Protein Chemistry: Protein Structures PDF
Document Details
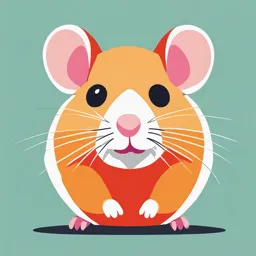
Uploaded by FriendlyMountain
University of Health and Allied Sciences
RUPERT K. DELIMINI
Tags
Summary
This document provides a lecture outline on protein structures, covering topics like amino acid composition, methods for determining sequences and the importance of the primary structure for peptide synthesis, protein sorting, and covalent modification of polypeptides. The document also has introductory information and explains the different levels of protein structures. It's designed for an undergraduate level biology course.
Full Transcript
PROTEIN CHEMISTRY Protein Structures 6/17/2024 1 Lecture Outline Amino acid composition of proteins Methods for determining amino acid sequence e.g. mass spectrometry. Importance of primary structure synthesis of peptides Protein sorting and traffick...
PROTEIN CHEMISTRY Protein Structures 6/17/2024 1 Lecture Outline Amino acid composition of proteins Methods for determining amino acid sequence e.g. mass spectrometry. Importance of primary structure synthesis of peptides Protein sorting and trafficking Covalent modification of polypeptides 6/17/2024 2 Introduction Purification of a protein is usually only a prelude to a detailed biochemical dissection of its structure and function. What is it that makes one protein an enzyme, another a hormone, another a structural protein, and still another an antibody? How do they differ chemically? The most obvious distinctions are structural, and to protein structure we now turn. 6/17/2024 3 Introduction There are four levels of Protein Structure. A description of all covalent bonds (mainly peptide bonds and disulfide bonds) linking amino acid residues in a polypeptide chain is its primary structure. The most important element of primary structure is the sequence of amino acid residues. Secondary structure refers to particularly stable arrangements of amino acid residues giving rise to recurring structural patterns. Tertiary structure describes all aspects of the three-dimensional folding of a polypeptide. When a protein has two or more polypeptide subunits, their arrangement in space is referred to as quaternary structure. 6/17/2024 4 Introduction The instructions for the unique shape of a protein are contained entirely within the linear amino acid sequence- primary structure Four hierarchy of protein structure Primary Secondary Tertiary Quatenary Amino acids are linked together in a peptide bond( an amide bond) The COO group of the preceding amino acid links to the NH2 of the next amino acid. The alpha carbons are separated by 3 covalent bonds Cα – C – N- Cα This peptide bond connects all the residues of a polypeptide chain and constitute the polypeptide backbone. 6/17/2024 5 6/17/2024 6 Types of protein structures 6/17/2024 7 Primary Structure 6/17/2024 8 Introduction Each protein has a distinctive number and sequence of amino acid residues. The primary structure of a protein determines how it folds up into its unique three-dimensional structure, and this in turn determines the function of the protein. 6/17/2024 9 The Function of a Protein Depends on it’s Amino Acid Sequence The bacterium E.coli produces more than 3,000 different proteins; a human has -25,000 genes encoding a much larger number of protein. In both cases, each type of protein has a unique three- dimensional structure and this structure confers a unique function. Each type of protein also has a unique amino acid sequence The amino acid sequence play a fundamental role in determining the three-dimensional structure of the protein, and ultimately its function. There exists an important relationship between amino acid sequence and biological function. Is there a basis for this claim? 6/17/2024 10 The Function of a Protein Depends on it’s Amino Acid Sequence Proteins with different functions always have different amino acid sequences. Thousands of human genetic diseases have been traced to the production of defective proteins. The defect can range from a single change in the amino acid sequence (as in sickle cell anemia) to deletion of a larger portion of the polypeptide chain (as in most cases of Duchenne muscular dystrophy: a large deletion in the gene encoding the protein dystrophin leads to production of a shortened, inactive protein) Thus we know that if the primary structure is altered, the function of the protein may also be changed. 6/17/2024 11 The Function of a Protein Depends on it’s Amino Acid Sequence Finally, on comparing functionally similar proteins from different species, we find that these proteins often have similar amino acid sequences. An extreme case is ubiquitin, a 76-residue protein involved in regulating the degradation of other proteins. The amino acid sequence of ubiquitin is identical in species as different as fruit flies and humans. Is the amino acid sequence absolutely fixed, or invariant? 