electrical substitute diagram, vector diagram and dissipation factor are presented in Table 1. Table 1 – Substitute diagram, vector diagram and dissipation factor of the ideal capacitor Vector diagram in the coordinate Dissipation Substitute diagram system factor IC IR̅ tan δ = = 0 IC̅ Im = IC Uq ≂ XC Im = IC Uq A Figure 2 – Substitute diagram of an ideal insulator Figure 3 – Vector diagram of an ideal insulator For ideal insulators and dielectrics, test current is identical to capacitive current since there are no losses, as described above. The angle between test current and capacitive current is zero and therefore dissipation factor tan δ is zero, too. 2.2.3 Dissipation factor: real component and real material When an insulator or dielectric is real, there are losses in the dielectric, for example from polarization, or losses on the insulators caused by surface currents over the shell or the component's outside. Impedance of the real insulator can be calculated as follows 1 (2) 𝑍̅ = R ∢ 0° + X ∢ − 90° = 𝑅∢ 0° + ∢ − 90° 2π𝑓𝐶 This means that active current is present on a real insulator; it stands for the losses of the insulator. This is illustrated in the substitute diagram in Figure 4. Table 2 – Substitute diagram, vector diagram and dissipation factor of the real capacitor Substitute diagram Vector diagram in the coordinate Dissipation system factor I IR̅ tan δ = > 0 IC̅ IR IC IR IC Uq ≂ R XC δ Im = IC + IR Im = IR + IC A Uq Figure 4 – Substitute diagram of the real insulator, parallel Figure 5 – Vector diagram of real insulator connection 15/101 Part II Version 01 PSP Diagnostics Compendium Part II_V01_EN.docx Losses over the surface of an insulator such as a bushing shed, are presented as parallel connection of ohmic resistance and capacitance. Losses within the insulator or dielectric are calculated as series connection of ohmic resistance and capacitance; but for reasons of simplicity, this will not be dealt with in this compendium. When evaluating an insulator or dielectric using the dissipation factor, current over the surface should not be recorded. This is discussed in section 2.2.8 in the context of test method selection and in section 2.5 in the context of Guarding. The vector diagram shown in Figure 5 applies for real components. Capacitive current IC̅ is an ideal current. This current does not flow but is introduced for reasons of illustration and calculation. This ideal, capacitive current IC̅ is, together with the ohmic portion IR̅ , the actually measured test current as sum of ohmic and capacitive current. If test current 𝐼m̅ is purely capacitive, the dissipation factor becomes zero. This would mean that a dielectric is perfect and ideal, that is, loss-free. Losses also depend on aging, therefore when comparing old and more recent measurements, aging of an insulation can be reconstructed and unsafe operating conditions can be identified. 2.2.4 Dissipation factor: typical values for transformers and bushings In new condition, the values of dissipation factors of insulators and dielectrics are close to zero. As values are so small, the dissipation factor is multiplied by 100 and then specified in percent. Typical test values range from 0.2 to 1 percent. Losses of transformer and bushing insulations are dependent on frequency and, to a large degree, on temperature. Therefore all of the manufacturer values are referenced to rated frequency and a typical common temperature of 20 °C. 2.2.5 Dissipation factor: influence of frequency and temperature All ohmic resistances in this section 2.2 depend on frequency and temperature. At low frequencies, surface water or moisture in the dielectric can still align its dipoles in accordance with polarity of the sine-shaped voltage in direction + or –. When increasing frequency, the dipole will align less and less, until it is not aligned at all to the sine of the input signal at high frequencies. This also implies that ohmic resistances get less and less. At the same time, temperature in the dielectric changes mobility of the molecules. This is obvious for frozen water. Water molecules move less at low temperatures, therefore dissipation factor at low temperatures is different than dissipation factor at high temperatures. This is very important for measurement and interpretation by means of comparative values. Therefore all manufacturer specifications refer to rated frequency and 20 °C. Temperature corrections using factors will continue to be used, though we are fully aware of the inaccuracy of these factors. 16/101 Part II Version 01 PSP Diagnostics Compendium Part II_V01_EN.docx 2.2.6 Short circuit and potential connection of capacities Measurements on site often require fast technical solutions that can be reached by changing connections on transformer or test equipment. In this context, let us discuss short-circuit of capacitances. When a capacitor or a capacitive arrangement, e.g., two windings, is loaded by a voltage source, this capacitive arrangement will change its load when the frequency of the voltage source changes. When the capacitance is short-circuited, the capacitor becomes operatively ineffective and on the other hand, the capacitor can self-discharge when the voltage source is switched off. The first point is important to avoid charge time of a capacitor where necessary, this is especially true for direct current. The last point contributes to safety at work. A further possibility during measurement is potential connection of a capacitor or capacitor-like arrangement. On complex test objects such as transformers, it may be necessary to connect components or arrangements to ground potential to avoid charging and discharging of the resulting capacitances. This is very often a short-circuit via