Adverse Drug Interactions PDF
Document Details
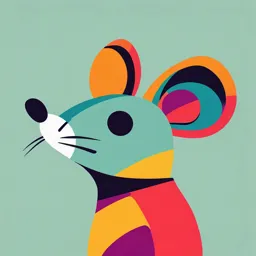
Uploaded by FeasibleTinWhistle
Tags
Summary
This document discusses adverse drug interactions, focusing on different types of toxicity and mechanisms. It explores on-target toxicity, where the drug impacts the same target as its intended effect, and hypersensitivity reactions. The document further touches on theories related to myotoxicity associated with statins.
Full Transcript
General Principle of Toxicology PCO 553 ADVERSE DRUG INTERACTIONS Page | 1 The first context of toxicity is on-target (or mechanism-based) toxicity. That is, the toxicity is due to inter...
General Principle of Toxicology PCO 553 ADVERSE DRUG INTERACTIONS Page | 1 The first context of toxicity is on-target (or mechanism-based) toxicity. That is, the toxicity is due to interaction of the drug with the same target that produces the desired pharmacological response. The concept is not one of competitive inhibition but rather that the biological response that the drug exhibits upon binding to its target is the same one that produces both the therapeutic and the toxic effects. In principle this type of toxicity is difficult to deal with because all classes of compounds developed to treat the disease will show the toxicity. Changing the target for the disease may be necessary. However, another strategy is exemplified in the case of statins. All statins produce hypercholesterolemic properties by inhibiting 3-hydroxy-3-methylglutaryl CoA (HMG CoA) reductase in the liver, i.e. the target. The adverse effects of statins are also due to inhibition of HMGCoA reductase in muscle and possibly other tissues, i.e. geranylgeranylation of proteins is inhibited. Fortunately the distribution of statins between tissues can be modulated by various transport proteins, and although on-target toxicity is an issue it can be controlled by inter- tissue distribution. Proposed Mechanisms of Myotoxicity The precise mechanisms underlying statin-associated myopathy are not well understood; however, theories do exist. One proposed mechanism suggests that decreased cholesterol concentrations secondary to statin therapy may result in alterations in myocyte membrane cholesterol. A second proposed mechanism involves depletion of isoprenoids that control myofiber apoptosis, and a third mechanism suggests that a depletion of ubiquinone or coenzyme Q10 (CoQ10) may account for the potential myotoxicity of statins. Reduced Sarcolemmal Cholesterol: It is proposed that because cholesterol plays a key role in cell membrane fluidity, lowering cholesterol with a statin may possibly disturb the integrity of the myocyte and lead to membrane destabilization. This theory is unlikely to be valid because it has been demonstrated in experimental models that nonstatin lipid-lowering agents, including fibrates, have induced myopathy through different pathways. Additionally, a second finding demonstrates that inherited disorders of the distal cholesterol synthetic pathway result in reduced cholesterol levels without occurrence of associated myopathy. Isoprenoid Depletion: Isoprenoids are lipids that are by-products of the HMG-CoA reductase pathway and play a key role in the control of myofiber apoptosis or cell death. Isoprenoids are linked to proteins by a process called farnesylation or geranyl generation. A reduction in these processes is thought to increase concentrations of cytosolic calcium, which activates a cascade leading to cell death. 3,5 In addition, it has been demonstrated that statin-induced apoptosis in the smooth muscle cells is prevented by exogenous supplementation of isoprenoids, with the two most important isoprenoids for the pathway being farnesyl pyrophosphate and geranyl pyrophosphate. The positive results from isoprenoid supplementation provide support to the role of isoprenoids in statin-associated myopathy. Inhibition of CoQ10 or Ubiquinone: Ubiquinone or CoQ10 is a product of the HMG-CoA reductase pathway. This coenzyme is an isoprenoid that plays a key role in the electron transport chain, and reduction in CoQ10 could cause abnormal mitochondrial respiratory function, leading to impaired energy production and induce myopathy. The second context of drug toxicity is hypersensitivity and immune responses. For instance, Page | 2 allergic reactions to penicillins have been recognized for many years. The concept, posits that drugs (or their metabolites) react with proteins in the body (as haptens) to induce antibodies and immune responses. The concept avers that the chemical is not completely stable and has the potential to bind covalently to proteins and initiate antibody production example penicillins. The term hapten (half antigen) defines low molecular weight chemical