[PHY LEC - LE 1] 04 - Nerve (V3).pdf
Document Details
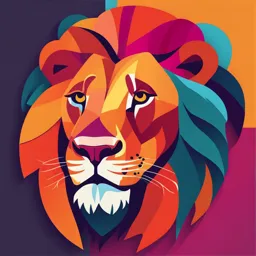
Uploaded by IntegralPlanet
Tags
Full Transcript
PHYSIOLOGY LECTURE | TRANS #4 LE Nerve CAROLINA C. JEREZ, MD | 08/ 14/2023...
PHYSIOLOGY LECTURE | TRANS #4 LE Nerve CAROLINA C. JEREZ, MD | 08/ 14/2023 01 OUTLINE B. PERIPHERAL VS CENTRAL I. Introduction III. Membrane potential Peripheral Nervous System (PNS) A. Nervous system A. Resting membrane → consists: cranial nerves and spinal nerves B. Peripheral vs Central potential → function: provides an interface between the environment and nervous system B. Resting membrane the CNS C. Peripheral Nervous potential equations Central Nervous System (CNS) System Division C. Factors affecting → consist: brain and spinal cord D. Autonomic Nervous RMP → function: processing center of the nervous system System Division D. Factors that bring II. Nerves back RMP C. PERIPHERAL NERVOUS SYSTEM DIVISION A. Neuron E. Ion channels Autonomic B. Neuron classification: IV. Action potential → involuntary nervous system processes A. Ionic basis of action → responsible for homeostasis C. Neuron classification: potential Somatic function B. Features of action → associated with voluntary control of the body D. Neuroglial cells potential Visceral E. Mechanism of injury for C. Refractory period → relay information between the CNS and visceral organs Peripheral nerves V. Conduction velocities VI. Review Questions D. AUTONOMIC NERVOUS SYSTEM DIVISION VII. References Sympathetic → ‘fight or flight’ responses ❗️ Must know 💬 Lecturer 📖 Book 📋 Previous Trans Parasympathetic → ‘rest and digest’ SUMMARY OF ABBREVIATIONS Enteric AP Action Potential → ANS in the gastrointestinal tract PNS Peripheral Nervous System II. NERVES CNS Central Nervous System ANS NT Autonomic Nervous System Neurotransmitter A. NEURON Parts of a Neuron 📋 SC Spinal Cord RMP Resting Membrane Potential LEARNING OBJECTIVES ✔ Know the division of the nervous system ✔ Describe the cells found in the nervous system and differentiate according to function ✔ Explain the resting membrane potential ✔ Differentiate local potentials; action potentials; synaptic potentials ✔ Differentiate the types of synapses Figure 2. Neuron structure [byjus.com] ✔ Differentiate neurotransmission and neuromodulation Cell body (soma) ✔ Enumerate the different neurotransmitters → where nucleus is located I. INTRODUCTION → cell’s life support center A. NERVOUS SYSTEM Dendrites Nervous system → Receive messages coming from the periphery → short processes that carry impulses toward the cell body → neuron may have several dendrites Axons → Long processes that carry impulses away from the cell body towards other neuron, muscles or glands Terminal button → refers to synaptic knobs at the end of the presynaptic terminals Terminal branches → Forms junction with other cells; different from terminal buttons Myelin Sheath → covers the axon of some neurons and helps speed neural impulses Figure 1. Overview of nervous system [Lecture PPT] Myelinated Presence of Myelin Sheath 📋 → Transmitting cells are called neurons which communicate with 📋 📋 each other using electrical potential called action potential → With myelin sheath on the surface of the cell (AP) → Acts as an insulator around nerve axons → Functions: → Faster conduction compared with unmyelinated (Saltatory - detect changes & feel sensation Conduction) - initiate appropriate responses to change → Some cells have Schwann cells that are able to produce myelin - organize information for immediate use and store it for sheath future use Unmyelinated LE 1 TG 6 | Forones, Frace, Framil, Fuedan, Galang, TE | Forones, Framil, Galang AVPAA | J. Enriquez, T. PAGE 1 of 6 TRANS 1 Galicia Guzman PHYSIOLOGY | LE1 Nerve | Carolina C. Jerez, MD → Without myelin sheath on the surface of the cell D. NEUROGLIAL CELLS B. NEURON CLASSIFICATION:PROCESSES Figure 5. Neuroglial cell types [Lecture PPT] aka glial cells non neuronal function ○ provide homeostatic