the transformer grounding. This is illustrated in Figure 6, with the secondary winding connected to ground potential. Capacitive coupling and circulating currents can only develop if two adjacent parts have different electrical potentials and an electric field can build up between these potentials. If we put this into common language, we can say: If both sides of a setup that was first arranged like a capacitor have the same potential, the electrons do not need to move in any direction because the electrons are already in balance. If you notice unintentional charging caused by coupling capacitance during DC measurements or capacitive interferences especially during high-frequent measurements with AC voltages, connecting both sides of the capacitor-like setup to ground potential can rectify the problem. When measuring capacitive setups, always consider which potential is supplied to a component by short-circuiting or deliberate potential connection. In the above example of a two-winding transformer, see Figure 6, ground potential was applied to the secondary winding. This changes capacitance C̅H , since potential of the transformer tank now brings ground potential closer to the primary winding. For measurements of capacitance, it is therefore necessary to remove any connections from the transformer. This is especially true for capacitor banks of industrial transformers. 17/101 Part II Version 01 PSP Diagnostics Compendium Part II_V01_EN.docx primary winding secondary winding CHL CH CH CL Figure 6 – Two-winding transformer: shorted capacitances of the secondary and grounded winding 18/101 Part II Version 01 PSP Diagnostics Compendium Part II_V01_EN.docx 2.2.7 Negative dissipation factor As described in previous chapters, the dissipation factor of a real insulator or dielectric is greater than zero, that is, the dissipation factor assumes a positive value. Yet negative dissipation factors were occasionally measured during measurements of transformers and bushings. A negative dissipation factor would mean that charges or current would flow permanently from the capacitive setup. But since a dielectric does not emit electrons, i.e., is not a current source, this is not possible. Therefore it is important to understand that in such cases, the causes are inductive or electrical couplings or electromagnetic interferences, see section 2.4, or resonances from unfavorable ground loop impedances or test cable or ground connections, see section 2.6. These causes can often be eliminated using simple measures or means. 19/101 Part II Version 01 PSP Diagnostics Compendium Part II_V01_EN.docx 2.2.8 Test methods UST and GST Test equipment with several inputs and outputs and several measuring units can be equipped with switch matrices. The switch matrices are supposed to add or remove inputs for measurement. For example it may be necessary to test a capacitive current on only one channel, and bypass surface currents of a bushing around the measuring unit, via the second channel and by means of a so-called guard. The dissipation-factor measurement of a two-winding transformer is shown below as simple example. The switch matrix is presented in a simplified and basic way in the test equipment in Figure 7. The point is that the equipment itself can make the necessary connections depending on the respective task, and consequently measure the relevant single or common channels. In the example shown below, only the capacitive current of the secondary side is routed to the Ampere meter. Current over the bushing surface and the transformer tank (capacitive and ohmic) is bypassed around the measuring unit. promary winding ICHL + ICL+ ICH Source / Ausgang secondary winding ICHL Input / Eingang A ~ Uq ICL+ ICH Input / Eingang B A ICHL CHL CH CL ICH ICL Ground / Erdung Figure 7 – Two-winding transformer: Switch matrix for UST-A measurement The following terms were established to identify the different connections of the switch matrix unambiguously: 1. ungrounded specimen test UST, for ungrounded specimen or ungrounded test circuit, and 2. grounded specimen test GST, for grounded specimen or grounded test circuit. The terms and principles are explained below. In the group of ungrounded test circuits (UST), only ungrounded channels are measured. Either one or two test circuit(s) with ground potential connection are always bypassed around the measuring unit. Therefore three measurements - presented in Table 3 - are possible, that are named in accordance with the test circuits that are routed into the measuring unit. 20/101 Part II Version 01 PSP Diagnostics Compendium Part II_V01_EN.docx Table 3 – UST measurements Measurement Input A Input B Ground potential UST A measured bypassed bypassed UST B bypassed measured bypassed UST (A+B) measured measured bypassed In the group of grounded test circuits (GST), all grounded test circuits are measured. That is, up to three channels can be measured concurrently. Therefore four measurements - presented in Table 4 - are possible, that are named in accordance with the test circuits that bypass the measuring unit. Table 4 – GST measurements Measurement Input A Input B Ground potential GST measured measured measured GSTg A bypassed measured measured GSTg B measured bypassed measured GSTg (A+B) bypassed bypassed measured Transformers are often tested in the substation. In substations, strong electromagnetical stress often exists due to adjacent overhead and power lines. Therefore it is possible that capacitive and inductive couplings are integrated into the measurement via the transformer tank. A clear disturbance of the measurement or falsification of the results can arise from this. As the loop is bypassed around the measuring unit during UST measurements, this method is more stable and insensitive. Therefore UST measurement should be preferred to GST measurement whenever this is possible. 