6/17/2024 12 The Function of a Protein Depends on it’s Amino Acid Sequence An estimated 20% to 30% of the proteins in humans are polymorphic, having amino acid sequence variants in the human population. Many of these variations in sequence have little or no effect on the function of the protein. Furthermore, proteins that carry out a broadly similar function in distantly related species can differ greatly in overall size and amino acid sequence. Although the amino acid sequence in some regions of the primary structure might vary considerably without affecting biological function, most proteins contain crucial regions that are essential to their function and whose sequence is therefore conserved. 6/17/2024 13 Methods for determining amino acid sequence 6/17/2024 14 Methods for determining amino acid sequence Two major discoveries in 1953 were of crucial importance in the history of biochemistry. In that year, James D. Watson and Francis Crick deduced the double-helical structure of DNA and proposed a structural basis for its precise replication. Their proposal illuminated the molecular reality behind the idea of a gene. In the same year, Frederick Sanger worked out the sequence of amino acid residues in the polypeptide chains of the hormone insulin surprising many researchers who had long thought that determining the amino acid sequence of a polypeptide would be a hopelessly difficult task. 6/17/2024 15 Amino acid sequence of bovine insulin 6/17/2024 16 Methods for determining amino acid sequence It quickly became evident that the nucleotide sequence in DNA and the amino acid sequence in proteins were somehow related. Barely a decade after these discoveries, the genetic code relating the nucleotide sequence of DNA to the amino acid sequence of protein molecules was elucidated. An enormous number of protein sequences can now be derived indirectly from the DNA sequences in the rapidly growing genome database 6/17/2024 17 Mass spectrometry reveals amino acid sequences A standard approach to sequencing a protein has several steps: However, firstly, the sample of protein to be sequenced is purified (for example, by chromatographic or other methods) so that it is free of other proteins. The approach to purification of a protein that has not previously been isolated is guided both by established precedents and by common sense. In most cases, several different methods must be used sequentially to purify a protein completely, each method separating proteins on the basis of different properties. The choice of methods is some what empirical, and many strategies maybe tried before the most effective one is found. Trial and error can often be minimized by basing the procedure on purification techniques developed for similar proteins. 6/17/2024 18 Protein separation and purification A pure preparation is essential before a protein's properties and activities can be determined. Given that cells contain thousands of different kinds of proteins, how can one protein be purified? Classical methods for separating proteins take advantage of properties that vary from one protein to the next, including size, charge, and binding properties. 6/17/2024 19 Protein separation and purification The source of a protein is generally tissue or microbial cells. The first step in any protein purification procedure is to break open these cells, releasing their proteins into a solution called a crude extract. Once the extract or organelle preparation is ready, various methods are available for purifying one or more of the proteins it contains. Commonly, the extract is subjected to treatments that separate the proteins into different fractions base on a property such as size or charge, a process referred to as fractionation. 6/17/2024 20 General purification strategies The most powerful methods for fractionating proteins make use of column chromatography. This takes advantage of differences in protein size, charge, binding affinity and other properties. A porous solid material with appropriate chemical properties (the stationary phase) is held in a column, and a buffered solution (mobile phase) percolates through it. The protein-containing solution, layered on the top of the column, percolates through the solid matrix as an ever expanding band within the larger mobile phase. Individual proteins migrate faster or slower through the column depending on their properties 6/17/2024 21 Column chromatography 6/17/2024 22 Ion-exchange chromatography This exploits