support, protection, etc. Table 1. CNS vs PNS neuroglial cells Figure 3: Neuron Types according to processes[Lecture PPT] CNS neuroglial cells Description Unipolar → Single process with different segments acting as Astrocytes maintain blood brain barrier receptive surface and releasing terminals control the amount of NT around Bipolar synapses → Two specialized process: (1) axon - carry impulses away from regulate ions the cell body, and (2) dendrites - carry impulses toward the cell provide metabolic support body Pseudounipolar Ependymal line the SC and ventricles of the → Single process which splits into two, both of which function as brain axons—one going to skin or muscle and another to the spinal Cerebrospinal Fluid (CSF) cord production Multipolar → singular axon, multiple dendrites Anaxonic Oligodendrocytes myelination in the CNS → Lack true axons and do not produce action potentials, but structural framework regulate local electrical charge of adjacent neurons Microglia brain’s ‘immune system’ C. NEURON CLASSIFICATION: FUNCTION removes dead cells and pathogens (phagocytosis) PNS neuroglial cells Description Satellite surround neuron cell bodies in the ganglia regulate NT levels Schwann myelination in the PNS regeneration of neurons post injury E. MECHANISMS OF INJURY FOR PERIPHERAL NERVES Figure 4: Neuron Types according to functions[Lecture PPT] Sensory Neurons → Afferent Neurons → Send impulses from periphery to center for processing Motor Neurons → Efferent neurons → Carry communication from the center towards periphery → Carry responses after the information has been processed by the CNS Interneurons → Multipolar cells area 📋 → Stays solely in the CNS and communicates only to cells in that Figure 6. Mechanisms of Injury for Peripheral Nerves[Lecture PPT] PHYSIOLOGY Nerve PAGE 2 of 6 PHYSIOLOGY | LE1 Nerve | Carolina C. Jerez, MD Table 2. Seddon’s nerve Injury classification[Lecture PPT] Where: Equation 1. Nernst equation❗️ Em = Nernts equilibrium potential R = Gas constant T = temperature in degrees Kelvin Ln = natural logarithm [A-]o = conc. of ion outside [A-]i = conc. of ion inside z = number of electron/valence electron F= Faraday’s constant This can be converted into a base ten log with the total of the constants being 58 (derived from a normal human physiological environment), divided by the number of valence electrons. III. MEMBRANE POTENTIAL Membrane Potential (MP) refers to the separation of opposite charges across the membrane or to the relative difference of the 📋 number of cations and anions in the intracellular fluid environment (ICF) and extracellular fluid environment Nerves, being polarized electrically, generate rapidly changing ❗️ 📖 electrochemical impulses to transmit signals along the nerve or Equation 2. Simplified Nernst equation muscle membranes. APPLYING THE NERNST EQUATION For Potassium (K+): 📋 A. RESTING MEMBRANE POTENTIAL (RMP) → The valence for K+ is 1, thus, the eqn. is as follows: The potential of the neuron when not active or at rest ▪ E = 58 X log [5 / 140] membrane in millivolts (mV) 📖 Expressed as the measured potential difference across the Established by diffusion potentials from concentration differences ▪ E = -84 mV For Chloride (Cl-): → The valence of Cl- is -1 of permeant ions (Na+, K+ and Cl-). ▪ E = 58 X log [110 / 10] → Inside the cell: More K+ and negatively charged proteins ▪ E = -60 mV (Results in more negative ICF than ECF) 📋 → Outside the cell: More Na+ and Cl-. 📋 RMP of Neuron: -70Mv Also known as Goldman Equation 📋 GOLDMAN-HODGKIN-KATZ (GHK) EQUATION 📖 → When depolarized, RMP will change because of the movement Used when the membrane has more than one ion producing of the ions: Na+, K+ and Cl- membrane potential difference. → Result of the high resting conductance to K+ that drives the membrane potential toward the K+ equilibrium potential. 