21/101 Part II Version 01 PSP Diagnostics Compendium Part II_V01_EN.docx 2.3 Polarization Basically, all materials have free electrons. Depending on number of free electrons or charge carriers, materials are referred to as conductive and non-conductive. If voltage is applied to electrically conductive material, an electric field is set up between parallel structures of different potentials. All free electrons of the conductive material align along the applied potential, see Figure 8. IC ++++++++++++++++++ Uq E - - - - - - - - - - - - - - - - - - Figure 8 – Plate capacitor: free electrons in conductive material and resulting electric field in dielectric If a real dielectric is present between parallel conductors, this dielectric also contains free electrons or charge carriers, see Figure 9. IC = 0 Uq = 0 + - + - - + - + Figure 9 – Plate capacitor: free electrons in dielectric When applying voltage, these free electrons in the dielectric align along the existing electric field, see Figure 10. The process of charge alignment is called polarization as the simplified arrangement represents two poles where positive and negative charges move. 22/101 Part II Version 01 PSP Diagnostics Compendium Part II_V01_EN.docx IC ++++++++++++++++++ - - - - Uq E + + + + - - - - - - - - - - - - - - - - - - Figure 10 – Plate capacitor: aligned electrons There are different types of polarization. They can be subdivided according to their character, which in turn depends largely on the specific dimensions. 1. Polarization within an atom is called electronic, 2. Polarization within a molecule is called atomic, 3. Polarization of entire molecules is called dipolar, and 4. Polarization of molecules along boundary surfaces of two dielectrics is called boundary surface polarization. The duration of the listed polarization types varies. If frequency of the applied electric field varies, rapidly completed operations are relevant with high frequencies, whereas the slower types are only relevant when lower frequencies are used, see Figure 11. 23/101 Part II Version 01 PSP Diagnostics Compendium Part II_V01_EN.docx IC = 0 IC electronic +++++ Uq = 0 ~ + E=0 Uq ~ + E(f = 1 000 000 000 000 000 Hz) - - - - - IC = 0 IC atomic +++++ + - - Uq = 0 ~ E=0 Uq ~ + + E(f = 1 000 000 000 000 Hz) - + - - - - -- IC = 0 IC dipole +++++ + - - - Uq = 0 ~ E=0 Uq ~ + + E(f = 1 00 000 000 Hz) - + - - - - - IC = 0 IC interfacial +++++ + - - + - - + + Uq = 0 ~ - + E=0 Uq ~ E(f = 0,001 Hz) - + - - + + - - - - - Figure 11 – Polarization: Schematic overview of types of polarization and time constant 24/101 Part II Version 01 PSP Diagnostics Compendium Part II_V01_EN.docx 2.4 Interference of test signal To achieve basic understanding, the physical phenomena and electrical components described in this chapter are presented in simplified form. This presentation is not intended for a more in-depth examination. Conductors, whether cables or wires, are susceptible to interferences from adjacent electric or magnetic fields. Put simply, there are two influences relevant for substations or industrial complexes: 1. AC power or overhead lines induce induction voltage in adjacent conductors. 2. In every conductor, capacitive influences add current in every adjacent conductor; this current again causes disturbing voltages. In accordance with the Faraday principle, a conductive surface such as metal foil or shield mesh can block a conductor from electric or magnetic fields, see Figure 12. The outer field with magnetic field strength HO hits the shield mesh, which then absorbs the field. That is, magnetic field strength inside the conductor Hi is very much smaller than in the shield. This correlation between inside and outside magnetic field strength is presented in Figure 7, using the mathematical expression Hi ≪ HO. 25/101 Part II Version 01 PSP Diagnostics Compendium Part II_V01_EN.docx Figure 12 – Schematic representation of conductor, shield and field lines Source: https://sekels-magnetic-shielding.eu/index.php?title=Magnetische_Abschirmungen; requested on 20190412 To discharge the voltages and currents developing in the shield, the shield potential can be connected to ground potential. Depending on type and character of interfering and test signal, both ends or one end of the shield are connected to ground potential. Capacitive and inductive interferences are present in substations and steel works. Where necessary and possible, a cable with one conductor and two shields is therefore used, see design of three- conductor cable in Figure 13 as an example. 26/101 Part II Version 01 PSP Diagnostics Compendium Part II_V01_EN.docx shields conductor insulation Figure 13 – Three-conductor cable: Schematic design with conductor and two shields From wdwd - Eigenes Werk, CC BY-SA 3.0, https://commons.wikimedia.org/w/index.php?curid=24154842; requested on 20190801 To be able to protect the test signal from any type of interference, one of the shields must be connected to ground potential on one side, the second shield must be connected to ground potential on two sides, see Figure 14. Figure 14 – Three-conductor cable: Connection to ground potential of inner shield on one side and of outer shield on two sides 27/101 Part II Version 01 PSP Diagnostics Compendium Part II_V01_EN.docx 2.5 Guarding 2.5.1 Definition of terms The term "guard" refers to the English verb "to guard", meaning to protect or the English noun "guard" for protector. In measurement technology, it describes bypassing of currents around the measuring unit, in order that the test signal can be measured and recorded without errors. A guard belt made of flexible, semi-conductive material is used for bushing measurements. This shell is attached to the outer shell of the bushing. Please see chapter 4 for details. The term "guard" is also used when you are planning to connect a shield of the three-conductive cable to ground potential. In PDC / FDS measurement, there is one connection on the test equipment and one on the terminal box on the Kelvin clamp, which are called guard bushings. Please see chapter 5 for details. 