reagents which, as such, will not induce immune responses. However, immune responses to haptenated macromolecular carriers (proteins) lead to the production of hapten-specific antibodies and T lymphocytes. Even though free hapten fails to induce antibody production, hapten-reactive antibodies bind to free hapten as well as to carrier-coupled hapten. The free hapten molecules, therefore, compete for antibody bound to hapten-carrier conjugates. Once bound to protein carriers, haptens form antigenic determinants that may activate the antigen receptors of B lymphocytes as well as of T lymphocytes. Haptens, such as dinitrophenol, are small chemicals that can be bound by specific antibodies but are not immunogenic by themselves. If, however, haptens are coupled to proteins, which serve as carriers, the conjugates are able to induce antibody responses against the haptens. There are three important characteristics of anti-hapten antibody responses to hapten-protein conjugates POI The Hapten Hypothesis. Landsteiner et.al (1935) reported an inability to induce an immune response to small molecules unless the molecules were chemically reactive and bound to protein – thus the trajectory of the hapten hypothesis: Small molecules are not immunogenic, but if they bind irreversibly to protein, the modified protein can induce an immune response (FIG ). The small molecule that binds to protein leading to an immune response is referred to as a hapten. Penicillin-induced allergic reaction exemplifies the hapten theory of drug toxicity. Studies showed that the β -lactam ring of penicillin reacts irreversibly with free amino and sulfhydryl groups on proteins. In some patients, this leads to an immune response against the penicillin–protein adduct, and if the antibody response generates sufficient IgE antibodies, a severe allergic reaction such as anaphylaxis ensues. On the basis of this, a test for penicillin allergy was developed, in which penicillin is bound to a polymer of lysine, which has free amino groups, and when small amounts of this material, referred to as the major determinant, are injected into the skin, it causes degranulation of mast cells leading to a local wheal and flare response. There are also breakdown products of penicillin that can covalently bind to proteins and lead to an immune response, and these adducts are referred to as minor determinants. The mechanism of penicillin-induced allergic reactions fits the hapten hypothesis where the chemical reactivity of the penicillin allows it to act as a hapten. Reactive Metabolites - A significant influence on the field of DT was the finding by the Millers that a common mechanism by which chemicals cause cancer involves the formation of chemically reactive metabolites that bind to DNA and lead to mutations. An example is the acetaminophen-induced hepatotoxicity. Studies showed that acetaminophen-induced hepatotoxicity is caused by a reactive imidoquinone metabolit. Most of this reactive metabolite is detoxified by reaction with glutathione, and significant toxicity does not occur until liver glutathione is substantially depleted. Another example is Page | 3 halothane hepatotoxicity. Halothane is oxidized by cytochrome P450 to the reactive trifluoroacetyl chloride. Patients who develop halothane-induced hepatotoxicity have antibodies against trifluoroacetyl-modified proteins and this implies that modification of protein by the reactive metabolite has led to an immune response. There are also examples of DT in which there are antibodies against the cytochrome P450 that is responsible for forming a reactive metabolite of the drug. Examples include tienilic acid-induced hepatotoxicity where the reactive metabolite is formed by Cyp2C9 (8) and dihydralazine- induced hepatotoxicity where the reactive metabolite is formed by Cyp1A2 (12). Presumably, this is also due to reactive metabolites acting as haptens and in this case, the result is antibodies directed primarily against the protein portion of the hapten–protein complex to produce autoantibodies. The third context of drug toxicity is off-target toxicity. The issue here is that the drug is not specific in its interactions. Binding to an alternate target is the cause of toxicity. With our current knowledge of the complexity of biological regulatory pathways and multi-gene families (e.g. protein kinases), it is not surprising that a drug might not be totally specific. The example in TAB is terfenadine, which binds not only to the H1 receptor (eliciting the desired antihistaminic response) but also to the hERG channel and thus causing arrhythmias. In principle, this liability can be addressed by more screening and development of drug candidates with lower IC 50 and Kd values, in that a lower dose might avoid the specificity