Physio 4 Notes - Sensory Systems PDF
Document Details
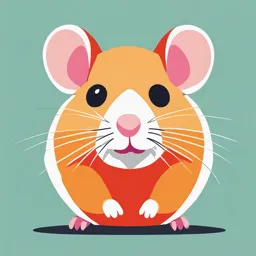
Uploaded by LuminousDoppelganger6400
Nadia Aldaher
Tags
Summary
These notes from Nadia Aldaher cover sensory systems, including vision, hearing, and vestibular systems. The document details the mechanisms of sensory reception and processes.
Full Transcript
Nadia Aldaher Physio 4 Notes Sensory Systems Nadia Aldaher Summarizing the Senses Sense Reception Receptor Trigger Highest Depolarization Specialized cells /...
Nadia Aldaher Physio 4 Notes Sensory Systems Nadia Aldaher Summarizing the Senses Sense Reception Receptor Trigger Highest Depolarization Specialized cells / type Cell center Mechanism Membranes Vision Secondary Rods and Light Stimuli Occipital Lobe In darkness: On/Off bipolar cells Cones Continuous Na+ influx Produces Cuneus (lower (voltage gated) Depolarization Receptor Produces visual field) seperate Depolarizatio and Lingual On/Off Ganglionic Rhodopsin remains from nerve n Gyrus (higher cell visual field) cGMP → Produces Action Potential Neurotransmitter realease Hearing Secondary Hair cells Lower pressure Superior K+ influx via Outer vs Inner hair in Scala Temporal mechanosensitive cells Produces vestibuli than Gyrus channels Receptor Scala Tympani Outer hair cells seperate Depolarizati due to negative connected to from nerve on Stapes moves membrane potential tectorial membrane out of oval compared to Endolymph window Ca+ influx due to voltage gated channels after depolarization → Neurotransmitter release Vestibular Secondary Hair cells Rotational Cerebral K+ influx via (Semicircular) in AMPULLA acceleration Cortex mechanosensitive Hair cell’s apical (Angular) channels surface covered by Produces Middle Capula Receptor Depolarizatio temporal Gyrus due to negative seperate from n membrane potential nerve compared to Endolymph Ca+ influx due to voltage gated channels after depolarization → Neurotransmitter release Nadia Aldaher Sense Reception Receptor Trigger Highest Depolarization Specialized cells / type Cell center Mechanism Membranes Vestibular Secondary Hair cells Linear Cerebral K+ influx via (Vestibulum) acceleration Cortex mechanosensitive Hair cell’s apical Receptor Produces (vertical and channels surface covered by seperate from Depolarizatio horizontal) Middle Otolithic nerve n temporal due to negative membrane VERTICAL: Gyrus membrane potential in UTRICLE compared to Saccule and Endolymph receptors SACCULE HORIZON Ca+ influx due to TAL: voltage gated channels Utricle after depolarization receptors → Neurotransmitter release Pain Primary Free nerve Mechanical Postcentral Na+ influx via Nerve fibers: (Nociceptive) endings pressure Gyrus mechanosensitive channels A-delta fibers: For Receptor in Produces Temperature primary pain nerve Action 1. Myelinated Potential Chemical 2. Fast 3. Small receptive fields 4. Dull pain 5. Good localization C fibers: For secondary pain 1. Unmyelinate d 2. Slow 3. Large receptive fields 4. Sharp pain 5. Poor localization Nadia Aldaher Sense Reception Receptor Trigger Highest Depolarization Specialized cells / type Cell center Mechanism Membranes Pain Primary Free nerve Mechanical Postcentral Na+ influx via Nerve fibers: (Antinociceptiv endings trigger Gyrus mechanosensitive e) channels C fibers: Receptor in Produces Peripheral nerve Action pathway Potential A-beta fibers Touch-pressure pathway Taste Secondary Modified Chemical Insula Salty: Salty: epithelial cells trigger Triggered by Na+ (Cerebral cortex) - Na influx Produces Ca+ influx due to voltage Sour: Depolarizatio gated channels after Triggered by H+ Receptor n depolarization → seperate from Neurotransmitter release Bitter: nerve Triggered by K+, Sour: Mg+, Alkaloids and Long-chain nitrogen - H+ influx containing substances - K+ outflux inhibition Sweet: Triggered by Sugars, Ca+ influx due to alcohols, amino acods voltage gated channels after depolarization → Umami: Neurotransmitter Triggered by release L-glutamate Bitter, Sweet & Umami: - G-protein stimulation → Phospholipase C activation → PIP2 → IP3 + DAG Ca+ influx from sarcoplasmic reticulum due to 2nd messenger generation → Neurotransmitter Nadia Aldaher release Smell Primary Modified Chemical Uncus G-protein stimulation nerve cells trigger Adenylyl cyclase → in Olfactory cAMP generation epithelium - Ca2+ influx Receptor in Produces - Na+ influx Action nerve - Cl- outflux Potential Nadia Aldaher Part 1 - General about Sensory Systems Lecture 3 Components of Sensory Systems Peripheral part Receptor + supplementary structures (such as hair on hair cells) Conductive part Conductive pathway from receptor to CNS Central part Analyzes information and creates the sense that we percieve Sensory Modality Principal type of sensation Qualitative Examples: Different tastes (sweet, salty, sour etc) Parameters Different colours Qualitative parameters are parameters we can’t measure between each other, for example, we can’t measure sweet compared to salt. They are two distinguishing things. Quantiative Example: Strength of stimuli applied Parameters Senses Conscious Sense Unconscious Sense Highest centers in Cerebral Cortex Highest centers Subcortically Senses we consciously perceive Senses we do not consciously percieve Example: Example: Hearing Chemoreceptors Pain Baroreceptors Temperature We do not know consciously how much CO2 is in our Touch blood, but our body does via baroreceptors Pressure Taste Smell Nadia Aldaher Absolute vs Discriminative Threshold Absolute Threshold Discriminative Threshold A Threshold stimulus: The absolute minimum Minimal difference between two stimuli that stimuli applied in order to activate a certain could be perceived as two. sense (Touch, pain, vision etc) 3 Types: Examples: 1. Spatial Discriminative Threshold Lowest concentration of a substance to Minimal distance between two equally strong trigger a taste receptor. (0.0001 grams of stimuli that can be perceived as two. sugar in a glass of water may not reach our threshold but 10^-4 mol/L will.) Examples: Most quiet noise we could perceive with The distance between 2 points that our ears (noise that reaches a certain dB touches our skin that we can perceive as 2 value) distinct points (when we poke our skin with 2 pens, if these pens are only 0.5 cm apart, we will not be able to distinguish them as 2 different pokes, and the discriminative threshold won’t be reached. But if the pens are 10 cm apart, we can easily distinguish them as 2 pokes reaching the discriminative threshold) 2. Temporal Discriminative Threshold Minimal time between two equally strong stimuli that can be perceived as two. Examples: FPS (frames per second). Amount of time that passes between two images that we can distinguish as two. (We can distinguish frames appearing on a screen if they appear 1 second apart from one another but not if they appear 0.01 seconds apart from one another.) 