2.5.2 Measuring principle Looking at real insulation, see Figure 15, capacitive current will develop when applying voltage to the two insulated conductive and parallel materials. Another current flows through particles in dirt or moisture over the surface of the real insulator. For reasons of simplicity and clarity, losses in the dielectric are neglected here. Therefore test current in Figure 16 is approximatively the sum of surface current IR̅ and capacitive current IC̅. Ic + IR Uq ≂ IR IR + IC A Figure 15 – Current of a simple arrangement of an insulation Currents can be bypassed around the measuring unit via the guard. To do so, the guard is connected to the surface of the insulator, that is connected to ground. For the arrangement of two conductive and parallel materials, a test circuit in accordance with Figure 16 can be assumed. 28/101 Part II Version 01 PSP Diagnostics Compendium Part II_V01_EN.docx IC + IR Uq ≂ IR IR A IC Figure 16 – Current over simple arrangement of an insulation with guard The arrangements shown in this section are supposed to clarify the "guard" principle. The same principle applies to more complex geometrical arrangements such as bushings or transformers. The currents bypassed around the measuring unit can be capacitive or ohmic; they will be presented in more detail in the respective section. Surface currents depend on frequency. Consequently, the ohmic portion, that is, loss current, changes with frequency of the test current. If moisture on the insulation fins of a bushing leads to changed graphs, this change is also dependent on frequency of the test voltage. This must always been taken into consideration for the plausibility check. 29/101 Part II Version 01 PSP Diagnostics Compendium Part II_V01_EN.docx 2.6 Device grounding 2.6.1 General Device grounding refers to the electrical connection of a conductive housing, component or device to ground potential. When a fault or failure occurs, touch voltage of a device should be max. 50 AC voltage for reasons of personal protection. In addition, fault currents should be diverted in order to protect components. Furthermore, parasitic and surface currents are diverted to ground. For the transformer test, the ground point - that is, the point where the terminal of the ground cable is connected to ground potential - should be identical with the ground point of the transformer. Basically, transformer test equipment is equipped with safety plugs. Whether this ground potential is connected and used in the test equipment, cannot be judged from the exterior. 2.6.2 Reduction of total resistance of ground loop Basically, the algebraic sign of a dissipation factor of a dielectric is positive. The dissipation factor for real insulations must always be positive. But it occurs occasionally that negative dissipation factors are measured by the test equipment. In those cases, the ohmic portion of the test circuit is too high. An important factor for improvement is to reduce ground loop resistance. Ground loop means the portion of the test circuit that is connected via ground cables, potential connections and substation grounding. In Figure 17, the ground loop is the dotted line as connection between transformer tank and test equipment housing. 30/101 Part II Version 01 PSP Diagnostics Compendium Part II_V01_EN.docx Uq primary winding ICHL + ICL+ ICH ~ ICHL + ICL+ ICH secondary winding ICHL A CHL CH CL ICH ICL Figure 17 – Schematic ground loop resistance between transformer and test equipment, dotted There are several factors to change the test result; please see detailed description below. 2.6.2.1 Increasing cross-sectional area of conductor Increasing the cross-sectional area between test equipment and test object improves ground loop resistance considerably. It is best to use a low-ohmic and low-inductive ground lead for this. A conventional cable / lead can also be used. Parallel to the existing ground connector, the additional conductor is connected between ground point of transformer and ground point of test equipment, in accordance with Figure 18. 31/101 Part II Version 01 PSP Diagnostics Compendium Part II_V01_EN.docx primary winding secondary winding CHL test equipment CH CL Figure 18 – Schematic overview ground loop resistance of transformer and test equipment with increased lead cross-section based on parallel conductors 2.6.2.2 Contact-circuit resistance on ground point Reducing contact-circuit resistance leads to a major improvement of ground loop resistance. Moisture, grease, foreign layers and varnish must be removed before the test, or during troubleshooting at the latest. 2.6.2.3 Equipotential bonding of test equipment on bushing base If the dissipation factor of a bushing is still negative after performing all the measures, equipotential bonding of the test equipment can be directly connected to the bushing flange. This will reduce the number of potential leads in the ground loop and can therefore reduce the influence of bad contact transitions from cable terminals and potential leads to the ground loop. When swapping the test setup to an additional or different bushing, it is also important to attach the equipotential bonding terminal of the device to the other bushing. 