differences in the sign and magnitude of the net electric charge of protein at a pH. The column matrix is a synthetic polymer (resin) containing bound charged groups; those with bound anionic groups are called cation exchangers, and those with bound cationic groups are called anion exchanges. Cation-exchange chromatography The solid matrix has negatively charged group. In the mobile phase, proteins with a net positive charge migrate through the matrix more slowly than those with a net negative charge. Because the migration of the former is retarded more by 6/17/2024 23 interaction with the stationary phase Ion-exchange Chromatography 6/17/2024 24 Size-exclusion & Affinity Chromatography 6/17/2024 25 Other protein separation Techniques Gel electrophoresis 6/17/2024 26 Isoelectric focusing and 2- Dimensional electrophoresis 6/17/2024 27 Mass spectrometry reveals amino acid sequences If the protein contains more than one kind of polypeptide chain, the chains are separated so that each can be individually sequenced. In some cases, this requires breaking (reducing) disulfide bonds. Large polypeptides must be broken into smaller pieces (100 residues) that can be individually sequenced. Cleavage can be accomplished chemically, for example, by treating the polypeptide with cyanogen bromide (CNBr), which cleaves the peptide bond on the C-terminal side of Met residues. Cleavage can also be accomplished with proteases (another term for peptidases) that hydrolyze specific peptide bonds. For example, the protease trypsin cleaves the peptide bond on the C-terminal side of the positively charged residues Arg and Lys. 6/17/2024 28 Mass spectrometry reveals amino acid sequences Each peptide is sequenced in a classic procedure known as Edman degradation. Alternatively, each peptide can be sequenced by mass spectrometry that analyzes protein structure by measuring the sizes of peptide fragments. In standard mass spectrometry, a solution of the protein (or another macromolecule) is sprayed from a tiny nozzle at high voltage. This yields droplets of positively charged molecular fragments, from which the solvent quickly evaporates. Each gas-phase ion then passes through an electric field. The ions are deflected, with smaller ions deflected more than larger ions, so that they are separated by their mass-to-charge ratios. In this way, the masses of the fragments can be measured and the mass of the intact molecule can be deduced 6/17/2024 29 Mass spectrometry reveals amino acid sequences 6/17/2024 30 Mass spectrometry reveals amino acid sequences Two mass spectrometers in series can be used to determine the sequence of amino acids in a polypeptide. The first instrument sorts the peptide ions so that only one emerges. This species is then allowed to collide with an inert gas, such as helium, which breaks the peptide, usually at a peptide bond. The second mass spectrometer then measures the mass-to- charge ratios of the peptide fragments. 6/17/2024 31 Mass spectrometry reveals amino acid sequences A solution of charged peptides is sprayed into the first mass spectrometer (MS1). One peptide ion is selected to enter the collision chamber to be fragmented. The second mass spectrometer (MS2) then measures the mass- to-charge ratio of the ionic peptide fragments. The peptide sequence is determined by comparing the masses of increasingly larger fragments 6/17/2024 32 An example of peptide sequencing by mass spectrometry. The difference in mass of each successive peak identifies each residue, allowing the amino acid sequence to be read from right to left 6/17/2024 33 Mass Spectrometry Application Mass spectrometry has been used for several decades in clinical and forensics laboratories to identify normal metabolic compounds as well as toxins and drugs (both therapeutic and illicit). Instruments that detect traces of explosives at airport security checkpoints also use mass spectrometry, which is rapid, sensitive, and reliable. The analysis of small compounds by mass spectrometry is relatively straightforward. It is much more challenging, however, to analyze large molecules in complex mixtures for the purpose of diagnosing a disease such as cancer or tracking its effects on human tissue 6/17/2024 34 Mass Spectrometry Application Fluids such as blood contain so many different proteins, with concentrations ranging over many order of magnitude, that it is difficult to detect rare proteins against a backdrop of very abundant ones such as serum albumin and immunoglobulins, which together account for about 75% of the plasma proteins. Urinalysis by mass spectrometry holds more promise, as urine normally contains relatively few proteins and none with a mass greater than about 15,000 D. Even so, over 2000 different proteins are detectable in urine. 