3. Intensity Discriminative Threshold Minimal intensity between two stimuli that can be perceived as two. Examples: If we carry a 100 gram bag, we won’t be able to notice added weight to that bag until a certain point (usually 30% increase) Nadia Aldaher Classification of Receptors Due to Environment Exteroreceptors (percieve stimuli from external environment): Touch from where stimuli are Pressure perceived Taste Smell Vision Interoreceptors (percieve stimuli from internal environment): Baroreceptors Chemoreceptors Proprioreceptors (special type of interoreceptor) Receptors in locomotor system Due to Relations with Contact receptors (Contacts the stimuli directly) Touch Stimuli Pressure Chemoreceptors Distant receptors (No direct contact with source of stimuli) Hearing (sound waves from distant sources) Vision (light from distant sources) Due to Energy Type Chemoreceptors (perceive stimuli of chemical nature) Taste Smell Mechanoreceptors (percieve stimuli due to stretch or touch) Touch Pain Due to Adaptation Phasic Fast adaptive receptors (Adapting - stop sending impulses to CNS if stimuli is constant) Tonic Slow adaptive receptors (Slow or Incomplete Adaptation - still sends impulses to CNS even when stimuli is constant) Example: Muscle spindle Nadia Aldaher Primary vs Secondary Reception Type of Reception Description Senses Primary Reception Receptor is a nerve cell derivative Touch and CAN generate Action Pressure Potential directly Pain Temperature Smell Interoreception Exteroreception Secondary Receptor is a specialized cell and Vision can NOT generate Action Hearing Reception Potential directly. Equilibrium sense Neurotransmitter release is (Vestibular System) necessary. Taste Synapses between receptor cell and nerve cell are included. Coding of Stimulus Type Property of Stimuli Explanation Type of Stimulus Different types of stimuli will lead to different types of sense-activation Examples: Taste receptors → Chemical stimuli on tongue Visual receptors → Photons on photoreceptors Touch receptors → Pressure applied on skin Intensity of Stimulus ALL-OR-NONE LAW Intensity is coded by: 1. FREQUENCY OF ACTION POTENTIALS 2. NUMBER OF NERVE FIBER ACTIVATION Nadia Aldaher Duration of Stimulus Phasic vs Tonic receptors, the duration of impulses from receptor to CNS Localization of Stimulus Specific sensory receptors are placed on body for us to localize the sense. Lateral Inhibition Precise localization of stimulus is perceived due to lateral inhibition (inhibition of weakly stimulated areas in order to pay more attention to stronger stimulated area) Without Lateral Inhibition With Lateral Inhibition Central nerve fibers: Fire high frequency signals to 1. If central neuron activates strongly strong CNS activation of inhibitory neuron 2. This will inhibit lateral sensory units and Peripheral nerve fibers: Fire low frequency signals stopping weak impulse transmission from to CNS them 3. Lateral sensory units activate with low Due to Peripheral nerve fibers firing it “hides” the frequency of impulses and might not high firing sensation of central nerve fibers, causing reach threshold for inhibitory neuron us to lose localization of the source of the stimuli. activation no inhibition of the centrally placed sensory units 4. Lateral inhibition causes suppression of weak impulse transmission, while not affecting strong impulse transmission and thus makes sharp borders of sensation Nadia Aldaher Touch Sensory System Highest center: Postcentral Gyrus Touch System Receptors Receptive Field Size Small Large Adaptation Fast Meissner’s Corpuscle Pacinian Corpuscle Slow Merkel’s Disk Ruffini’s endings Two-point Discrimination Test (Spatial Discriminative threshold): Twos stimuli with equal intensity are applied on the skin, the least distance between the two stimuli is determined at which the person is able to recognize two stimuli Temperature Sensory System Highest center: Postcentral Gyrus Cold Receptors Warmth Receptors More active if T° < 40 °C Increasing firing rate if T° > 30 °C Maximal firing rate 25-27 °C Maximal firing rate at about 44-45 °C At 37 °C (natural core temperature of the body) cold and warmth receptors fire equally Visceral Sensation Can be of different types which are activated due to Stretch receptors Thermoreceptors Chemoreceptors Osmoreceptors Pain receptors Nadia Aldaher Part 2 - Vision Lecture 4 Sense Reception Receptor Trigger Highest Depolarization Specialized cells / type Cell center Mechanism Membranes Vision Secondary Rods and Light Stimuli Occipital Lobe In darkness: On/Off bipolar cells Cones Continuous Na+ influx Produces Cuneus (lower (voltage gated) Depolarization Receptor Produces visual field) seperate Depolarizatio and Lingual On/Off Ganglionic Rhodopsin remains from nerve n Gyrus (higher cell visual field) cGMP → Produces Action Potential Neurotransmitter realease Transparent structures and Refractive Index Structure Refractive index from most to least refractive index Lens 1.40 Cornea 1.38 Vitreous Humor 1.34 Aqueous Humor 1.33 Air 1 Refraction occurs most at Cornea due to sharp change between air touching the eye Pupillary Reactions to Light Pupillary