32/101 Part II Version 01 PSP Diagnostics Compendium Part II_V01_EN.docx 3 Dissipation factor and capacitance measurement on the transformer 3.1 General In the following, the term dissipation-factor measurement will be used as synonym for capacitance and tan δ or Tangens Delta. Measurement of dissipation factor and HV capacitances has been used for decades to determine the condition of various equipment such as medium-voltage cables, rotating machines and bushings. For transformers, the measurement was originally introduced to asses paper insulation, using 10 kV or 12 kV test voltage. For values of 10 kV and more and 15 to 400 Hz, it is the oil distance that is decisive for the dissipation factor. Therefore dissipation-factor measurement of transformers is only suitable to a limited extent for interpreting paper insulation. Capacitance values are sometimes interesting for transformer manufacturers. They are of little use for diagnosing transformers. Dielectric frequency response, described in chapter 5, has become a superior system to assess moisture in transformer insulation. When dissipation-factor measurement on the transformer is requested by the customer, there are often other reasons apart from technical ones. Using this unhelpful measurement to determine transformer condition is often induced by insurance companies, internal regulations or lack of knowledge. The basics described in chapter 2 are prerequisite for understanding the following relationships. 3.2 Definition of terms The statements presented in 2.2.1 are valid. Diagnosis using dissipation-factor measurement on the transformer is a voltage and frequency run. In most cases, sine-wave 50-Hz voltage in steps of 2 kV up to 12 kV and typical frequencies of 15 Hz, 20 Hz, 35 Hz, 50 Hz, 135 Hz, 220 Hz, 305 Hz, 400 Hz are used for measurement. That is, when talking about dissipation-factor measurement on a transformer, this refers to a multitude of test points that result in graphs showing an expected course. In addition, it is a comparative measurement. As dissipation- factor measurement is not a routine measurement at the transformer manufacturer's site, it is necessary to perform a footprint measurement in the field, comparing later measurements with this first measurement. 33/101 Part II Version 01 PSP Diagnostics Compendium Part II_V01_EN.docx 3.3 Components The insulation distances of transformers consist of various windings of paper, pressboard and wood, and insulating oil. When components of different potentials are insulated against each other by paper and / or oil, they are capacitors. The properties of the capacitors are defined by geometry (area, distance) and composition of the dielectric. It has been shown that moisture in paper has less influence on dissipation-factor measurement than conductivity of the oil. 3.4 Influencing factors As described above, the result of the dissipation-factor measurement is determined by transformer insulation. Basically, moisture and acidity in the paper and composition of the oil do have influence. The ground loop of the transformer to the test equipment has an additional, important influence. Temperature dependency of the transformer plays an important role. It is generally recommended that the measurements are always performed at the same temperature, close to 20 °C. 3.5 Measuring principle All phases of a winding are short-circuited, and primary winding is measured to secondary winding and to tank. The most important capacitance is the one between primary and secondary winding. The other capacitances can also be measured, but they are of secondary importance for transformer diagnosis. Depending on the rated voltage of the transformer, between 2 kV and 12 kV can typically be tested in the voltage run and 15 Hz to 400 Hz in the frequency run. There are no specific designations defined for the dissipation factors and capacitances. In Figure 19, all capacitances that are possible on a three-winding transformer are shown as example, in accordance with the naming conventions used by Omicron (equipment manufacturer). The same designation logic is valid for any other equipment. 34/101 Part II Version 01 PSP Diagnostics Compendium Part II_V01_EN.docx primary winding secondary winding tertiary winding CHL CLT CH CL CHT CT Figure 19 – Three-winding transformer: names of all capacitances Legend: H = high voltage winding, L = low voltage winding, T = tertiary winding 3.6 Evaluation A measurement is plausible when the capacitances are constant in both the voltage and frequency run, and are in the range of Nanofarad (nF = 10−9 F) or Pikofarad (pF = 10−12 F). For the three-winding transformer, this relationship is shown in the upper halves of Figure 20 and Figure 21. A measurement is plausible when the dissipation factors are constant in the voltage run. In the frequency run from lower to higher frequencies, it is normal that the dissipation factor increases. These relationships are shown in the lower halves of Figure 20 and Figure 21 for a three-winding transformer 35/101 Part II Version 01 PSP Diagnostics Compendium Part II_V01_EN.docx Figure 20 – Dissipation factor on the transformer: Voltage run for capacitances and dissipation factors of a three-winding transformer, FFAC15118 The graph for tan δ cor ICHT in Figure 20 shows a change in the voltage run, and all the values between primary and tertiary winding are close to or below zero. One the one hand, this insulation distance between primary and tertiary winding is difficult to measure because capacitance is very low, based on the transformer geometry, and on the other hand, it is of little importance from an physical point of view. Therefore, when tertiary windings are involved in dissipation-factor measurements on site, a graph that is different from other measurements is often accepted. 