📖 📖 Solves for the diffusion potential when the membrane is RMP is negative inside the cell relative to the outside because: → The resting membrane is 10-100 times more permeable to K+ permeable to several different ions. The diffusion potential that develops depends on three factors: → the polarity of the electric charge of each ion 📖 than to Na+ → the permeability of the membrane (P) to each ion charged ions with it. 💬 → Slow leakage of K+ out of the cell, carrying the positively → The concentration of the ions The equation is as follows: 💬 → Cl- can’t be carried outside by K+ because of the chloride’s high extracellular concentration. ions) can’t leave the cell. 💬 → The non-diffusible anion (protein, sulfate and phosphate Equation 3. Goldmann-Hodgkin-Katz (GHK) equation ❗️ To Remember Movement of Ions: PISO: Potassium In, Sodium Out 📋 Where: Vm = Membrane potential P = Permeability constant of ion R = Gas constant T = temperature B. RESTING MEMBRANE POTENTIAL EQUATIONS F = Faraday’s constant Ln = natural logarithm Goldman-Hodgkin-Katz (GHK) Equation and Nernst Equation helps in determining the magnitude of the membrane potential. 💬 K+ = Potassium [ ]o = conc. of ion outside Na+ = Sodium Cl- = Chloride [ ]i = conc. of ion inside each ion individually. 📋 Nernst equation helps in solving for the equilibrium potential for The magnitude of membrane potential at any given time depends ❗️ Chloride is reversed in the eqn. since it is a negative ion, while Sodium and Potassium are positive ions. the GHK equation is used. 💬 on the distribution and permeability of Na, K. and Cl ions. For this, MEMBRANE POTENTIAL 💬 GHK Equation solves for the indicated roles in the generation of membrane potential. the fluid mosaic model of the membrane 📋 The membrane of a neuron is made up of a phospholipid bilayer of → Outer & inner edges: phosphate heads (hydrophilic) NERNST EQUATION → Inside: lipid tails (hydrophobic) conditions. 📋 Allows the determination of the cell potential under nonstandard 📋 There is a potential difference across the membrane of the neuron → Inside of the cell is negative compared to the outside of the cell 📋 Predicts the membrane potential for a single ion. → The cell will send signal to other neurons in the brain/spinal Used to calculate the equilibrium potential at a given cord when this potential is changed by incoming stimuli 📖 concentration difference of a permeable ion across a cell membrane. equilibrium. 📖 Tells us at what potential the ion would be at electrochemical Varies with body temperature. 📋 📋 The Nernst Equation. is determined by starting with the Gibbs free energy as follows: PHYSIOLOGY Nerve PAGE 3 of 6 PHYSIOLOGY | LE1 Nerve | Carolina C. Jerez, MD Chloride → Inside the cell, chloride level is 10 mM → Outside the cell chloride level is 110 mM → Membrane is not permeable to chloride → When the voltage-dependent chloride channels open, chloride wants to move into the cell down its chemical gradient → It doesn’t go far because the electrical gradient pulls the ion outward → When chloride enters the cell it makes the inside even more negative, hyperpolarizing the cell Figure 7. Membrane of a neuron [Video in Lecture] SODIUM Inside of the cell has a sodium level of 10 mM Outside of the cell is 145 mM The membrane is not permeable to sodium so it does not allow sodium to cross ▪ Sodium can only cross the membrane when voltage-gated channels are opened Figure 10. Movement of Chloride and Calcium in membrane [Video in ▪ Both sodium electrical gradient and concentration gradient Lecture] are directed inward ▪ When the voltage-gated sodium channels open, sodium EXAMPLES moves into the cell then explosively depolarizing the cell or Example using K making membrane potential less negative E = 58 x log [5 / 140] → Inside of the cell has many proteins which have a negative E = -84 mV charge which makes the inside of the cell negatively charged Example using Cl overall E = -58 x log [110 / 10] E = -60 mV IONIC OHM’S LAW/ CHORD CONDUCTANCE EQUATION Estimates the MP knowing equilibrium potentials and conductance of each ionic species in question