reflex components: - Pretectal nucleus - Edinger-westphal nucleus - III cranial nerve - Ciliary ganglion - Constrictive pupillary muscle Nadia Aldaher Damages to the Eye Normal eye Lateral side of vision fields for both eyes: Cross at Optic Chiasm - Right lateral view goes through Optic Chiasm to Left optic tract - Left lateral view goes through Optic Chiasm to Right optic tract Damage to the Right Optic Nerve Affected: - Medial field of Right eye - Lateral field of Right eye Damage to the Left Optic Nerve Affected: - Medial field of Left eye - Lateral field of Left eye Nadia Aldaher Damage to the Optic Chiasm Affected: - Lateral field of Right eye - Lateral field of Left eye Damage to the Right Optic Tract Affected: - Medial field of Right eye - Lateral field of Left eye Damage to the Left Optic Tract Affected: - Medial field of Left eye - Lateral field of Right eye Nadia Aldaher Accommodation Reflex Ciliary Suspensory Lens Pupil Pupil Pupil Edinger-We Muscle Ligaments Sphincter Dilator stphal Flatter = Muscle Muscle nucleus Controls Less Controls lens refractive Constricts III cranial lens power pupil Dilates pupil nerve Constriction Constriction = Flatter Rounder Ciliary = Rounder Lens = More Ganglion Lens Relaxation refractive Relaxation = = Rounder power All pupillary Flatter Lens Lens light reflex components If Object is Relaxes Contracts Flatter Dilates Relaxes Contracts Inactivated Far Away In order to In order to Less Less Constricts Dilates pupil make lens make lens refractive focused pupil flatter flatter power pupil If Object is Contracts Relaxes Rounder Constric Contracts Relaxes Activated Close In order to In order to Rounder = ts More focused Less focused make lens make lens More More pupil pupil rounder rounder refractive focused power pupil Due to Acetylcholi ne binding to M3 receptors Refraction Anomalies Name Image Description Far Point Near Correction Vision Point Vision Nadia Aldaher Emmetropia Normal shape of eye Infinite 10-12 cm N/A and lens (Normal sight) Normal function of ciliary muscle contraction Myopia Eye is elongated 1 meter 5-6 cm Concave lens Near Sight (better than (opposite shape of Higher Refractive ememtropic oblong to fight power ) against the (the rounder / oblong less--flat eye) shape the eye is the more refraction it causes, A.k.a: compare a magnifying Minus lens glass to a normal flat Divering lens window) Hyperopia Eye is shorter Infinite 50 cm Convex lens Far sight “more squished” (opposite shape of shortness no fight against the Lower flatter eye) Refractive power (the shorter / squished A.k.a: shape the eye is the less Plus lens refraction it causes, Convering compare a magnifying lens glass to a normal flat window) Astigmatism Lens is more N/A N/A Cylindrical Uneven lens shape oval-shaped lens (mimicking the Blurry image shape of a normal lens to Light refraction is put focus on only 1 part of the uneven for every part eye-lens so the of the lens, some part rays aren’t split of visual field can be across the uneven affected surface) Some parts good refractive power, others too high or Nadia Aldaher too low Presbyopia N/A Ciliary muscle Infinite 1 meter Convex lens Loss of elasticity of contraction decreases nasally lens via ciliary muscle due to loss of elasticity Concave lens peripherally Usually due to old age Determination of Refraction Anomalies from Visus Tests Anomaly Visus for Near Vision test Visus for Distance Vision test Emmetropia 1 or above 1 or above Myopia 1 or above (usually above) Less than 1 Hyperopia Less than 1 1 or above Astigmatism Less than 1 Less than 1 Presbyopia Less than 1 1 or above Visus test Explanation Formula: The distance at which the test person is able to read a particular line The distance at which a normal emmetropic person can read the same line Visus value Less than 1 Worse than average vision Visus value More than 1 Better than average vision Visus value Equal to 1 Average vision Nadia Aldaher Example in Distance Visus test: - You are asked to read lines of symbols or letters from a poster at a set distance (d), for every new line the letters or symbols become increasingly smaller. T - The absolute smallest line you can read at your particular distance is what will be counted. The line has a value of “D” next to it, which corresponds to the distance a normal emmetropic person can properly read that particular line. - If you can read a line at 5 meters (d), and the “D” value of that line is 6 meters, that gives you a visus value of ⅚ = 0.833. Which is less than 1, thus you have a worse than average vision - If you can read a line at 5 meters (d), and the “D” value of that line is 4 meters, that gives you a visus value of 5/4 = 1.25. Which is more than 1, thus you have a better than average vision. - If you can read a line at 5 meters (d) and the “D” value of that line is 5 meters, that gives you a visus value of 5/5 = 1. Which is equal to 1, thus you have a better than average vision Circulation of Aqueous Humor, Introcular Pressure and Glaucoma 1. Ciliary body (secretes it) 2. Posterior chamber 3. Pupil (travels through it) 4. Anterior chamber 5. Canal of Schlemm (main draining area) Normal intraocular pressure: 10-22 mmHg Glacoma: Increased intraocular pressure, can be due to obstruction of Aqueous Humor drainage or decreased reabsoprtion Phototransduction in Photoreceptors Phototransduction in Phototransduction in Dark Conditions Light Conditions Rhodopsin Inactive Active (becomes Metharhodopsin) Turns 11-cis retinal into all-trans retinal Phosphodiesterase Inactive Active due to Metharhoropsin cGMP Untouched Cleaved into 5’GMP by Phosphodiesterase Ionic Transport Na+ and Ca2+ channels keep Na+ and Ca2+ channels closed due to functioning due to untouched lack of cGMP (since it’s been turned into cGMP 5’GMP) Nadia Aldaher Cell membrane potential positive Cell membrane more negative due to no even while Na+/K+ pump and Na+ or Ca2+ influx while K+ outflux K+ outflux. and Na+ outflux is still occurring. Cell activity Depolarization Hyperpolarization