36/101 Part II Version 01 PSP Diagnostics Compendium Part II_V01_EN.docx Figure 21 – Dissipation factor on the transformer: Frequency run for capacitances and dissipation factors of a three-winding transformer, FFAC15118 At rated frequency and an oil temperature of 20 °C, the value of 0.5 % is typical for the dissipation factor, whereas 1 % is considered as borderline. Low or negative dissipation factors are not plausible. If they do occur, the test setup must be changed, see section 2.2.7 and section 2.6. All the contacts must be cleaned and dried, the test setup with cross- section enlargement or ground leads of the test setup must be changed. In addition, the transition resistance of the test equipment grounding to the transformer should be changed. 37/101 Part II Version 01 PSP Diagnostics Compendium Part II_V01_EN.docx 4 Dissipation factor and capacitance measurement on bushings 4.1 General In the following, the term dissipation-factor measurement is used as synonym for measurement of capacitances and tan δ or Tangens Delta. When discussing diagnosis on site with the user, dissipation-factor measurement on bushings used to mean HV measurement with voltage run up to 12 kV and frequency run of 15 to 400 Hz. In the future, more and more measurements of the dielectric frequency response (FDS method) of bushings will be used. This should be taken into consideration when dealing with the customer. When the FDS method for frequencies up to 10 μHz is used, the influence of moisture on bushings can be shown in greater detail. The advantage of the 12 kV measurement is that it is easier to detect contact problems of the test tap using the voltage run. Bushings can be measured when they are condenser bushings and equipped with a test tap at the flange of the bushing, see Figure 22 as an example. This applies in particular to bushings for voltages including 110 kV and more. Figure 22 – Bushing: Typical test tap (left), American version (right) Source: Cigré, Working Group A2.43, Brochure 755 Transformer bushing reliability, Paris, 2019 There is a possibility to retrofit condenser bushings with insulation in order to test them. This is done using insulating sleeves and washers and an insulating gasket that separates transformer and bushing flange electrically. This setup should provide an insulation resistance of at least 10 kΩ. During operation, a potential lead must connect transformer and bushing. The lead must be removed for capacitance or dissipation-factor measurement. The bushing flange can then be used as test tap. It is important not to mistake the vent screw for test tap. All elements of this section are shown and labelled in Figure 23. 38/101 Part II Version 01 PSP Diagnostics Compendium Part II_V01_EN.docx Vent screw Potential lead Figure 23 – Bushing: insulation installed later on Source: Cigré, Working Group A2.43, Brochure 755 Transformer bushing reliability, Paris, 2019 Aluminum layers in bushings are used in order to achieve a more homogeneous field distribution, that is necessary for higher voltages, along the bushing conductor. This is done by wrapping electrically conductive foils in insulation material such as paper, see Figure 24 and Figure 25. 39/101 Part II Version 01 PSP Diagnostics Compendium Part II_V01_EN.docx Figure 24 – Bushing: cross section of middle tube, paper insulation and condenser layers Source: Krüger, Dr. M: Diagnostic Measurements on High Voltage Bushings, Presentation Omicron Energy, AT, 2014 40/101 Part II Version 01 PSP Diagnostics Compendium Part II_V01_EN.docx Figure 25 – Bushing: Schematic overview of condenser coatings and insulation material Source: Krüger, Dr. M: Diagnostic Measurements on High Voltage Bushings, Presentation Omicron Energy, AT, 2014 41/101 Part II Version 01 PSP Diagnostics Compendium Part II_V01_EN.docx The insulation between the single condenser layers is crucial for operational safety of transformer and condenser bushing. Dielectric measurements therefore concentrate on aging and insulation condition between the condenser layers and any additional insulation that might be present between wrapping and outer insulator, see Figure 26. Figure 26 – Bushing: Setup with test tap and condenser body Source: ABB, ABB, Transformer Bushings type GOB (OIP) - Technical Guide, Ludvika, SE, 2010. 4.2 Definition of terms The statements presented in 2.2.1 are valid. Diagnosis using dissipation-factor measurement on bushings is based on voltage and frequency run. In most cases, sine-wave 50-Hz voltage in steps of 2 kV up to 12 kV and typical frequencies of 15 Hz, 20 Hz, 35 Hz, 50 Hz, 135 Hz, 220 Hz, 305 Hz, 400 Hz are used for measurement. In addition, capacitances are always measured as well. After manufacture, bushings are measured at laboratory conditions with rated voltage, rated frequency and at 20 °C. Listed as VF, tan δ or similar in the test protocol, this value is part of the transformer book, which is handed over to the customer; it is also printed on the bushing nameplate. It is absolutely necessary to find out this value on site and document it in a suitable way. Exposure to weather conditions can make it difficult to read the nameplate, therefore the transformer book should basically and always be requested on site. Experience over the past years has shown that measured values of capacitance and dissipation factor at nominal frequency alone are not significant in regard to aging of the bushing. This is partly because the influence of water at rated frequency cannot be measured. On the other hand, the environment of 42/101 Part II Version 01 PSP Diagnostics Compendium Part II_V01_EN.docx the bushing in the laboratory is different from its condition when installed in the