6/17/2024 35 Mass Spectrometry Application One common approach for identifying proteins in biological samples is to fractionate the mixture by electrophoresis, then extract the separated proteins from the gel, partially digest them with a protease, and subject them to mass spectrometry. The resulting pattern of peaks, a “fingerprint,” can be compared to a database to identify the protein. A partial sequence of just a few amino acids is often sufficient to identify the parent protein. Of course, the availability of complete genomic sequences makes this approach possible, and a number of software programs have been developed to translate mass spectral data into query sequences for searching sequence databases 6/17/2024 36 Protein structures are determined by X-ray crystallography, electron crystallography, and NMR spectroscopy Most proteins are too small to be directly visualized, even by electron microscopy, but their atomic structures are accessible to high-energy probes in the form of X-rays. X-Ray crystallography is performed on samples of protein that have been induced to form crystals. A protein preparation must be exceptionally pure in order to crystallize without imperfections. A protein crystal, often no more than 0.5 mm in diameter, usually contains 40–70% water by volume and is therefore more gel-like than solid. Crystals of the protein streptavidin. 6/17/2024 37 Protein structures are determined by X-ray crystallography, electron crystallography, and NMR spectroscopy When bombarded with a narrow beam of X-rays, the electrons of the atoms in the crystal scatter the X-rays, which reinforce and cancel each other to produce a diffraction pattern of light and dark spots that can be captured electronically or on a piece of X-ray film. Mathematical analysis of the intensities and positions of the diffracted X-rays yields a three-dimensional map of electron density in the crystallized molecule. The level of detail of the image depends in part on the quality of the crystal. An X-ray diffraction pattern 6/17/2024 38 Protein structures are determined by X-ray crystallography, electron crystallography, and NMR spectroscopy Proteins in solution can be analyzed by nuclear magnetic resonance (NMR) spectroscopy, which takes advantage of the ability of atomic nuclei (most commonly, hydrogen) to resonate in an applied magnetic field according to their interactions with nearby atoms. An NMR spectrum consists of numerous peaks that can be analyzed to reveal the distances between two H atoms that are close together in space or are covalently connected through one or two other atoms. These measurements, along with information about the protein’s amino acid sequence, are used to construct a three-dimensional model of the protein. The NMR structure of glutaredoxin 6/17/2024 39 Importance of knowing the primary structure 6/17/2024 40 Protein phylogenetics and Evolution Changes in amino acid sequence during evolution can indicate the relatedness of species and also amino acid residues that are conversed across species Phylogenetic analyses are used to understand evolutionary relationships determine the spread of infectious agents explore biogeographic processes detect genetic recombination in multiple loci 6/17/2024 41 Understanding evolution through protein sequence analysis The amino acid sequence of proteins provides a wealth of information. As more protein sequences become available, more powerful methods for extracting information from them has become a major biochemical enterprise. Analysis of information available in many biological databases including gene and protein sequences and macromolecular structures has given rise to the new field of Bioinformatics Bioinformatics tools has made it possible to identify functional segments in new proteins and help establish both their sequence and their structural relationships to proteins already in the database. On another level, protein sequences are beginning to tell us how the proteins evolved and, ultimately, how life evolved on this planet 6/17/2024 42 Understanding evolution through protein sequence analysis The study of molecular evolution generally focuses on families of closely related proteins. These protein families chosen for analysis have essential functions in cellular metabolism that must have been present in the earliest viable cells, thus greatly reducing the chance that they were introduced relatively recently. Example, a protein called EF-1α (elongation factor 1α) is involved in the synthesis of proteins in all eukaryotes. A similar protein, EF-Tu, with the same function, is found in bacteria. Similarities in sequence and function indicate that EF-1α and EF-Tu are members of a family of proteins that share a common ancestor. The members of protein families are called homologous proteins, or homologs. 