Neurotransmitter Released Inhibition of Release (Glutamate) Phototransduction in BIPOLAR OFF CELL Sign-Conserving Phototransduction in Phototransduction in Dark Conditions Light Conditions Neurotransmitter Recieves neurotransmitter Does NOT receive neurotransmitter Receptor Ionotropic (Activated) Ionotropic (Inactivated) Ionic Transport Na+ influx due to open No open Na+ channels ligand-gated Na+channels Cell activity Depolarization Hyperpolarization Phototransduction in BIPOLAR ON CELL Sign-Inverting Phototransduction in Phototransduction in Dark Conditions Light Conditions Neurotransmitter Recieves neurotransmitter Does NOT receive neurotransmitter Receptor Metabotropic (activated) Metabotropic (Inactivated) Ionic Transport Inhibition of Na+ channels Normal Na+ influx (due to metabotropic receptor activation) Cell activity Hyperpolarization Depolarization Nadia Aldaher Action potential produced later in the GANGLIONIC cell Receptive Fields Receptive Fields: Area of photoreceptors that are connected to one bipolar and ganglionar cell Receptive field Center: - Receptors in the center of a visual field directly connected to Ganglionar cell Receptive field Periphery: - Receptors around the center connected to Horizontal Bipolar cells (not connected to Ganglionar cell) Phototransduction in GANGLIONAR OFF CELL / OFF CENTER FIELD → React when there IS Neurotransmitter release (Dark) Light only in Light in entire Light only in Periphery Receptive Field Center (Peripheral (Full (Central Illumination) Illumination) Illumination) Frequency of HIGH LOW NONE Action Potentials Reason for Center Receptive Both Peripheral and Center Receptive Field directly Center field’s field’s Reaction Nadia Aldaher connected to neurotransmitter neurotransmitter Ganglionar cells production is halted. production is halted. If there’s darkness Still there are HOWEVER, in the center → some peripheral field’s More neurotransmitters neurotransmitter neurotransmitter released (minimal release is release → More amount) → Low continuous → As a action potentials action potential response Horizontal generated by generation from cell inhibits all ganglionar OFF ganglionar OFF receptors on that cells cells field, including center. Now center’s neurotransmitter production is not only halted it is also INHIBITED due to the Horizontal cell inhibitory activation. → No action potentials generated by ganglionar OFF cells Phototransduction in GANGLIONAR ON CELL / ON CENTER FIELD → React when there is NOT Neurotransmitter release (Dark) Light only in Light in entire Light only in Periphery Receptive Field Center (Peripheral (Full (Central Illumination) Illumination) Illumination) Nadia Aldaher Frequency of NONE LOW HIGH Action Potentials Reason for Center Receptive Both Peripheral and Center Receptive Field directly Center field’s field’s Reaction connected to neurotransmitter neurotransmitter Ganglionar cells production is halted. production is halted. If there’s darkness Still there are HOWEVER, in the center → some peripheral field’s More neurotransmitters neurotransmitter neurotransmitter released (minimal release is release → No amount) → Low continuous → As a action potentials action potential response Horizontal generated by generation from cell inhibits all ganglionar ON ganglionar ON receptors on that cells cells field, including center. Now center’s neurotransmitter production is not only halted it is also INHIBITED due to the Horizontal cell inhibitory activation. → High action potential Nadia Aldaher generation from ganglionar ON cells Types of Ganlgionar cells: 1. P (Parvocellular): Small receptive fields 2. M (Magnocellular): Large receptive fields 3. K (Koniocellular): For color vision 4. Photosensitive: For circadian rhythms Darkness Adaptation: 20-30 minutes Light Adaptation: 5-10 minutes Color vision. Types of Cones and their Light absorption Spectra Cone Spectrum (Colour) Pathology / Disturbance S cone (Short length) 430 nm (blue) Tritanopia (Lack of S cones) M cone (Medium length) 530 nm (green) Deuteranopia (Lack of M cones) L cone (Long length) 560 nm (red) Protanopia (Lack of L cones) Visual Fields Medially and Upward 60° Downwards 70° Laterally (Sides) 90° Signals from lower visual field comes to: Cuneus Signals from higher visual field comes to: Lingual Gyrus Signals from right eye: Goes to left Cuneus or Lingual Gyrus (depending on if it’s high or low) Signals from left eye: Goes to right Cuneus or Lingual Gyrus (depending on if it’s high or low) Examples: Signals from low visual fields via right eye → To left Cuneus Signals from high visual fields via left eye → To right Lingual Gyrus Nadia Aldaher Part 3 - Hearing and Vestibular System Lecture 5 Sense Reception Receptor Trigger Highest Depolarization Specialized cells / type Cell center Mechanism Membranes Hearing Secondary Hair cells Lower pressure Superior K+ influx via Outer vs Inner hair in Scala Temporal mechanosensitive cells Produces vestibuli than Gyrus channels Receptor Scala Tympani Outer hair cells seperate Depolarizati due to negative connected to from nerve on Stapes moves membrane potential tectorial membrane out of oval compared to Endolymph window Ca+ influx due to voltage gated channels after depolarization → Neurotransmitter release Vestibular Secondary Hair cells Rotational Cerebral K+ influx via (Semicircular) in AMPULLA acceleration Cortex mechanosensitive Hair cell’s apical (Angular) channels surface covered by Produces Middle Capula Receptor Depolarizatio temporal Gyrus due to negative seperate from n membrane potential nerve compared to Endolymph Ca+ influx due to voltage gated channels after depolarization → Neurotransmitter release Vestibular Secondary Hair cells Linear Cerebral K+ influx via (Vestibulum) acceleration Cortex mechanosensitive Hair cell’s apical Receptor Produces (vertical and channels surface covered by seperate from Depolarizatio horizontal) Middle Otolithic nerve n temporal due to negative membrane VERTICAL: Gyrus membrane potential in UTRICLE compared to Saccule and