issue. This ion channel (sometimes simply denoted as 'hERG') is best known for its contribution to the electrical activity of the heart: the hERG channel mediates the repolarizing IKr current in the cardiac action potential, which helps coordinate the heart's beating (SEE DIAGAM) The fourth context of drug toxicity is bioactivation. Many drugs are converted to reactive products (often termed (reactive) “metabolites”). These entities modify the proteins they react with and somehow cause toxicity, although mechanisms have been evasive (vide infra). One theory is that important regulatory or other proteins are modified, with loss of function. Another possibility is that the modified proteins induce immune responses, linking with the second context of toxicity BIOACTIVATION Q. Describe briefly bioactivation. Formation of harmful or highly reactive metabolic from relatively inert/ nontoxic chemical compounds is called bioactivation or toxication. The bioactive metabolites often interact with the body tissues to precipitate one or more forms of toxicities such as carcinogenesis, teratogenesis, and tissue necrosis. The bioactivation reactions are generally catalyzed by cytochrome P450-dependent monooxygenase systems, but some other enzymes like those in intestinal flora are also involved in some cases. The reactive metabolites primarily belong to three main categories—electrophiles, free radicals, and nucleophiles. The Page | 4 formation of electrophiles and free radicals from relatively harmless substances/xenobiotics account for most toxicities. Q. Define electrophiles. Electrophiles are molecules which are deficient in electrons pair with a positive charge that allows them to react by sharing electron pairs with electron-rich atoms in nucleophiles. Important electrophiles are epoxides, hydroxyamines, nitroso and azoxy derivatives, nitrenium ions, and elemental sulfur. These eletrophiles form covalent binding to nucleophilic tissue components such as macromolecules (proteins, nucleic acids, and lipids) or low molecular weight cellular constituents to precipitate toxicity. Covalent binding to DNA is responsible for carcinogenicity and tumor formation. Q. Define free radicals. Free radicals are molecules which contain one or more unpaired electrons (odd number of electrons) in their outer orbit. Q. Define nucleophiles. Nucleophiles are molecules with electron-rich atoms. Formation of nucleophiles is a relatively uncommon mechanism for toxicants. Examples of toxicity induced through nucleophiles include formation of cyanides fro amygdalin, acrylonitrile, and sodium nitroprusside and generation of carbon monoxide from dihalomethane. Bioactivation Extensive reversible binding of drugs to plasma and tissue proteins has been well documented, and this type of binding is considered to be a critical factor influencing hepatic and renal disposition and efficacy of drugs However, the covalent binding of drugs to proteins also occurs, giving rise to the formation of drug- protein adducts, which has long been associated with drug toxicity. Previous studies had reported that chemical carcinogens,(e.g., p-dimethylaminoazobenzene) were biotransformed to electrophilic metabolites that covalently bound macromolecules, including DNA and proteins. Covalent binding of acetaminophen (APAP) metabolite to hepatic proteins was closely associated with the hepatotoxicity of APAP. In the last 20 years, a large body of data concerning the formation of protein adducts and its relevance to toxicity has emerged , which has initially established an association between protein adducts and toxicity of drug. The drugs giving rise to protein adducts are always nontoxic per se, but undergo enzyme-mediated bioactivation to chemically reactive metabolic intermediates (MIs). Theoretically, all Phase I and Phase II drug-metabolizing enzymes can bioactivate drugs, resulting in MIs that may render nucleophilic attack on these enzymes and/or other important cellular proteins. Thus, cellular functions may be disrupted, leading to cell death or apoptosis. More importantly, the protein adducts may be recognized by immune effector cells as nonself or neoantigens and thereby trigger immune responses against the drug-hapten, the carrier protein, or both. These immune responses may lead to cytokine release, autoantibodies, or mild to severe immune-mediated tissue/organ injuries. Page | 5 APAP adducts When used at therapeutic doses, APAP is mainly detoxified by glucuronidation (52 –57% total urinary metabolites) and sulfation (30 –44%), giving rise to glucuronides and sulfates excreted into the urine. In vitro studies indicated that most UGTs catalyzed APAP glucuronidation, but UGT1A1, 1A6, and 1A9 were most active. Acetaminophen also undergoes oxidation (