A. FACTORS CONTRIBUTING TO RMP Concentration gradient 📋 → Usually, ion will flow from greater concentration to lower concentration Electrical gradient → Like signs; repel Figure 8. Movement of Na+ in membrane [Video in Lecture] → Opposite signs; attract Permeability of the cell membrane POTASSIUM Active transport Inside the cell, the potassium level is 140 mM → Transport ions against their concentration gradient Outside the cell, potassium level is 5 mM → Most important example is is Na+ & K+ pump which needs In the neuron, membrane is permeable to potassium so it can energy in the form of ATP pass through cell membrane easily ▪ Transport of Na+ to outside and K+ to inside → Potassium dilemma ▪ Chemical gradient for potassium is to move out of the cell NOTE while electrical gradient is to stay inside the cell These forces are responsible for: ▪ Potassium does move out of the cell but it doesn’t go far, → Maintenance of resting membrane potential sitting just outside the membrane and returning it to its → Development of action potential resting potential → Bringing the cell back to its resting state after the action potential is over B. TYPES OF CHANNELS IN THE CELL Ligand-gated channels → open in response to ligand binding Mechanically-gated channels → Open or close due to mechanical stimulus → Example: sensory receptors that respond to pressure or stretch Volted-gated channels Figure 9. Movement of K+ in membrane [Video in Lecture] → open in response to change in the voltage gradient CHLORIDE AND CALCIUM → allow passage of specific ions along their concentration Calcium Leak channels → Inside the cell, the calcium level is 0.0001 mM → Open or close randomly → Outside the cell, calcium level is 2 mM → Channels which are open all the time usually allow potassium → Membrane is not permeable to calcium to pass through (ex. K+ leak channels cause nerve and muscle → When the voltage-gated calcium channels open, the calcium membranes to be more permeable to K+ than Na+) wants to move into the cell down both its chemical and electrical gradient; This influx of calcium will depolarize the membrane locally PHYSIOLOGY Nerve PAGE 4 of 6 PHYSIOLOGY | LE1 Nerve | Carolina C. Jerez, MD → Due to the activity of the sodium-potassium ATPase pump, as well as the slow closure of the potassium channels Go back to resting membrane potential [Lecture PPT] Figure 11. Ion channels Figure 13. Action Potential [Lecture PPT] B. FEATURES OF THE ACTION POTENTIAL Propagated potential → From the point of stimulation up to the terminal of the neuron → To produce an action potential, a propagated potential, you need a threshold stimulus - minimum amount of stimulus Figure 12. Voltage-gated channels [Lecture PPT] All or none 💬 strength to elicit a response → AP happens or it just doesn’t Decrementless C. FACTORS THAT BRING BACK THE RMP → No decrease in the amplitude of the action potential from the Active sodium/potassium ATPase pump start of stimulation up to the terminal of the neuron. → (3) NA+ out and (2) K+ in Has a Period of Refractoriness Slow closure of the voltage-gated potassium channels → Period of rest for neurons C. REFRACTORY PERIOD IV. ACTION POTENTIAL Absolute Refractory Period Propagating → Period where no amount of stimulus strength will be able to re → Rapid changes in MP that spread rapidly along the nerve fiber excite your neuron membrane → Ion channels have not yet recovered from its previous activity A property of excitable cells that consist of: Relative Refractory Period → Rapid depolarization/upstroke → A stronger than threshold stimulus may be able to restimulate → Repolarization of the MP neuron and may have another action potential Has stereotypical shape and size ▪ Some sodium channels have recovered from its previous Exhibits “all-or none” response activity → The stimulus either produces a full-sized action potential or fails to produce one It is decrementless → It will not change its amplitude from the start up to the terminal