Nadia Aldaher SACCULE receptors Endolymph HORIZON Ca+ influx due to TAL: voltage gated channels Utricle after depolarization receptors → Neurotransmitter release Characteristics of Sound waves Frequency → Pitch Human range: 16-20 000 Hz Amplitude → Loudness Human range: 0-120 dB Normal hearing Threshold: Air conduction: 0-20 dB Bone conduction: 0-20 dB Air and Bone Conduction Air Conduction Bone Conduction Sound waves conducted to the external and middle Sound waves conducted directly into the inner ear ear via vibrations (skipping external and middle ear) Audiometry. Conduction and Neural Deafness Sensorineural Hearing Loss Conductive Hearing Loss Air conduction: OVER 20 dB Air conduction: OVER 20 dB Bone conduction: OVER 20 dB Bone conduction: 0-20 dB (normal) Air conduction < Bone Conduction means that there is conductive hearing loss Due to sound waves not being processed Due to sound waves not reaching the inner well in the inner ear or further ear Nadia Aldaher Audiometry - Rinne’s Test Compares air and bone conduction by placing a tuning fork on the mastoid bone first, then once the noise isn’t hear anymore, placing it above the ear in the air (you should hear the noise return once it’s placed in the air since air conduction is better perceived than bone conduction) The ONLY thing we can test in Rinne’s method is CONDUCTIVE HAERING LOSS (Not sensorineural hearing loss) Results and conclusions from Rinne’s Test If person HEARS noise return after the tuning Normal Air conduction in that ear (person has fork is placed in the air next to the hear better Air conduction than bone conduction which is normal) Air conduction > Bone conduction No Conductive Hearing Loss in that ear If person does NOT HEAR noise return after the Abornal conduction in that ear (person has worse or tuning fork is placed in the air next to the hear similar Air conduction than bone conduction) Air conduction < Bone conduction Conductive Hearing Loss in that ear Nadia Aldaher Audiometry - Weber’s Test Compares air and bone conduction by placing a tuning fork on the forehead (you should hear the noise be conducted EQUALLY on both ears) We can test BOTH Sensorineural loss and Conductive Hearing loss in Weber’s test, but we can NOT distinguish between them without applying Rinne’s test too. Results and conclusions from Weber’s Test If person hears noise EQUALLY from both ears. Normal Air conduction and Bone conduction in both ears Air conduction > Bone conduction No Conductive Hearing Loss or Sensorineural Hearing Loss If person does NOT hear noise equally from both TWO possible Conclusions (only one is true): ears / One ear perceives noise better than the other 1. Sensorineural loss in ear that hears noise WORSE Sound lateralization in one ear over the other 2. Conductive hearing loss in ear that hears noise BETTER Reason for Sensorineural hearing loss in ear that hears noise WORSE: Neural issue or damage in that ear makes it harder to process noise, thus causing that ear to perceive noise worse Reason for Conductive hearing loss in in ear that hears noise BETTER: Due to air conduction being the most prevalent hearing method for humans, once there are issues with conduction of air sounds to that ear, the hair cells in the ear becomes more sensitive to bone conductive sounds, making us perceive bone conductive sounds better than the other ear, even though that ear is technically suffering from conductive hearing loss. Nadia Aldaher If Weber’s test is negative (we experience sound lateralization in one ear over the other), the two possible conclusions can not be distinguished until we perform Rinne’s test on the ear that hears the noise in Weber’s test better (if lateralization in left ear, we perform Rinne’s test on left ear). We need a combination of both Weber’s and Rinne’s test. If person passes Rinne’s test, we know that the Conductive hearing loss conclusion is proven false, thus we can make the conclusion that it’s Sensorineural hearing loss in the opposite ear. If person does NOT pass Rinne’s test, we know that the Conductive hearing loss conclusion is proven correct, and we can dismiss the Sensorineural hearing loss hypothesis Examples of Audiometry Test results: Test Results Conclusion Weber’s test No Conductive Hearing Loss No lateralization (both ears perceive noise the same amount) No Sensorineural Hearing Loss Rinne’s test: Higher air conduction over bone conduction on both ears Weber’s test Conductive Hearing Loss in Left ear Lateralization in Left ear (Left ear hears noise better than Right) (If we only used Weber’s test, another reason for left Rinne’s test: ear lateralization is Sensorineural hearing loss in Left ear tested: Worse or same air Right ear, but since person didn’t pass Rinne’s test on conduction over bone conduction (Air left ear, we can conclude that the person is suffering conduction < Bone conduction) from conductive hearing loss) (Rinne’s test not passed) Weber’s test Sensorineural Hearing Loss in Left ear Lateralization in Right ear (Right ear hears noise better than Left) (If we only used Weber’s test, another reason for right Rinne’s test: ear lateralization is Conductive hearing loss in Right ear tested: Higher air conduction Right ear, but since person DID pass Rinne’s test on over bone conduction on right ear (Air right ear, we can conclude that the person is NOT conduction > Bone conduction) suffering from conductive hearing loss so it has to be (Rinne’s test passed) sensorineural hearing loss) Nadia Aldaher Functions of the External Ear Sound wave collection Sound wave Transmission Resonance Defence function (earwax) Humidity and Temperature regulation of Tympanic Membrane Functions of the Middle Ear Sound Transmission Sound Amplification Defense Function Equilibration of Pressure with regards to the Tympanic Membrane (Tuba Auditiva) Sound Amplification in the Middle Ear 1. Membranes 17 Times greater surface area difference between Tympanic Membrane and Oval Window. Surface Area Difference between Tympanic Membrane Will