transformer. Consequently, the dissipation factor of the bushing after it has been installed in the transformer is lower than the dissipation factor measured in the lab. With aging, the dissipation factor increases again over time and reaches the nominal value. That is, dissipation factor values of a 15 year old bushing that are in the range of the nominal value, can already mean aging. In regard to measurements on site, it is important to know that measured values around the nominal value or less may well be plausible. Regarding the measurement itself, bushing technology differences are irrelevant. However, basic knowledge is necessary for technical discussions and clarification of plausibility. An overview of typical technologies is therefore shown in Figure 27. Figure 27 – Bushings: Overview manufacturing technologies and distribution in the market Source: Krüger, Dr. M: Diagnostic Measurements on High Voltage Bushings, Presentation Omicron Energy, AT, 2014 4.3 Components Capacitance C̅1 and dissipation factor of this capacitance are measured, by applying voltage to the bushing pin and measuring back over the test tap. Loss of condenser layers increases measured capacitance. Supply voltage through the grid remains constant, with less condenser layers, the voltage load per condenser layer will be increased. In the worst case, this leads to further flashovers. Inside the bushing, the influence of the insulating material on the measurement depends on the technology. Moisture can have a strong influence on paper and oil insulation. No moisture can penetrate into resin-impregnated synthetic foil bushings, or "RIS" for short, and resin-impregnated GRP bushings, or "RIF" for short. Originally in RBP bushings, resin in the wrapping excludes oil and moisture. Cracks develop over time, and oil penetrates into the holes. This will change capacitance and dissipation factor 43/101 Part II Version 01 PSP Diagnostics Compendium Part II_V01_EN.docx without creating a situation that is critical for operation. Furthermore, this is the reason for considerably increased limit values of RBP bushings. 44/101 Part II Version 01 PSP Diagnostics Compendium Part II_V01_EN.docx 4.4 Influencing factors Design and manufacturing process determine capacitance and dissipation factor. These values do not change normally. If dissipation factor or capacitance changes, these changes often indicate specific aging processes, dirt or faults. But the measurement is also strongly influenced by external influences. In the following, the influences will be described in detail to be able to differentiate between avoidable external influences and faults. As they are a capacitive arrangements, bushings must be disconnected on all sides and a distance of at least 10 cm must be kept to adjacent conductive parts. Short-circuit bridges or cables used as potential equalization must be sufficiently distant from components with ground potential, since the applied high voltages may cause insulation damage to cables or incorrect measurements. General humidity of the surrounding air can also influence the measurement. Always be sure to document ambient conditions well, to be able to evaluate the result correctly even with very unfavorable conditions. Attachment of the HV cable that is part of the test equipment can also influence the measurement considerably. The HV cable has a grounded shield almost over its whole length. If the cable rests on components with HV potential, partial discharges between cable shield and component occur above approx. 4 kV, which can make the measurement useless and damage components. The last approx. 50 cm of the cable insulation consists of the cable jacket only. This part may touch conductive parts which have been brought to HV potential. But this should be avoided. Therefore it is very important to keep sufficient distance when connecting the HV cable to the bushing. See Figure 28 for a favorable test setup. The support used in this case is available at ST3 and should be taken to the site as a precaution when performing measurements since only the situation on site makes a decision possible as to whether it should be used or not. 45/101 Part II Version 01 PSP Diagnostics Compendium Part II_V01_EN.docx Figure 28 – Bushing: Test setup with HV cable and potential connection using spiral cable The test setup shown in Figure 29 leads to dissipation factors that clearly depend on voltage, that is, it leads to faulty measurements. 46/101 Part II Version 01 PSP Diagnostics Compendium Part II_V01_EN.docx Figure 29 – Bushing: Test setup with HV cable leads to measurement errors due to partial discharges 47/101 Part II Version 01 PSP Diagnostics Compendium Part II_V01_EN.docx Temperature influences the test result substantially. In the ideal case, measurements on bushings should be performed at 20 °C. Minimum temperature for performing a measurement is 5 °C. Below this value, the aggregate state of water is variable, rendering the dissipation factor test method unreliable. To be able to correct test values later on, the following three temperatures are recorded per bushing, before and after every measurement: 1. Flange temperature at bushing base, corresponding to highest oil temperature in the transformer, as the case may be, 2. Temperature on bushing head, and 3. Ambient temperature. The temperatures should be recorded before and after every single bushing measurement. The corresponding table is available in appendix A.1. If it is not possible to use a pyrometer or an infrared camera for the measurements, the mean value of ambient temperature and highest oil temperature of the transformer must be used. Surface currents over the outmost layer or exterior of the component must be prevented. Dirt and