6/17/2024 43 Understanding evolution through protein sequence analysis If two proteins in a family (that is, two homologs) are present in the same species, they are referred to as paralogs. Homologs from different species are called orthologs. The process of tracing evolution involves first identifying suitable families of homologous proteins and then using them to reconstruct evolutionary paths 6/17/2024 44 Evolutionary tree derived from amino acid sequence comparison A bacterial evolutionary tree, based on the sequence divergence observed in the GroEL family of proteins. Also included in this tree (lower right) are the chloroplasts (chl.) of some nonbacterial species 6/17/2024 45 A consensus tree of life The tree shown here is based on analyses of many different protein sequences and additional genomic features. Branches shown as dashed lines remain under investigation. The tree presents only a fraction of the available information, as well as only a fraction of the issues remaining to be resolved. Each extant group shown is a complex evolutionary story unto itself 6/17/2024 46 Conserved amino acids likely have important functions in the protein 6/17/2024 47 Protein Secondary Structure 6/17/2024 48 Lecture Outline β- pleated sheets α-helix Fibrous protein structure Circular Dichroism 6/17/2024 49 Overview of protein structure In the late 1930s, Linus Pauling and Robert Corey embarked on a series of studies that laid the foundation for our current understanding of protein structure. They began with a careful analysis of the peptide bond. 6/17/2024 50 Overview of protein structure The spatial arrangement of atoms in a protein is called its conformation. Proteins in any of their functional, folded conformations are called native proteins. The α carbons of adjacent amino acid residues are separated by three covalent bonds, arranged as Cα-C-N-Cα. Peptide C-N bond is somewhat shorter than the C-N bond in a simple amine and that the atoms associated with the peptide bond are co- planar ( in the same plane). There exists partial sharing of two pairs of electrons between the carbonyl oxygen and the amide nitrogen. The oxygen has a partial negative charge and the nitrogen a partial positive charge, setting up a small electric dipole 6/17/2024 51 Overview of protein structure From these findings Pauling and Corey concluded that the peptide C-N bonds, because of their partial double-bond character, cannot rotate freely. 6/17/2024 52 Secondary Structure 6/17/2024 53 Protein Secondary Structure The term secondary structure refers to any chosen segment of a polypeptide chain and describes the local spatial arrangement of its main-chain atoms, without regard to the conformation of its side chains or its relationship to other segment. The most prominent are the α helix and β conformation. The simplest arrangement the polypeptide chain can assume, given its rigid peptide bonds (but free rotation around its other, single bonds), is a helical structure which Pauling and Corey called the α helix 6/17/2024 54 Models of the α helix, showing different aspects of its structure α helix viewed from one end, looking down the Space-filling model shows Ball & stick model showing longitudinal axis, R- that atoms in center are in intrachain hydrogen bond groups represented as very close contact 6/17/2024 purple spheres 55 α helix In all proteins, the helical twist of the o helix is right-handed. 6/17/2024 56 α helix The α helix proved to be the predominant structure in a-keratins. More generally, about one-fourth of all amino acid residues in proteins are found in α helices, the exact fraction varying greatly from one protein to another. Why does the a helix form more readily than many other possible conformations? α helix makes optimal use of internal hydrogen bonds. The structure is stabilized by a hydrogen bond between the hydrogen atom attached to the electronegative nitrogen atom of a peptide linkage and the electronegative carbonyl oxygen atom of the fourth amino acid on the amino-terminal side of that peptide bond. Within the α helix, every peptide bond (except those close to each end of the helix) participates in such hydrogen bonding. Each successive turn of the α helix is held to adjacent turns by three to four hydrogen bonds, conferring significant stability on the overall structure 6/17/2024 57 α helix Constraint on the formation of the α helix is the presence of Pro or Gly residues, which have the least proclivity to form α helices. In proline, the nitrogen atom is part of a rigid ring, and rotation about the N-C. bond is not possible. Thus, a Pro residue introduces a destabilizing kink in an α helix. In addition, the nitrogen atom of a Pro residue in a peptide linkage has no substituent hydrogen to participate in hydrogen bonds with other residues. For these reasons, proline is only rarely found in an α helix. Glycine occurs infrequently in α helices: it has more conformational flexibility than the other amino acid residues. 