amplify the sound 17 times. and Oval Window Membrane 2. Lever system 1.3 Times greater size difference between Malleus and Incus Size Difference between Will amplify the sound 1.3 times. Malleus-lever and Incus-lever RESULTING 22 times more amplified sound if compared to how it was in AMPLIFICATION the external ear (17 times from membrane size difference * 1.3 times from lever system = 22.1) Sound Transmission in Inner Ear - Structure of the Cochlea Image Description The Cochlea is shaped like a spiraling shell Nadia Aldaher Apex / Top of the Cochlea Helicotrema The top of the cochlea is where the shell protrudes the most, i.e. the center of the shell or the Helicotrema. More wide and loose Basilar Membrane Here membrane will vibrate more to LOW FREQUENCY SOUNDS Base / Bottom of the Cochlea The Oval window (where sound enters) and Round window (where sound exists) The bottom of the cochlea is where there are openings to the shell. Think of it as where a snail’s heard would protrude if this was a snail’s shell. More narrow and stiff Basilar Membrane Here membrane will vibrate more to HIGH FREQUENCY SOUNDS Coding of Sound Pitch At base of Cochlea: High Frequency sounds At apex of Cochlea: Low Frequency sounds Nadia Aldaher Sound Transmission in Inner Ear - Structure of the Cochlea Scala Vestibuli & Scala Media Scala Tympani PERILYMPH (Inner fluid) ENDOLYMPH (Inner fluid) Rich in Na+ ions Rich in K+ ions Poor in K+ ions This is where the Organ of Corti is located (Receptors for Hearing System) Secretion of Endolymph The Endolymph in the Scala Media has to be rich in K+ ions for the Reception of hearing to work. Hair cells release K+ ions into Perilymph K+ ions transported to Spiral ligament Fibrocytes in Spiral ligament take up K+ ions via: 1. Na+/K+ pump 2. Na+/K+/2Cl- symport K+ transported into intermediate cells via gap junctions Intermediate cells secrete K+ into Intrastrial fluid Marginal cells take up K+ in Intraastrial fluid via: 1. Na+/K+ pump 2. Na+/K+/2Cl- symport K+ diffuse into Endolymph Potential of Endolymph: +80 mV Nadia Aldaher Diseases affecting Endolymph (Decreased Endolymph Removal) Meniere’s disease Decreased Endolymph removal from inner ear Cause: Viral bacterial infection, Autoimmune reactions or Genetic conditions Symptoms: Vertigo, Sensorineural hearing loss, Tinnitus Sound Transmission THROUGH Inner Ear - Mechanism of Hearing When Stapes from Middle ear pushes When Stapes from Middle ear pushes against Oval window away from Oval window (Moves into Oval Window) (Moves out of Oval Window) Basilar Membrane Moves DOWN Basilar Membrane Moves UP Tecotrial Membrane Moves INWARDS Tectorial Membrane Moves OUTWARDS Hair Cell’s Stereocilia is bent towards Hair Cell’s Stereocilia is bent towards SHORTEST ONE LONGEST ONE Mechanosensitive K+ channels CLOSE Mechanosensitive K+ channels OPEN Influx of Ca2+ into Hair cell due to Voltage-gated channels Nadia Aldaher Neurotransmitter release Pressure in Scala Vestibuli > Pressure in Scala Pressure in Scala Vestibuli < Pressure in Scala Tympani Tympani Scala Vestibuli: Where sound enters Scala Vestibuli: Where sound enters Scala Tympani: Where sound exits Scala Tympani: Where sound exits Scala Media: In between Vestibuli and Tympani Scala Media: In between Vestibuli and Tympani When Stapes moves against Oval window, there will When Stapes moves against Oval window, there will be shock waves produced that will travel into the be shock waves produced that will travel into the Scala Vestibuli, thus making the pressure their larger Scala Vestibuli, thus making the pressure their larger than the one in the Scala Tympani. than the one in the Scala Tympani. Influx of K+ into Hair cells via Mechanosensitive K+ Channels Due to 2 Factors 1. Chemical Gradient Amount of K+ in Endolymph (that surrounds hair cell): 150 mM/L Emount of K+ in Hair cell: 120 mM/L 2. Electrical Gradient Endolymph charge: +80 mV Hair cell membrane potential: -70 mV Potential difference of 150 mV (Endococlear Potential) drives K+ into the cell If Hair cell’s hairs bend towards LONGEST If Hair cell’s hairs bend towards SHORTEST Hair Hair 1. Mechanosensitive K+ channels open 1. Mechanosensitive K+ channels are NOT 2. Depolarization of cell open 3. Voltage-gated Ca2+ channels open → 2. Hyperpolarization of cell due to K+ Ca2+ influx outflux to perilymph 4. Neurotransmitter release 3. Neurotransmitter release INHIBITED 5. Excitatory Postsynaptic Potential 4. No postsynaptic potential → NO Action generation potential in afferent nerve fiber 6. Action potential in afferent nerve fiber (NOT in hair cell) Impulse Conduction to Brain - Loudness & Time Delay From Right Ear Loudness Differentiation (via Lateral Superior Olivary (Sounds arrives to Right ear) Nucleus): Lateral Superior Olivary Nucleus in RIGHT ear: ACTIVATED Nadia Aldaher Lateral Superior Olivary Nucleus in LEFT ear: INACTIVATED Ipsilateral Effect Time Differentiation (via Medial Superior Olivary Nucleus): Medial Superior Olivary Nucleus in RIGHT ear: INACTIVATED Medial Superior Olivary Nucleus in LEFT ear: ACTIVATED Contralateral Effect From Left Ear Loudness Differentiation (via Lateral Superior Olivary (Sounds arrives to Left ear) Nucleus): Lateral Superior Olivary Nucleus in LEFT ear: ACTIVATED Lateral Superior Olivary Nucleus in RIGHT ear: INACTIVATED Ipsilateral Effect Time Differentiation (via Medial Superior Olivary Nucleus): Medial Superior Olivary Nucleus in LEFT ear: INACTIVATED Medial Superior Olivary Nucleus in RIGHT ear: ACTIVATED Contralateral Effect Vestibular Sensory System (Primary reception) Two types: 1. Vestibulum (for Linear Equilibrium Up/Down Side-to-Side) 2. Semicircular Canal (for Rotational Equilibrium) 1. Vestibulum (for Linear Equilibrium Up/Down Side-to-Side) Receptors Hair cell Receptor Location Types Utricle: For horizontal Equilibrium Contains Macula (sensory epithelium with Hair cells) Saccule: For vertical Equilibrium Contains Macula (sensory epithelium with Hair cells) Nadia Aldaher HORIZONTAL UTRICLE (Otolith organ) EQUILIBRIUM