end of the neuron A. IONIC BASIS OF ACTION POTENTIAL Resting Membrane Potential: → Upon stimulation, which is the rapid change in membrane potential (MP) across the nerve fibre membrane → In this case, we have -65 millivolts Depolarization → Once stimulated and it reaches the threshold, there will be an opening of the sodium gates, → Thus sodium will rush into the cell Overshoot → The sodium channels will start to close and the potassium gates will open Repolarization → The potassium will get out of the cell, thus an increase of Figure 14. Refractory Period [Lecture PPT] potassium efflux Physiological Importance of Refractory Periods: renders → Hence the downward slope on the AP neurons indefatigable. Hyperpolarization → A state where it is lower than the resting membrane potential PHYSIOLOGY Nerve PAGE 5 of 6 PHYSIOLOGY | LE1 Nerve | Carolina C. Jerez, MD V. CONDUCTION VELOCITIES 2. False. Neuroglial cells have no neuronal function. They function as supporting cells Nerve conduction velocity studies measure how fast an 3. Absolute - no action potential can occur when with strong stimulus. Relative electrical impulse travels through the length of the nerve. - follows an absolute refractory period, action potential can be generated (From point of stimulation) with a large enough stimulus Use in diagnosing nerve disorders (ex. Carpal Tunnel Syndrome 4. B. The velocity of an action potential increases in proportion to the diameter or Guillain Barre’). of the axon for both myelinated and unmyelinated axons. Myelination It is requested when symptoms like a tingling sensation (in the increases the velocity of an action potential by several orders of magnitude fingers, hands, or legs), numbness in the extremities (or some more compared with the effect of an increase in axon diameter, which means that a large myelinated axon has the highest velocity of conduction. 📋 📋 changes in sensation in the body). It is also known as Nerve Conduction Velocity (NCV) The nerve conduction velocity is determined by two main Therefore, even though unmyelinated axon E has the greatest diameter, myelinated axon B can conduct an action potential at a much greater velocity factors: 5. D. The resting potential of any cell is dependent on the concentration ➔ Diameter of the nerve/size gradients of the permeant ions and their relative permeabilities (Goldman - larger diameter = faster conduction velocity equation). In the myelinated nerve fiber, as in most cells, the resting ➔ Degree of Myelination membrane is predominantly permeable to K+. The negative membrane potential observed in most cells (including nerve cells) is due primarily to the - faster in myelinated neurons relatively high intracellular concentration and high permeability of K+ Table 3. Conduction velocities study[Lecture PPT] VII. REFERENCES 2026B Trans PDF/Nerve and Synapse – Carolina Jerez, MD (Asynch) Hall, J. (2015). Guyton and Hall Textbook of Medical Physiology (13th edition). Elsevier. Koeppen, B., & Stanton, B. (2018). Berne & Levy physiology. Philadelphia, PA: Elsevier. ➔ Conduction velocities studies for individuals from a segment of the nerve (from axilla to the wrist) VI. REVIEW QUESTIONS 1. Which of the following is true for the Peripheral Nervous System? a. Consists of the brain and spinal cord b. It is processing center of the nervous system c. Consists of cranial nerves and spinal nerves 2. True or False. Neuroglial cells have neuronal function. 3. What is the difference between absolute refractory period and relative refractory period? 4. Five hypothetical nerve axons are shown in the above figure. Axons A and B are myelinated, whereas axons C, D, and E are non-myelinated. Which axon is most likely to have the fastest conduction velocity for an action potential? 5.The resting potential of a myelinated nerve fiber is primarily dependent on the concentration gradient of which of the following ions? a. Ca++ b. Cl− c. HCO3 − d. K+ e. Na+ ANS: 1. C PHYSIOLOGY Nerve PAGE 6 of 6