6/17/2024 58 α helix In summary, five types of constraints affect the stability of an a helix: (1) the intrinsic propensity of an amino acid residue to form an a helix; (2) the interactions between R groups, particularly those spaced three (or four) residues apart (3) the bulkiness of adjacent R groups (4) the occurrence of Pro and Gly residues (5) interactions between amino acid residues at the ends of the helical segment and the electric dipole inherent to the a helix. The tendency of a given segment of a polypeptide chain to form an a helix therefore depends on the identity and sequence of amino acid residues within the segment. 6/17/2024 59 The β conformation organizes polypeptide chains into Sheet This is a more extended conformation of polypeptide chains, and its structure has been confirmed by x-ray analysis. In the β conformation, the backbone of the polypeptide chain is extended into a zigzag rather than helical structure. 6/17/2024 60 The β conformation organizes polypeptide chains into Sheet The zigzag polypeptide chains can be arranged side by side to form a structure resembling a series of pleats. In this arrangement, called a β sheet, hydrogen bonds form between adjacent segments of polypeptide chain. The individual segments that form a β sheet are usually nearby on the polypeptide chain, but can also be quite distant from each other in the linear sequence of the polypeptide; they may even be in different polypeptide chains. The R groups of adjacent amino acids protrude from the zigzag structure in opposite directions, creating the alternating pattern seen in the side views 6/17/2024 61 The β conformation organizes polypeptide chains into Sheet Some protein structures limit the kinds of amino acids that can occur in the β sheet. When two or more β sheets are layered close together within a protein, the R groups of the amino acid residues on the touching surfaces must be relatively small. β -Keratins such as silk fibroin and the fibroin of spider webs have a very high content of Gly and AIa residues, the two amino acids with the smallest R groups. Indeed, in silk fibroin GIy and AIa alternate over large parts of the sequence. 6/17/2024 62 Backbone structure of carboxypeptidase A Proteins contain mixtures of α- helices and β-sheets as shown here for carboxypeptidase. Helices are blue and sheets are green. Regions without secondary structure are orange often referred to as loop/turns as they are connecting elements that link successive runs of α helix or β conformation. Note that the β-sheets form a twisted structure sometimes resembling a propeller 6/17/2024 63 Fibrous Proteins Proteins can be classified into two major groups: fibrous proteins, with polypeptide chains arranged in long strands or sheets, and globular proteins, with polypeptide chains folded into a spherical or globular shape. The two groups are structurally distinct. Fibrous proteins usually consist largely of a single type of secondary structure, and their tertiary structure is relatively simple Globular proteins often contain several types of secondary structure. The two groups also differ functionally: the structures that provide support, shape, and external protection to vertebrates are made of fibrous proteins, whereas most enzymes and regulatory proteins are globular proteins 6/17/2024 64 Fibrous Proteins 6/17/2024 65 Fibrous Proteins Fibrous proteins are highly elongated molecules dominated by a single type of secondary structure. Keratin is a fibrous protein found in hair, nails, horns and feathers. It is also the dominant protein in the outer layer of our skin, the epidermis Keratin has a coiled-coil structure that resembles 2 interwoven α-helices with a pitch of 5.1Å rather than the 5.4Å of α-helix. The two helices form a left-handed coil. 6/17/2024 66 Higher Order Organization of Keratin Fibrils Keratin is rich in Cys residues and considerable cross-linking can occur, especially in curly hair. Relaxing of curly hair involves reducing agents that reduce Cys cross-links followed by re-oxidation. 