Senses Horizontal acceleration such as side-to-side or forward and backward VERITCAL SACCULE (Otolith organ) EQUILIBRIUM Senses Vertical acceleration such as Up and Down Apical Surface of Hair cells OTOLITHIC MEMBRANE (in Vestibulum) covered by: If we move forwards / Otolithic membrane displaced in opposite direction in backwards / sideways / Up / Utricle or Saccule Down Bending of stereocilia towards the longest hair → Opening of K+ mechanosensitive channels → Depolarization of Hair cell When we stop movement Otolithic membrane displaced in same direction as previous movement in Utricle or Saccule Stereocilia bent in opposite direction as before → → Closing of K+ mechanosensitive channels → Hyperpolarization of Hair cell Work the same as Hearing system, only instead of noticing pressure changes, the Utricle notices horizontal position of the head, the Saccule notices vertical position of the head causing K+ mechano-sensitive channels to open. 2. Semicircular Canal (for Rotational Equilibrium) Receptors Hair cell Receptor Location Types Crista: Specialized sensory structures within the Ampullae at the base of each semicircular canal Ampulla: Home for Crista, directly involved in sensing rotational equilibrium Apical Surface of Hair cells CAPULA MEMBRANE (in Semicircular Canal) covered by: First 15 seconds of Endolymph will move in the opposite side of rotation Rotation during the start of rotation: Nadia Aldaher Capula displaced in opposite direction of rotation Bending of stereocilia towards the longest hair → Opening of K+ mechanosensitive channels → Depolarization of Hair cell After 15 seconds of Endolymph will acquire the same rotational speed and Rotation direction as labyrinth Capula NOT deformed Stereocilia NOT bent After stopping Rotation Endolymph moves in same direction as previous rotation: Capula displaced in direction of previous rotation Stereocilia bent in opposite direction as before → → Closing of K+ mechanosensitive channels → Hyperpolarization of Hair cell Work the same as Hearing system, only instead of noticing pressure changes, the Crista and Ampulla notice angular and rotational position of the head causing K+ mechano-sensitive channels to open. Hair cell Depolarization and Hyperpolarization in Vestibular System If Hair cell’s hairs bend towards LONGEST If Hair cell’s hairs bend towards SHORTEST Hair Hair 7. Mechanosensitive K+ channels open 5. Mechanosensitive K+ channels are NOT 8. Depolarization of cell open 9. Voltage-gated Ca2+ channels open → 6. Hyperpolarization of cell due to K+ Ca2+ influx outflux to perilymph 10. Neurotransmitter release 7. Neurotransmitter release INHIBITED 11. Excitatory Postsynaptic Potential 8. No postsynaptic potential → NO Action generation potential in afferent nerve fiber 12. Action potential in afferent nerve fiber (NOT in hair cell) Electrical Gradient in Vestibular System for K+ influx: Endolymph charge: +80mV Hair cell membrane potential: -40 mV Potential difference of 120 mV drives K+ into the cell Nadia Aldaher Type I and Type II Hair Cells in Vestibular System Type I Hair Cells Type II Hair Cells Better connection to afferent nerve fibers Less extensive connection to afferent nerve Are more influenced by the brain via fiber better connection to efferent nerve fibers Less influenced by the brain since efferent fiber connection is less extensive CONVERGENCE: Many Type II hair cells can converge into a single nerve fiber Vestibulo-Ocular Reflex - Mechanism of Reflex Reflex we have to keep our sight and eyes fixed on a point in the visual field while moving our head side-to-side If we move head to the RIGHT EYE MECHANISM: LEFT Right side’s (contralateral to the movement) oculomotor nerve (III) is INHIBITED → Medial rectus muscle is Both left and right eyes will move RELAXED towards the RIGHT. Right side’s (contralateral to the movement) abducens nerve (VI) is ACTIVATED → Lateral rectus muscle is CONTRACTED Why: When turning our head to the left, we want the right eye to move towards the RIGHT to keep our point of sight. We tighten muscle at the outer corner of the right eye (lateral rectus muscle) to force the eye towards the right, and relax muscle at the inner corner of the right eye (medial rectus muscle) so that the eye doesn’t move towards the left. LEFT EYE MECHANISM: Left side’s (ipsilateral to the movement) oculomotor nerve (III) is ACTIVATED → Medial rectus muscle is CONTRACTED Left side’s (ipsilateral to the movement) abducens nerve (VI) is INHIBITED → Lateral rectus muscle is RELAXED Why: When turning our head to the left, we want the left eye to move towards the RIGHT to keep our point of sight. We loosen muscle at the outer corner of the left eye (lateral rectus muscle) so the eye doesn’t move towards the left, and tighten muscle at the inner corner of the left eye (medial rectus muscle) so that the eye move towards the right. If we move head to the RIGHT EYE MECHANISM: RIGHT Right side’s (ipsilateral to the movement) oculomotor nerve (III) is ACTIVATED → Medial rectus muscle is Both left and right eyes will move CONTRACTED Nadia Aldaher towards the LEFT. Right side’s (ipsilateral to the movement) abducens nerve (VI) is INHIBITED → Lateral rectus muscle is RELAXED Why: When turning our head to the right, we want the right eye to move towards the LEFT to keep our point of sight. We loosen muscle at the outer corner of the right eye (lateral rectus muscle) so the eye doesn’t move towards the left, and tighten muscle at the inner corner of the right eye (medial rectus muscle) so that the eye is forced towards the left. LEFT EYE MECHANISM: Left side’s (contralateral to the movement) oculomotor nerve (III) is INHIBITED → Medial rectus muscle is RELAXED Left side’s (contralateral to the movement) abducens nerve (VI) is ACTIVATED → Lateral rectus muscle is CONTRACTED Why: When turning our head to the right, we want the left eye to move towards the LEFT to keep our point of sight. We tighten muscle at the outer corner of the left eye (lateral rectus muscle) forcing the eye