humidity on the bushing surfaces must be removed. It is important not to leave any textile fibers or oil films behind. Cleaning agents recommended by the manufacturer must be used especially for silicon bushings. When using the guard, see section 2.5, cleaning of the bushing is always recommended. The influence of contact transition resistance existing on bushing connection and test tap has proven to be of major importance. In addition, humidity on the test tap at the bushing flange modifies the measurement. Use a blow-dryer to dry the test tap. Bad ground connections lead to negative or low dissipation factors. Therefore varnish, grease and dirt must be removed from ground connections on the transformer, see section 2.6. Capacitance C̅2 is present between test tap and transformer tank. Capacitance depends on the transformer; therefore it can only be compared with previous measurements. 48/101 Part II Version 01 PSP Diagnostics Compendium Part II_V01_EN.docx 4.5 Measuring principle For measuring capacitance C̅1 , the test equipment output is connected to the connecting bolt of the bushing. The test tap on the bushing flange is used for the return measurement. Voltage and frequency run are performed for all bushings that need to be measured. Capacitance C̅2 is measured by applying max. 500 V at nominal frequency to the test tap. The ground loop is used for the return measurement. 4.6 Evaluation First find out which technology is used for the bushing; then you can check plausibility of the measured values. Please note that the exterior appearance cannot be used to identify the technology clearly. For example, not every oil-filled bushing must have an expansion tank or a sight glass. Three factors are used to check plausibility: Value range, Deviation of measured values from manufacturer tests at rated frequency and 20 °C, evaluated in accordance with the standards, see Figure 30 as nameplate example, Course of graphs in regard to the measurements, and Comparison between the phases. Figure 30 – High-voltage bushing: Nameplate If the measured values are too high, a repeat measurement with cleaned insulation fins and guard should be performed. The guard should be connected to the second or third fin of the bushing, see guard in Figure 28 and Figure 29. If the values are clearly too low, resistance of the ground loop must be reduced, see section 2.6. This is especially true for negative values as in Figure 31. At least the negative dissipation factors indicate an incorrect test setup. The course of the graphs and the range of measured values for the capacitances 49/101 Part II Version 01 PSP Diagnostics Compendium Part II_V01_EN.docx seems plausible. But on closer inspection it can be noticed that the graphs are voltage-dependent here, too, indicating the incorrect test setup. Figure 31 – Bushing: Frequency run for capacitances and negative dissipation factors, MR185904 It can be plausible if the bushing of the neutral conductor is different when comparing it directly, as - presupposing symmetrical network operation and load - the neutral conductor does not carry any current and therefore has lower temperatures, which results in slower aging. In addition, as neutral conductor bushings are often designed for lower operating voltages, capacitance can be lower, too, see Figure 32 and Figure 33. 50/101 Part II Version 01 PSP Diagnostics Compendium Part II_V01_EN.docx Figure 32 – OIP bushing: dissipation factor frequency run with moisture in bushing of phase V, deviating measurement for neutral conductor; MR083167 Figure 33 – OIP bushing: dissipation factor voltage run; MR083167 However, it is important to ensure that the measured values are not too low. This is shown using Figure 34 as an example, where the course of the dissipation factor seems plausible at first glance. For the N bushing, the measured value for 10 kV at 50 Hz is 0.11 % after temperature correction. The dissipation factor for new OIP bushings is typically between 0.2 % and 0.4 %, see Table 5 for details. It can be assumed in the shown measurement that the resistance of the ground loop is too high, and an increased contact-circuit resistance in the ground loop must be assumed. In this case, measures as described in section 2.6 were therefore taken, and should be taken in similar cases. 51/101 Part II Version 01 PSP Diagnostics Compendium Part II_V01_EN.docx Figure 34 – Bushing: Note very low values of dissipation-factor measurement for bushing N up to 50 Hz, MR1014185 Voltage-dependent dissipation factors as in Figure 35 indicate contact problems of the test tap to the last condenser layer. If such measurements occur, the bushing must be taken out of service. Figure 35 – Bushing: Voltage run on RIP bushings with irregular test contact (red) and regular test contact (blue) Source: Cigré, Working Group A2.43, Brochure 755 Transformer bushing reliability, Paris, 2019 It is true for dissipation factors that positive measured values and a regular course of the graphs that is similar in all three phases is a good indication of the plausibility of the measurements. If you compare the measured values with the manufacturer's values for dissipation factors at rated frequency and check the deviations according to Table 5, this can be another rough indication of plausibility when you are on site. 52/101 Part II Version 01 PSP Diagnostics Compendium Part II_V01_EN.docx Table 5 below applies to dissipation factors at rated frequency and 20 °C for bushings, depending on the manufacturing technology: Table 5 – Dissipation factors: Typical values and limit values for bushings at rated frequency and 20 °C Type RIP resin impregnated OIP oil impregnated RBP hard paper soft paper IEC 60137 < 0.7 < 0.7 < 1.5 IEEE C57.10.01 < 0.85