6/17/2024 67 Permanent Waving is Biochemical engineering 6/17/2024 68 Collagen Like the α-keratins, collagen has evolved to provide strength. It is found in connective tissue such as tendons, cartilage, the organic matrix of bone, and the cornea of the eye. Collagen is another ubiquitous fibrous protein found in many structures including bone, teeth, cartilage, tendon, skin, eyes and blood vessels. It is the most abundant vertebrate protein. It’s fibrils are very strong and can support considerable weight without breaking. The strength results from the triple helical structure much like that of a rope. Collagen has a distinctive amino acid composition and repeating sequence of Gly-X-Y where X is often Pro and Y is often modified Pro, 4-hydroxyproline (Hyp). This sequence is required for the tight packing in the triple helix. 69 Collagen Triple Helix Hydroxyproline stabilizes the triple helix. If the enzyme that makes hydroxyproline, prolyl hydroxylase, is inhibited, collagen is much less stable becoming denatured at 24o C rather than 39o C. 6/17/2024 70 Modifications of amino acids in collagen Synthetic polyproline polypeptides spontaneously fold into a helix, polyproline II helix, resembling the helices observed in collagen. There is an absolute requirement for a glycine every third residue to allow for efficient packing in the triple helix interior. 6/17/2024 71 Why college students should eat their fresh fruits and vegetables Scurvy is caused by lack of vitamin C, or ascorbic acid (ascorbate). Vitamin C is required for, among other things, the hydroxylation of proline and lysine in collagen; scurvy is a deficiency disease characterized by general degeneration of connective tissue. Manifestations of advanced scurvy include numerous small hemorrhages caused by fragile blood vessels, tooth loss, poor wound healing and the reopening of old wounds, bone pain and degeneration, and eventually heart failure. Milder cases of vitamin C deficiency are accompanied by fatigue, irritability, and an increased severity of respiratory tract infections. Most animals make large amounts of vitamin C, converting glucose to ascorbate in four enzymatic steps. But in the course of evolution, humans and some other animals-gorillas, guinea pigs, and fruit bats-have lost the last enzyme in this pathway and must obtain ascorbate in their d 6/17/2024 72 Why college students should eat their fresh fruits and vegetables Collagen is constructed of the repeating tripeptide unit Gly-X-Y, where X and Y are generally Pro or 4-Hyp-the proline derivative (4R)- L- hydroxyproline, which plays an essential role in the folding of collagen and in maintaining its structure. The hydroxylation of specific Pro residues in procollagen, the precursor of collagen, requires the action of the enzyme prolyl 4-hydroxylase. 6/17/2024 73 Diseases Resulting from Improper Collagen Structure 1. Scurvy: Prolyl hydroxylase requires vitamin C. In vitamin C deficiency not enough hydroxyproline is formed resulting in weakened collagen fibrils. This affects skin, blood vessels and wound healing. 2. Lathyrism: The sweet pea plant contains an inhibitor of lysl oxidase which therefore inhibits collagen cross-linking. Loss of cross- linking affects bones, joints and blood vessels. 3. Familial disorders of collagen structure result from mutations in the collagen gene. Osteogenesis imperfecta, brittle bone disease, results from mutations at several positions in collagen genes. 4. Ehlers-Danlos syndrome, also caused by mutations in collagen genes, result in extreme hyperextensibility of joints and skin (rubber man). 6/17/2024 74 Silk Fibroin Fibroin, the protein of silk, is produced by insects and spiders. Its polypeptide chains are predominantly in the β conformation. Fibroin is rich in Ala and GIy residues, permitting a close packing of β sheets and an interlocking arrangement of R group 6/17/2024 75 Circular Dichroism Spectroscopy Circular dichrosim(CD) : is the difference in the absorption of left-handed circularly polarized light and right-handed circularly polarized and occurs when a molecule contains one or more chiral group In CD spectroscopy, the CD of a molecule is measured over a range of wavelength It’s a primary way of analyzing the secondary structure of proteins Since environmental conditions such as temperature and pH affects the secondary Structure of a protein CD spec can be employed to identify such changes Structural, kinetic and thermodynamic information about macromolecules can be derived from CD spectroscopy 6/17/2024 76 6/17/2024 77 6/17/2024 78 6/17/2024 79 6/17/2024 80 6/17/2024 81 6/17/2024 82 6/17/2024 83 6/17/2024 84 6/17/2024 85 6/17/2024 86 6/17/2024 87 6/17/2024 88 6/17/2024 89 6/17/2024 90 6/17/2024 91 6/17/2024 92 6/17/2024 93 6/17/2024 94 6/17/2024 95 6/17/2024 96 6/17/2024 97 6/17/2024 98 6/17/2024 99 6/17/2024 100 6/17/2024 101 6/17/2024 102 6/17/2024 103 6/17/2024 104 6/17/2024 105