to move left, and loosen muscle at the inner corner of the left eye (medial rectus muscle) so that the eye isn’t moved towards the right. In summary of the Vestibulo-Ocular Reflex If we move head to one side; Excitation of Contralateral abducens nucleus → Lateral rectus contraction in the Contralateral side to the movement Excitation of Ipsilateral oculomotor nucleus → Medial rectus contraction in Ipsilateral side to the movement Inhibition of Ipsilateral abducens nucleus → Lateral rectus relaxation in Ipsilateral side to the movement Inhibition of Contralateral oculomotor nucleus → Medial rectus relaxation in Contralateral side to the movement Nadia Aldaher Part 4 - Pain, Taste & Smell System Lecture 6 Sense Reception Receptor Trigger Highest Depolarization Specialized cells / type Cell center Mechanism Membranes Pain Primary Free nerve Mechanical Postcentral Na+ influx via Nerve fibers: (Nociceptive) endings pressure Gyrus mechanosensitive channels A-delta fibers: For Receptor in Produces Temperature primary pain nerve Action 1. Myelinated Potential 2. Fast 3. Small receptive fields 4. Dull pain 5. Good localization C fibers: For secondary pain 1. Unmyelinate d 2. Slow 3. Large receptive fields 4. Sharp pain 5. Poor localization Pain Primary Free nerve Mechanical Postcentral Na+ influx via Nerve fibers: (Antinociceptiv endings pressure Gyrus mechanosensitive e) channels C fibers: Receptor in Produces Peripheral nerve Action pathway Potential A-beta fibers Touch-pressure pathway Taste Secondary Modified Chemical Insula Salty: Salty: epithelial cells trigger Triggered by Na+ (Cerebral cortex) - Na influx Produces Ca+ influx due to voltage Sour: gated channels after Triggered by H+ Nadia Aldaher Receptor Depolarizatio depolarization → seperate from n Neurotransmitter release Bitter: nerve Triggered by K+, Sour: Mg+, Alkaloids and Long-chain nitrogen - H+ influx containing substances - K+ outflux inhibition Sweet: Triggered by Sugars, Ca+ influx due to alcohols, amino acods voltage gated channels after depolarization → Umami: Neurotransmitter Triggered by release L-glutamate Bitter, Sweet & Umami: - G-protein stimulation → Phospholipase C activation → PIP2 → IP3 + DAG Ca+ influx from sarcoplasmic reticulum due to 2nd messenger generation → Neurotransmitter release Smell Primary Modified Chemical Uncus G-protein stimulation nerve cells trigger Adenylyl cyclase → in Olfactory cAMP generation epithelium - Ca2+ influx Receptor in Produces - Na+ influx Action nerve - Cl- outflux Potential Nadia Aldaher Pain System (Primary reception) Two Categories: 1. Nociceptive Sensory System (causes pain) 2. Antinociceptive Sensory System (inhibits pain) 1. Nociceptive Sensory System Causes Thermal Stimuli > 45°C and < 5 °C Chemical Stimuli Acids and other substances Mechanical Stimuli Pressure, stretch or damage Hyperalgesia and Allodynia Hyperalgesia Increased pain sensation on a normally Painful stimuli Pain stimuli on damaged or irritated tissue that would lead to an increase pain sensation Example: Applying a painful stimuli on a bruise on your thigh will increase your level of pain sensation compared to applying a painful stimuli on a non-bruished part of your thigh. Important to note: You are comparing painful stimuli on same area, only difference is one produces more pain than the other, but both should produce pain. Hyperalgesia is not triggered by non-painful stimuli as to not mix it up with Allodyna. Allodyina Pain sensation on a normally NON-painful stimuli Non-painful stimuli on body that leads to a sensation of pain Example: Turning your head to the side should NOT produce any pain, but due to a decreased pain threshold, stimuli that would normally not cause pain now causes pain Hyperalgesia and Allodyna can be present at the same time, for example with a sunburnt area. Allodyna makes the area that is sunburnt experience pain from when light touches the area, and Hyperalgesia will increase your pain sensation when applying brute force on the area, compared to doing it on a non-sunburnt part of your skin. Nadia Aldaher Peripheral Sensitization vs Central Sensitization Peripheral Sensitization Release of inflammatory substances in Peripheral Tissues Opens Na+ channels in nerve ending making it more sensitive (Hyperalgesia) “Pain” substances are: Histamine Bradykinin Interleukins ATP Adenosine Serotonin Endothelin Growth Factors Both Primary and secondary Hyperalgesia occurs here Primary Hyperalgesia: All but Histamine release causes Primary Hyperalgesia Proliferation of keratinocytes → Activation of immune system cells → Vasodilation in skin → Edema formation Secondary Hyperalgesia: Only Histamine release causes Secondary Hyperalgesia Pain receptor sensitization and inflammatory reactions around the irritated region Central Sensitization Reorganization of impulse transmission in the central nervous system Normally: Touch stimuli causes sensitization through touch pathway and Pain stimuli causes sensitization through pain pathway (both separate) But in central sensitization: Allodynia: Reorganization of pain synapses also affects touch pathway (touch stimuli can lead to painful sensation) Hyperalgesia: High impulse pain frequency due to increased neurotransmitters and receptors on pain pathway Pain due to Mechanism of Generation Acute Pain (Nociceptive) Chronic Pain Nadia Aldaher Physiological pain in previously intact tissues. Pain that has persisted for longer than 3 months Caused by sudden damage of tissues. Short-lasting. Two categories: 1. Inflammatory Chronic pain Pain following tissue injury with no neural injury, has both peripheral and central sensitization Treated by: Cyclooxygenase 2 inhibitors (inhibition of prostaglandin production) Opioids (suppress pain impulse transmission) 2. Neuropathic Chronic pain Pain that occurs after neural injury also accompanied by peripheral and central reorganization Treated by: Trycilic antidepressant Anticonvulsants (block calcium influx into presynaptic terminals) Na+ channel blockers (block sodium